Mandarin fish (Siniperca chuatsi) is highly favoured among the Chinese, just as described in the ‘A Fisherman’s Song’ authored by the poet, Zhihe Zhang, in Tang Dynasty. In 2023, the production of mandarin fish was above 470 000 tonnes(1). However, the mandarin fish largely rely on live bait, and in practice, about less than 10 % of the total production of compound feed has been applied in the cultivation of this fish species(Reference Xiong and Lin2), which is mainly due to the lack of studies on its nutrient requirement. Hence, studies relating to the nutritional physiological characteristics of this fish species could produce significant benefits to its cultivation sector.
Carbohydrates and lipids act as the main non-protein energy sources of aquafeed, and their potential protein-sparing effects have been well documented in teleosts(Reference Kamalam, Medale and Panserat3,Reference Turchini, Francis, Du, Halver and Hardy4) . Carbohydrates are the cheapest energy source and act as a binder in aquafeed. However, considerable studies have proved that dietary carbohydrates content for most warm water carnivorous fish should be less than 100 g/kg, and excessive dietary carbohydrates could lead to metabolic disorders, which further induce the reduction in growth and feed utilisation, suppression of immune function and increased pathogens susceptibility to cultured fish, such as the study on largemouth bass (Micropterus salmoides)(Reference Gou, Chen and Xu5), hybrid grouper (Epinephelus fuscoguttatus ♀ × E. lanceolatus ♂)(Reference Li, Li and Zhang6) and red-spotted grouper (Epinephelus akaara)(Reference Wang, Li and Han7). Lipid is another essential energy source, which is also an important source of essential fatty acids and function as the carriers of fat-soluble vitamins(Reference Turchini, Francis, Du, Halver and Hardy4). However, excessive dietary lipid also produces negative impacts on farmed fish, such as abnormal lipid deposition, oxidative stress and inflammation response, as reported in large yellow croaker (Larimichthys crocea)(Reference Yan, Liao and Wang8), Japanese seabass (Lateolabrax japonicus)(Reference Xie, Lin and Wu9) and turbot (Scophthalmus maximus L.)(Reference Ma, Bi and Kong10). Therefore, suitable dietary carbohydrates and lipids content for carnivorous fish should be determined, while it cannot be truly defined due to the interaction between dietary lipids and carbohydrates as described in some fish species, such as gilthead sea bream (Sparus aurata)(Reference Castro, Corraze and Firmino-Diógenes11), hybrid grouper(Reference Li, Li and Chen12) and large yellow croaker(Reference Li, Yin and Zhang13). However, to date, little information is available on the interaction between dietary carbohydrates and lipids in mandarin fish.
In recent years, with the improvement in living standards, the flesh quality of farmed fish has attracted more attention. In practice, some consumers believe that the flesh quality of farmed mandarin fish fed compound feed does not match those fed forage fish. It has been well documented that dietary compositions affect the flesh quality, including nutritional and sensory quality of farmed fish(Reference Lie14). The texture and structure of fish muscle are important fresh quality attributes, which include hardness, springiness, chewiness, cohesiveness, resilience and internal cross-linking of connective tissue(Reference Cheng, Sun and Han15). Growing evidences have confirmed that dietary lipid content is a basis for the forming of flesh texture, and muscle lipid deposition commonly softens fish flesh(Reference Johnston, Li and Vieira16–Reference Wang, Qiao and Zhang18). Meanwhile, the variation in dietary carbohydrate content leads to glycogen deposition and influences the flesh texture as reported in farmed dentex (Dentex dentex)(Reference Suárez, Martínez and Abellán19)and olive flounder (Paralichthys olivaceus)(Reference Liu, Deng and Pan20). Therefore, it’s suggested that the dietary carbohydrate and lipid levels and their interactions might also affect the flesh quality of mandarin fish.
Considering all of the above, a two-factor (3 × 3) orthogonal study was designed to investigate the effects of dietary carbohydrate and lipid levels on growth performance, hepatic histology and antioxidant capacity and flesh quality of mandarin fish. It is envisaged that the present study would be helpful to enrich the current knowledge of this fish species and further promotes the development of this aquaculture sector.
Materials and methods
Experimental diets
Nine isonitrogenous (crude protein 520 g/kg) experimental diets containing graded levels of carbohydrates (40 g/kg, 80 g/kg and 120 g/kg) and crude lipids (80 g/kg, 120 g/kg and 160 g/kg) were formulated in a 3 × 3 factorial design (Table 1). The feed ingredients were mixed thoroughly and then produced a stiff dough following the description in Li et al.(Reference Li, Li and Zhang6) Then, the dough was extruded with a pelleting machine through the 2·5 mm, 3·5 mm and 6·0 mm die, respectively, to form different particle sizes with the length of 1·3 cm, 1·7 cm and 2·5 cm, respectively. After that, the pellets were cooked for starch gelatinisation (105°C, 15 min) and dried (55°C, 6 h) in a ventilated oven. Then, the diets were stored at –20°C until the experiment was started.
Table 1. Formulation and chemical composition of experimental diets (g/kg DM)
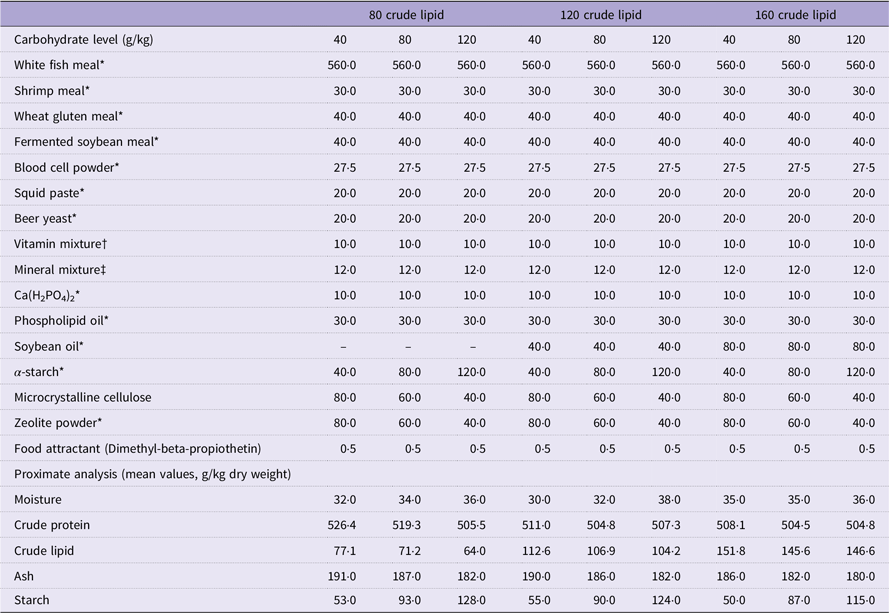
* Supplied by Xinxin Tian’en Aquatic Feed Co. Ltd.
† Vitamin Premix (mg/kg diet): vitamin A (Retinol), 4.8; vitamin D3, 0.2; vitamin K3, 14·72; vitamin B1, 17·80; vitamin B2, 48; vitamin B6, 29·52; vitamin B12, 0·24; vitamin E, 160; vitamin C, 800; niacinamide, 79·20; calcium-pantothenate, 73·60; folic acid, 6·40; biotin, 0·64; inositol, 320; choline chloride, 1500; l-carnitine, 100.
‡ Mineral Premix (mg/kg diet): Cu (CuSO4), 2·00; Zn (ZnSO4), 34·4; Mn (MnSO4), 6·20; Fe (FeSO4), 21·10; I (Ca (IO3)2), 1·63; Se (Na2SeO3), 0·18; Co (CoCl2), 0·24; Mg (MgSO4·H2O), 52·7.
Experiment procedure
The feeding trial was conducted in the joint laboratory of Shanghai Ocean University and Guangdong Evergreen Feed Industry Co. Ltd (Zhanjiang, China). The juvenile mandarin fish, average body weight of 15 g, were obtained from Guangdong Liangshi Aquatic Seed Industry Co. Ltd (Guangzhou, China), which were then domesticated using the experimental diet (120 g/kg crude lipid, 40 g/kg carbohydrate). After 4 weeks’ acclimation, a total of 945 fish with similar body weights (55 g) were randomly assigned to twenty-seven tanks (thirty-five fish/tank; water volume, 1000 litre). Triplicate tanks of fish were fed one of the experimental diets twice daily (07.00 and 16.30) for 10 weeks. During the feeding trial, the fish were gradually fed the diet with particle diameters of 2·5 mm (body weight < 50 g), 3·5 mm (body weight < 120 g) and 6·0 mm (body weight > 120 g). The feeding trial was conducted in a circulating water system, which was equipped with a thermostat and a UV lamp for disinfection. During the experiment process, a siphon was used to remove the dirt from the bottom of the bucket and discharge the sewage from the filtration tank 2 h after feeding, and then groundwater was added to the system as a supplement. The water quality of the system was monitored to be consistent throughout the entire feeding process. Water quality variables were maintained at pH 8·0 ± 0·2, temperature 28 ± 0·5°C, nitrite content less than or equal to 0·01 mg/l, ammonia nitrogen less than 0·2 mg/l and nitrate nitrogen within 25 to 50 mg/l.
Sample collection
After 10 weeks’ feeding trial, the total fish number and fish weight were recorded after the fish were fasted for 24 h, and the fish for sampling were anaesthetised with eugenol (1:10 000). Then, fifteen fish were randomly selected for further sample collection. Briefly, for each tank, five fish were randomly collected for the analysis of the composition of the whole body, and the individual body length and weight of the remaining ten fish were measured for the calculation of condition factor. Then, the liver and visceral mass of six fish were separated for hepatosomatic index and viscerosomatic index calculation, respectively. After that, liver samples were collected for histological analysis, proximate composition analysis and enzyme activity analysis, and muscle samples were separated for the analysis of proximate composition and texture.
Body proximate composition analysis
Body composition was analysed based on the method in the Association of Official Analytical Chemists (AOAC)(21). Moisture content was measured by drying samples to a constant weight (# 934.01; AOAC, 2003), ash content was determined by combustion (# 942.05; AOAC, 2003) and the Kjeldahl nitrogen determination method was used to measure crude protein content (KD310, Opsis) (# 976.05; AOAC, 2003). Soxhlet extraction method was taken to measure crude lipid content (SX-360, Opsis) (# 920.29; AOAC, 2003). The potassium hydroxide/anthrone was used to measure hepatic glycogen content(Reference Seifter, Dayton and Novic22), following a commercial kit (Nanjing Jiancheng Bioengineering Institute).
Hepatic antioxidant capacity analysis
The liver tissue samples were cleaned with precooled physiological saline at 4°C, and then the surface water of the liver sample was absorbed using absorbent paper. The cleaned liver samples were mixed with phosphate buffer and then homogenised using a tissue homogeniser (1:9, w/v, 10 000–15 000 r/min) and centrifuged (2000 rpm, 10 min, 4°C) to separate the supernatants. The total antioxidative capacity, malondialdehyde (MDA) content and the activity of superoxide dismutase (SOD) and catalase (CAT) were analysed using commercial kits following the instruction (Nanjing Jiancheng Bioengineering Institute; catalogue no.: total protein, A045–2–2; MDA, A003–1; total antioxidative capacity, A015–2–1; CAT, A007–1–1; SOD, A001–3). In detail, total antioxidant capacity was evaluated by measuring the content of Fe2+ reduced from Fe3+, which could form a solid orange–red complex with phenanthroline analogues, and its antioxidant capacity could be measured by colorimetry(Reference Benzie and Strain23). The total SOD activity was measured with the ferricytochrome C method(Reference Mccord and Fridovich24). MDA, a degradation product of lipid peroxidation, condenses with thiobarbituric acid to form a red product with a maximum absorption peak at 532 nm(Reference Kei25). The above indices were expressed as specific activity, and the soluble protein content was determined with the coomassie brilliant blue method(Reference Bradford26). CAT activity was determined by measuring the decreased concentration of H2O2 per minute according to Aebi(Reference Aebi27).
Hepatic histology analysis
After sampling, liver samples were cut into 1 cm3 and fixing with 4 % paraformaldehyde. Then the samples were dehydrated and embedded in paraffin wax. After that, the samples were sectioned at 5 μm thickness for sub sequent staining. The slides were deparaffinized and rehydrated with dimethyl benzene and graded concentrations of ethanol stained with haematoxylin and eosin in conjunction. Finally, the images were captured (40×) using the Olympus-DP73 optical microscope (Olympus) was used to capture images.
Flesh quality analysis
The texture analysis of the dorsal muscle was performed with texture profile analysis using a Universal TA device (TA. ZM-Plus, Dexin Technology (Kunming) Co. Ltd). Test conditions were set as previously described by Xu et al.(Reference Xu, Yang and Zhang28)
Statistical methods
All data were analysed using SPSS version 22. The interaction effects of dietary lipid and carbohydrate levels on cultured fish were analysed by factorial (two-way) ANOVA. If the interaction was not significant, the Tukey’s multiple range test was followed to determine the effects of a single main effect (lipid or carbohydrate). If the interaction was significant, one-way ANOVA followed by Tukey’s multiple range tests were used to determine the effects of one factor under different levels of another factor. The significance level was set at P < 0·05.
Result
Growth performance and feed utilisation
Different dietary lipid and carbohydrate levels did not significantly affect the survival rate, final body weight, specific growth rate, feed intake, feed efficiency rate and protein efficiency rate of mandarin fish (P > 0·05) (Table 2). The fish fed a dietary lipid (160 g/kg) and carbohydrate level (120 g/kg) displayed significantly higher condition factor and viscerosomatic index compared with those fed a low dietary lipid level (80 g/kg) and a low carbohydrate level (40 g/kg), respectively, (P < 0·05) (Table 2). The hepatosomatic index was only affected by dietary carbohydrate level and significantly increased with increasing carbohydrate levels (P < 0·05) (Table 2).
Table 2. Growth performance and feed utilisation of mandarin fish fed diets with varying lipid and carbohydrate levels for 10 weeks

IBW, initial body weight; FBW, final body weight; SR, survival rate; FI, feed intake; SGR, specific growth rate; FER, feed efficiency ratio; PER, protein efficiency ratio; CF, condition factor; VSI, viscerosomatic index; HSI, hepatosomatic index.
* Treatment means represent the average values of three tanks per treatment.
† Differences were regarded as significant when P < 0·05. L and C showed the main effect of each factor, and L × C indicated their interactive effect. Values (means, N = 3) with a different superscript letter are significantly different from the other dietary groups (p < 0.05).
Survival rate (SR, %) = final fish number/initial fish number × 100.
Feed intake (FI, g/fish/d) = dry feed consumption/[(final fish number + initial fish number)/2] /experimental days.
Specific growth rate (SGR, %/d) = ln (final body weight/initial body weight)/experimental days × 100.
Feed efficiency ratio (FER) = increased body weight/dry feed consumption.
Protein efficiency ratio (PER) = (final body weight − initial body weight)/protein intake.
Condition factor (CF, g/cm3) = final body weight (g)/length (cm)3 × 100.
Viscerosomatic index (VSI, %) = viscera weight/final body weight × 100.
Hepatosomatic index (HSI, %) = liver weight/final body weight × 100.
Approximate body composition
The whole body and liver moisture content of mandarin fish decreased significantly with increasing dietary lipid levels (P < 0·05) (Table 3). No significant effects were observed on whole-body moisture content with increasing carbohydrate levels (P > 0·05) (Table 3). However, fish fed a carbohydrate level of 40 g/kg displayed significantly higher liver moisture content than those fed 80 g/kg and 120 g/kg carbohydrate (P < 0·05) (Table 3). High dietary lipid and carbohydrate levels significantly decreased the protein content of the whole body and muscle of cultured fish (P < 0·05) (Table 3); however, only the dietary carbohydrate level affected liver protein content (P < 0·05) (Table 3). Additionally, significant interaction was observed between these two main factors in muscle protein content (P < 0·05) (Table 3). The lipid content of the whole body, liver and muscle all significantly increased with increasing levels of lipid in the feed (P < 0·05) (Table 3), while only liver lipid level was significantly increased as the increase of dietary carbohydrate level (P < 0·05) (Table 3). Hepatic glycogen content increased significantly with increasing dietary carbohydrate levels (P < 0·05) (Table 3). However, among different dietary lipid level groups, hepatic glycogen content reached the highest value in the fish feeding 120 g/kg dietary lipid (P < 0·05) (Table 3), and significant interaction between the main factors in hepatic glycogen content was observed (P < 0·05) (Table 3).
Table 3. Body composition of mandarin fish fed diets with varying lipid and carbohydrate levels for 10 weeks (g/100 g wet weight)
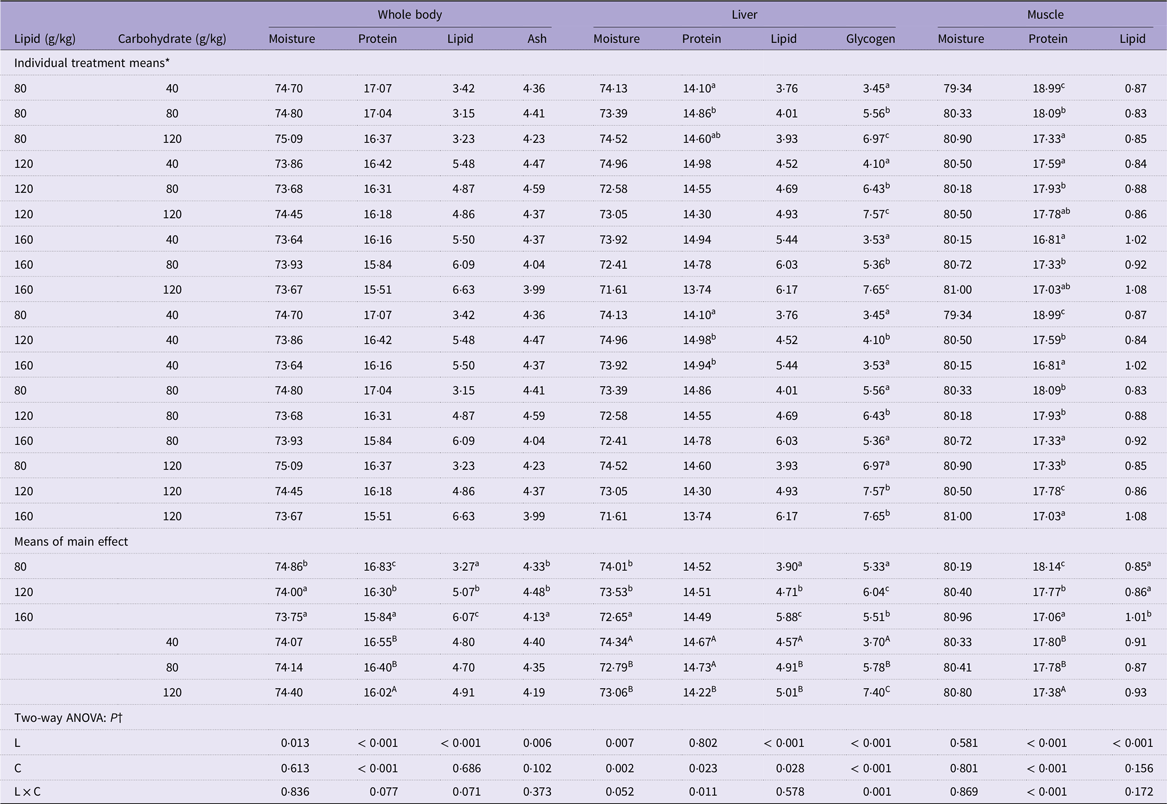
* Treatment means represent the average values of three tanks per treatment.
† Differences were regarded as significant when P < 0·05. L and C showed the main effect of each factor, and L × C indicated their interactive effect. Values (means, N = 3) with a different superscript letter are significantly different from the other dietary groups (p < 0.05).
Liver antioxidant capacity
Significant interactions of two main factors in liver MDA content and SOD and CAT activities of cultured fish were observed (P < 0·05) (Table 4). In detail, MDA content was significantly different among the dietary treatments, with its highest content measured in fish fed 160 g/kg lipid and 120 g/kg carbohydrate levels (P < 0·05) (Table 4). Liver CAT activity decreased significantly with increasing dietary lipid or carbohydrate levels (P < 0·05) (Table 4). Hepatic SOD activity increased significantly with increasing levels of lipid content in the feed (P < 0·05) (Table 4); however, it decreased first and then increased with the increasing level of dietary carbohydrate (P < 0·05) (Table 4).
Table 4. Liver antioxidant capacity of mandarin fish fed diets with varying lipid and carbohydrate levels for 10 weeks
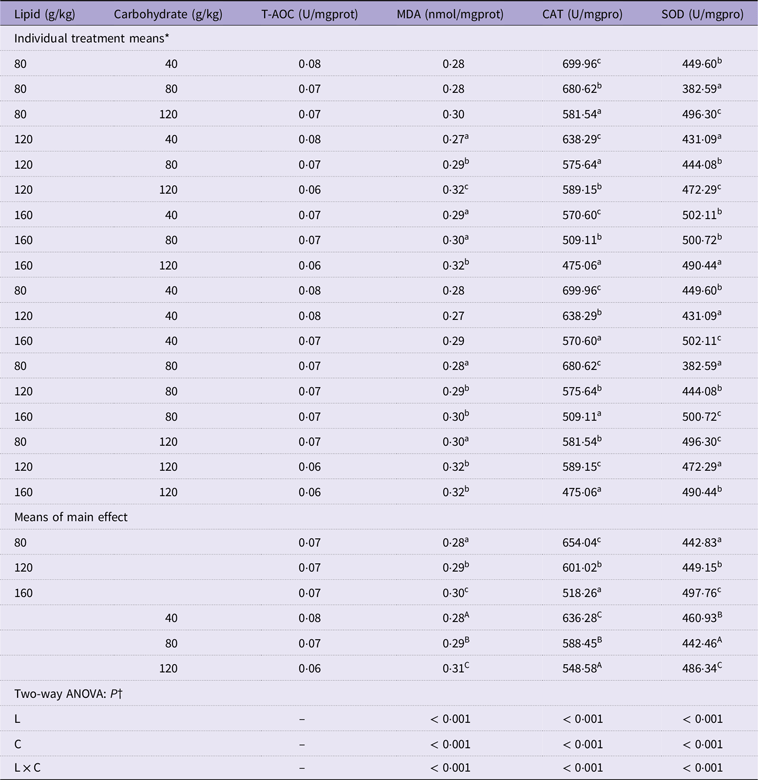
T-AOC, total antioxidative capacity; MDA, malondialdehyde; CAT, catalase; SOD, superoxide dismutase.
* Treatment means represent the average values of three tanks per treatment.
† Differences were regarded as significant when P < 0·05. L and C showed the main effect of each factor, and L × C indicated their interactive effect. Values (means, N = 3) with a different superscript letter are significantly different from the other dietary groups (p < 0.05).
Liver morphology
As shown in Fig. 1, when the dietary crude lipid levels were at 80 g/kg and 120 g/kg, and the dietary carbohydrate levels were at 40 g/kg and 80 g/kg, the result showed that the hepatocytes of mandarin fish were uniformly arranged and regular, and the cell morphology was relatively normal, with occasional small-volume lipid droplets appearing (Fig. 1(a), (b), (d) and (e)). When the dietary carbohydrate level reached 120 g/kg, the hepatocytes’ size began to become larger, with blurred edges, and the cells appeared to be swollen and vacuolated, and the nuclei of the cells were shifted (Fig. 1(c), (f) and (i)). At a high lipid level (160 g/kg), compared with a medium lipid level (120 g/kg) and low lipid level (80 g/kg), hepatocytes were surrounded by a large number of oval lipid droplets, with irregular cell morphology and obvious vacuolation (Fig. 1(g), (h) and (i)). At a high carbohydrate level (120 g/kg), the interaction effect produced by the increase in the crude lipid level exacerbated the swelling and vacuolisation of the cells, resulting in the confusion of cellular arrangement, the increased volume of lipid droplets, the disappearance of some cells nuclei and the necrosis of some hepatocyte tissues occurred (Fig. 1(f) and (i)).
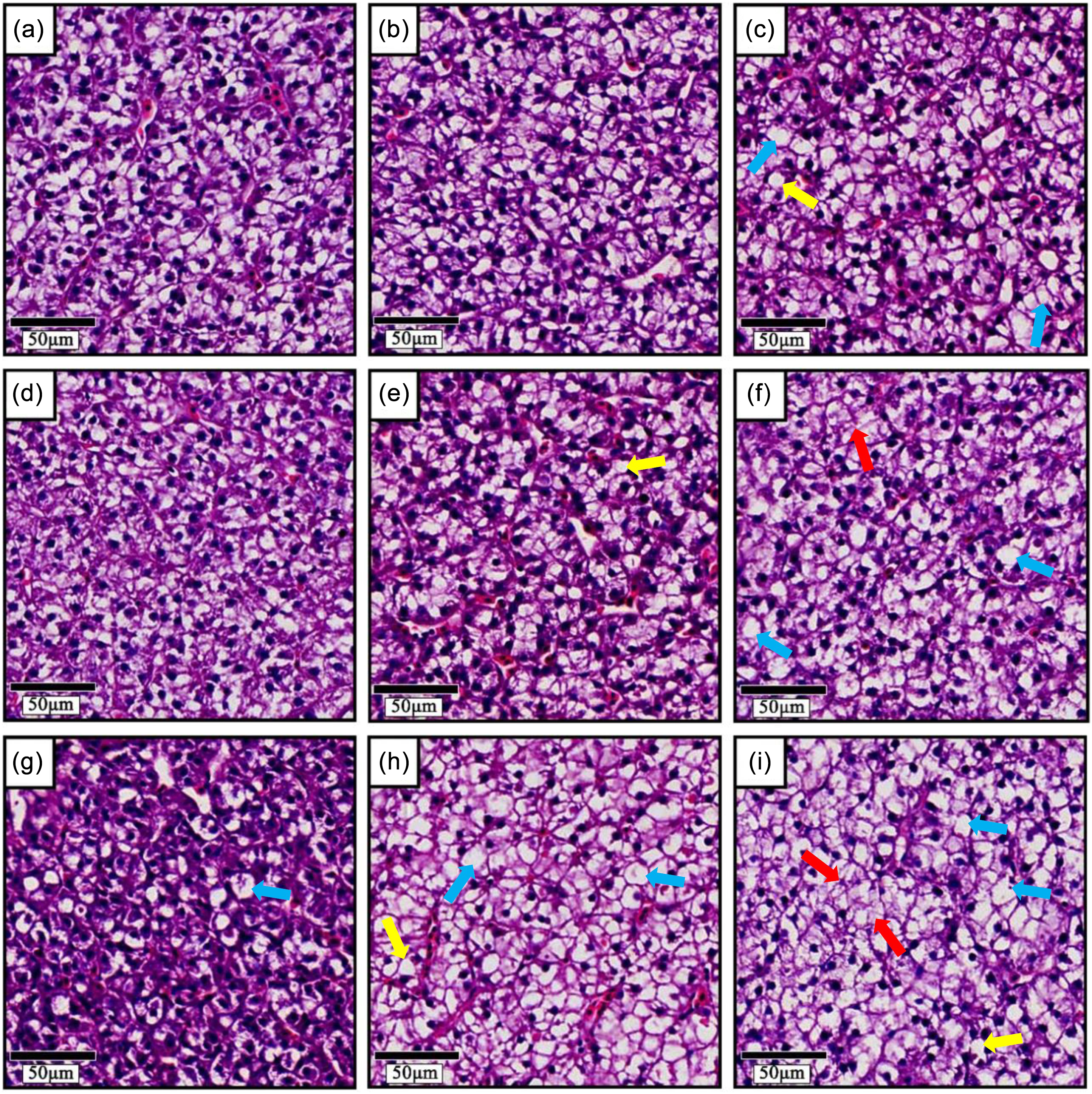
Figure 1. Effects of dietary lipid and carbohydrate interactions on the histomorphology of the liver of mandarin fish (40×). (a–c) 80 g/kg crude lipid. Carbohydrate levels were 40 g/kg, 80 g/kg, and 120 g/kg. (d–f) 120 g/kg crude lipid. Carbohydrate levels were 40 g/kg, 80 g/kg and 120 g/kg. (g–i) 160 g/kg crude lipid. Carbohydrate levels were 40 g/kg, 80 g/kg, and 120 g/kg. Lipid droplets (yellow arrow); swelling cells (green arrow); hepatocyte vacuolation (blue arrow); focal necrosis (red arrow).
Flesh texture quality
Muscle hardness of cultured fish decreased significantly with increasing levels of dietary lipid under different dietary carbohydrate levels (P < 0·05) (Table 5). However, with the dietary carbohydrate changing, it only significantly decreased in fish feeding 120 g/kg carbohydrate compared with those feeding 40 g/kg and 80 g/kg carbohydrate (P < 0·05) (Table 5). Additionally, only at 120 g/kg and 160 g/kg dietary lipid levels, muscle hardness was significantly affected by dietary carbohydrate content (P < 0·05) (Table 5). The significant interaction between the two factors was also observed in muscle resilience (P < 0·05) (Table 5). However, muscle chewiness, gumminess and shear force were only affected by dietary lipid level, which were significantly decreased with the increase of dietary lipid level (P < 0·05) (Table 5).
Table 5. Flesh texture quality of mandarin fish fed diets with varying lipid and carbohydrate levels for 10 weeks
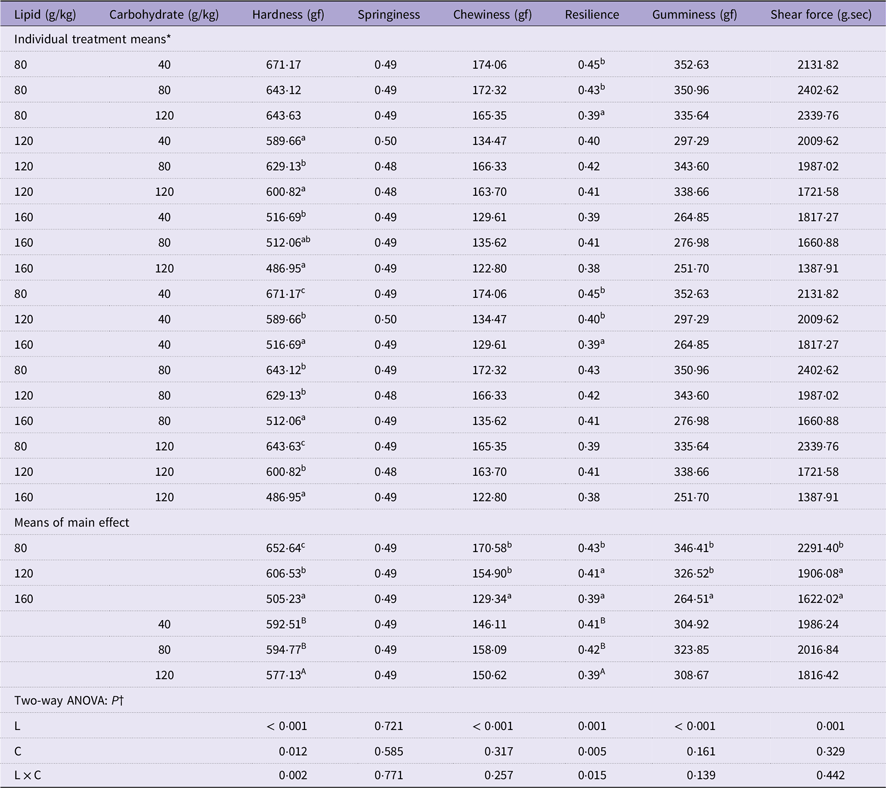
* Treatment means represent the average values of three tanks per treatment.
† Differences were regarded as significant when P < 0·05. L and C showed the main effect of each factor, and L × C indicated their interactive effect. Values (means, N = 3) with a different superscript letter are significantly different from the other dietary groups (p < 0.05).
Discussion
The result of the present study showed that the survival, growth performance and feed utilisation of cultured mandarin fish, including survival rate, specific growth rate, protein efficiency rate and feed efficiency rate, were not significantly affected by different dietary lipid and carbohydrate levels. It is worth noting that the growth performance of cultured fish in the present study was comparable to the production practice, which was outstanding compared with some current studies on mandarin fish(Reference Li, Fang and Liang29,Reference Li, Liang and Cai30) . This was possibly related to the fact that under the present culture situation, all the designed feed formulas could satisfy the growth requirement of cultured fish, and the experiment diets had a high palatability.
Considerable research has demonstrated the protein-sparing effects of dietary lipid and carbohydrates in aquatic animals(Reference Kamalam, Medale and Panserat3,Reference Turchini, Francis, Du, Halver and Hardy4,Reference Wang, Li and Wang31–Reference Welengane, Sado and Bicudo34) . The growth of cultured fish in this study was not affected by dietary lipid levels (80 g/kg–160 g/kg), indicating that the lowest lipid level in this study could satisfy the lipid requirement of mandarin fish, which was similar to the lipid requirement of red-spotted grouper(Reference Jiang, Wang and Han35), large-scale shovel-jaw fish (Onychostoma macrolepis)(Reference Gou, Chang and Deng36) and loach (Paramisgurnus dabryanus)(Reference Wang, Zhou and Zhang37). For carnivorous fish, limited glucose utilisation ability has been well illustrated, and the recommended dietary carbohydrate level should be no more than 200 g/kg(38). In the present study, the increasing carbohydrate level from 40 g/kg to 120 g/kg did not significantly affect the survival, growth performance and feed utilisation of cultured mandarin fish, which were in line with the study in Yangtze sturgeon (Acipenser dabryanus)(Reference Qu, Ke and Wen39) and hybrid snakehead (Channa maculata ♀ × Channa argus ♂)(Reference Xu, Liu and Huang40). However, dietary carbohydrate levels exceeding 100 g/kg would negatively affect the growth performance of largemouth bass(Reference Liu, Liu and Wang41) and hybrid grouper(Reference Li, Li and Zhang6), which might be because mandarin fish had a higher carbohydrate tolerance and the underlying mechanism required further exploration.
Although the growth performance and the feed utilisation were not significantly affected by dietary lipid and carbohydrate levels, significant differences were observed in body compositions among different treatment groups. In the present study, liver and muscle crude lipid content of cultured mandarin fish showed an increasing trend with higher levels of dietary lipid and carbohydrate, which could also be confirmed by the H&E staining results. This observation was similar to the study on turbot(Reference Zhang, Dan and Zhuang42), lumpfish (Cyclopterus lumpus)(Reference Berge, Zhou and Johansen43) and triploid rainbow trout (Oncorhynchus mykiss)(Reference Chen, Cao and Huang44). In addition, liver glycogen significantly increased with the increase in dietary carbohydrate level, and the same trend was found under each dietary lipid level, which was in line with the results of hybrid snakehead(Reference Xu, Liu and Huang40) and spotted sea bass (Lateolabrax maculatus)(Reference Zheng, Wang and Zhang45). Interestingly, liver glycogen was not affected by dietary lipid levels at low carbohydrate levels; however, when carbohydrates reached 120 g/kg, liver glycogen was higher in the 120 g/kg and 160 g/kg lipid groups than in 80 g/kg lipid group, which might be because that at high dietary carbohydrate level, increasing dietary lipid could negatively affect liver glycometabolism of mandarin fish, but the underline mechanism required further investigation. Furthermore, high liver lipid and glycogen content might lead to liver injury(Reference Yan, Liao and Wang8,Reference Xu, Dong and Zuo46) . In the present study, liver injury could be observed in the high lipid and high carbohydrate groups. The increased dietary lipid and carbohydrate level also reduced muscle protein content, and the increased dietary lipid level enhanced muscle lipid content, which indicated that dietary lipid and carbohydrate inclusion levels would also affect muscle compositions and further affect muscle growth of mandarin fish(Reference Huang, Jiang and Abasubong47).
Increased liver lipid and glycogen content would affect the liver antioxidant capacity of aquatic animals(Reference Li, Li and Chen12,Reference He, Tang and Xu48) . As a representative cell peroxidation product, MDA content could directly reflect peroxidation damage(Reference Ding, Yuan and Huang49). In the present study, the MDA content increased with the increase of dietary lipid and carbohydrate levels, which indicated the liver peroxidation damage induced by high dietary lipid and carbohydrate levels. The existence of antioxidant enzymes, such as CAT and SOD, could effectively reduce peroxidation damage; thus, they play an essential role in balancing the oxidation and anti-oxidation defence systems(Reference Wang, Zhang and Zhang50,Reference Escobar, Rubio and Lissi51) . CAT could catalyse H2O2 decomposition, and SOD could catalyse superoxide radical dismutation, both of which could reflect reactive oxygen species removal ability(Reference Hao and Liu52). In the present study, liver CAT activity was significantly decreased with the increase of dietary lipid and carbohydrate level, which was similar to the study on largemouth bass(Reference Zhou, Guo and Tang53), mud crab (Scylla paramamosain)(Reference Xu, Han and Li54) and spotted sea bass(Reference Zheng, Wang and Zhang45). However, the SOD activity significantly increased in both high lipid (160 g/kg) group and high carbohydrate (120 g/kg) group, which seems to be conflict to previous studies. The main reason here might be that although 160 g/kg lipid level and 120 g/kg carbohydrate level might not be the suitable level for mandarin fish, its effect was within the limit of the fish adaptive capacity, and the increased SOD activity indicated the self-regulation process, which might also be the reason that explained the unaffected growth performance of mandarin fish under different lipid and carbohydrate levels.
Since dietary lipid and carbohydrate levels significantly affected muscle protein and lipid content, both of which were essential factors affecting flesh texture quality, the flesh texture quality indexes were further analysed(Reference Liu, Deng and Pan20,Reference Gong, Weng and Wang55) . In the present study, muscle hardness and resilience decreased with the increase of dietary lipid and carbohydrate levels, which might be related to the interaction of dietary lipid and carbohydrate, which induced a decrease in muscle protein content, and this result was in line with a previous study on the relationship between muscle protein content and flesh texture(Reference Zhou, Wu and Jiang56). However, the chewiness, gumminess and shear force only react to dietary lipid changes, which might be due to the relation between flesh quality and muscle lipid content(Reference Jacob and Pethick57,Reference Wang, Su and Elzo58) .
In conclusion, dietary lipid and carbohydrate level up to 160 g/kg and 120 g/kg, respectively, would not significantly affect the growth performance and feed utilisation of mandarin fish, but high lipid and carbohydrate levels negatively affect liver antioxidant capacity and flesh quality of mandarin fish. Taking liver histology and antioxidant capacity, flesh texture quality and feed processing characteristics into account, the appropriate levels of lipid and carbohydrate in feed of mandarin fish were 120 g/kg and 80 g/kg, respectively. This study provided reliable experimental data and theoretical basis for the application of artificial compound feed for mandarin fish.
Acknowledgements
This work was financially supported by Shanghai Agricultural Science and Technology Innovation Program (K2024-02-08-00-12-F00029), Shanghai Sailing Program (24YF2717200) and the China Agriculture Research System of MOF and MARA (CARS-46).
Z. H.: investigation, data curation, formal analysis, writing – review and editing; Y. G.: conceptualisation, supervision, writing – original draft; N. Z.: investigation, methodology; Z. S.: investigation, methodology; S. L.: investigation, methodology; X. H.: conceptualisation, investigation; N. C.: conceptualisation, project administration, funding acquisition; S. L.: conceptualisation, supervision, writing – review and editing.
The experiment was conducted following the procedures set by the Animal Care and Use Committee of Shanghai Ocean University.