- AUC
area under curve
- BW
body weight
- DP
degree of polymerisation
- EI
energy intake
- GLP-1
glucagon-like peptide 1
- NDC
non-digestible carbohydrate
- FFA2 and FFA3
free fatty acid receptor 2 and 3, respectively
- OF
oligofructose
- PYY
peptide YY
- VAS
visual analogue scales
Human subjects are living in an increasingly ‘obesogenic’ environment with easily accessed, energy dense foods and sedentary lifestyles. These ‘obesogenic’ factors can override homeostatic systems resulting in a gradual increase in the population body weight (BW)(1). The need for strategies to prevent the rise in obesity is therefore becoming increasingly urgent. One strategy is to identify and develop foods that enhance satiety, thereby reducing subsequent energy intake (EI)(Reference Blundell and Halford2, Reference Cummings and Overduin3).
SCFA have been suggested to have satiety-enhancing properties, with some researchers suggesting SCFA may explain the inverse association between dietary fibre intake and BW found in some observational studies(Reference Burton-Freeman4–Reference Lutsey, Jacobs and Kori16). This review considers the evidence for SCFA having a role in appetite regulation, starting first with the description of SCFA, followed by a discussion of the SCFA-activated receptors FFA receptor 2 (FFA2) and FFA receptor 3 (FFA3), which provide a rationale that SCFA may have a role in energy homeostasis. Finally, studies that have investigated the effects of orally and colonically delivered SCFA on appetite are reviewed.
SCFA
SCFA are organic fatty acids generated in the colon when non-digestible carbohydrates (NDC) such as dietary fibre, resistant starch and inulin resist digestion and absorption in the small intestine, instead proceeding to the colon to undergo bacterial fermentation. The SCFA formed comprise between one and seven carbon units, with acetate (two carbon units) being the most predominant anion in the colon, followed by propionate (three carbon units) and then butyrate (four carbon units)(Reference Cummings, Pomare and Branch17, Reference Topping and Clifton18) (shown in Fig. 1), accounting for approximately 90–95% of total colonic SCFA(Reference Dass, John and Bassil19). Total colonic SCFA concentrations in human subjects are in the range of 60–130 mM; however, relatively low quantities reach the peripheral circulation, with normal blood concentrations in the range of 100–150 μM acetate, 4–5 μM propionate and 1–3 μM butyrate(Reference Cummings and Englyst20).
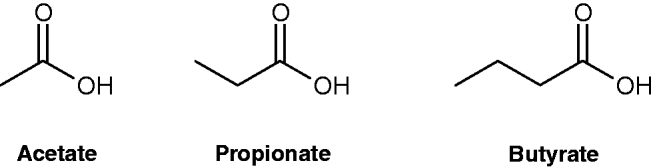
Fig. 1. Chemical structures of acetate, propionate and butyrate.
In addition to being generated from fermentable NDC, dietary SCFA sources include various foods containing SCFA, particularly foods that have been fermented. Examples include sourdough bread, vinegar and vinegar-containing products such as pickles, and some dairy products such as cheese, butter, crème fresh and soured cream. Due to the volatility of SCFA, SCFA-containing products have a distinctive odour and taste associated with them.
The SCFA receptors
In 2003, two orphan G-protein coupled receptors, FFA2 and FFA3 were identified as being activated by SCFA(Reference Brown, Goldsworthy and Barnes21–Reference Nilsson, Kotarsky and Owman23). FFA2 and FFA3 were originally known as GPR43 and GPR41, respectively(Reference Stoddart, Smith and Milligan24). Initial studies indicate that FFA2 is equally sensitive to activation by propionate, butyrate and acetate, while sensitivity for FFA3 is in the order propionate≥butyrate>acetate(Reference Brown, Goldsworthy and Barnes21–Reference Xiong, Miyamoto and Shibata26). FFA2 and FFA3 are expressed in a variety of tissues including adipose and the colon(Reference Brown, Goldsworthy and Barnes21, Reference Le Poul, Loison and Struyf22, Reference Xiong, Miyamoto and Shibata26, Reference Covington, Briscoe and Brown27).
In adipocytes, in vitro and in vivo evidence from murine models indicate that the activation of FFA3 may mediate leptin expression. In vitro, leptin expression was up-regulated following the treatment of murine adipocytes with SCFA solutions, while oral provision of propionate to mice in vivo led to elevated circulatory leptin concentrations. Propionate was the most potent ligand in vitro, and the stimulatory effects of propionate treatment were abolished in cells infected with a virus that targeted FFA3 mRNA, suggesting that FFA3 is the relevant receptor(Reference Xiong, Miyamoto and Shibata26, Reference Covington, Briscoe and Brown27).
In the colon, molecular investigations of cell lines of both rat and human origin have shown that FFA2 and FFA3 are co-localised with the anorexigenic ‘ileal brake’ gut hormone peptide YY (PYY) in enteroendocrine l-cells(Reference Karaki, Mitsui and Hayashi28–Reference Tazoe, Otomo and Karaki30). When administered in vitro to a vascularly infused rat colon lumen, solutions of propionate, butyrate, but not acetate stimulate PYY secretion(Reference Plaisancié, Dumoulin and Chayvialle31), with no significant release of the gut hormone glucagon-like protein 1 (GLP-1)(Reference Plaisancié, Dumoulin and Chayvialle32). In vivo, luminal administration of SCFA solutions to live rats and large white pigs have been shown to significantly increase subsequent blood PYY concentrations(Reference Cherbut, Ferrier and Rozé33, Reference Cuche and Malbert34) and reduce upper gastrointestinal motility(Reference Cherbut, Ferrier and Rozé33), an effect reproduced with a PYY infusion(Reference Cherbut, Ferrier and Rozé33). As sensitivity to stimulation in vitro is in the order propionate≥butyrate>>acetate for PYY secretion(Reference Karaki, Mitsui and Hayashi28–Reference Tazoe, Otomo and Karaki30), FFA3 is implicated as the responsible receptor for modulating these effects.
Thus, SCFA may have a role in energy homeostasis via FFA3 activation in adipocytes to stimulate leptin expression(Reference Xiong, Miyamoto and Shibata26, Reference Covington, Briscoe and Brown27) and via FFA2 and FFA3 activation in the colonic mucosa to enhance production of the anorexigenic gut hormone PYY(Reference Karaki, Mitsui and Hayashi28–Reference Cuche and Malbert34).
The role of SCFA in appetite regulation
As outlined above, the presence of the SCFA-activated receptors FFA2 and FFA3 in adipocytes(Reference Xiong, Miyamoto and Shibata26, Reference Covington, Briscoe and Brown27) and co-localised with PYY in the colonic mucosa(Reference Karaki, Mitsui and Hayashi28–Reference Cuche and Malbert34) is suggestive of a role for SCFA in energy homeostasis. The effects of SCFA on appetite have been investigated both by (1) oral provision of SCFA and (2) colonic delivery of SCFA via fermentable NDC such as inulin-type fructans as discussed later.
The effect of orally delivered SCFA on appetite
A number of studies investigating the effects of orally ingested SCFA, in particular acetate (delivered as vinegar)(Reference Hlebowicz, Lindstedt and Björgell35–Reference Kondo, Kishi and Fushimi38) and propionate (delivered as sodium (Na) propionate)(Reference Frost, Brynes and Dhillo39–Reference Liljeberg, Lonner and Bjorck41), on subsequent satiety have been published (Table 1). These studies will be reviewed below.
Table 1. Summary of human clinical studies investigating the acute effects of oral SCFA supplementation on appetite

AUC, area under curve; EI, energy intake; VAS, visual analogue scales; GLP-1, glucagon-like peptide 1; ↑, higher; ↓ lower.
Orally delivered acetate and appetite effects
The effects of long-term supplementation with apple cider vinegar as a source of acetate on anthropometric measures in human subjects were reported in a recently published three-way parallel study(Reference Kondo, Kishi and Fushimi38). In this study, healthy obese Japanese volunteers were randomised to the control (n 58, 50 completed), low-dose (n 59, 54 completed) or high-dose (n 58, 51 completed) treatment, consuming a beverage containing 0, 15 or 30 ml apple cider vinegar, respectively (equivalent to 0, 12·5 and 25 mmol acetate) daily for 12 weeks. At the end of the intervention, those in both the low- and high-dose treatment groups had a significantly lower BW, BMI, % body fat, waist and hip circumference, waist:hip ratio, visceral fat area, subcutaneous fat area and TAG concentrations relative to control. Additionally total fat area and systolic blood pressure were significantly lower in the high-dose group relative to control(Reference Kondo, Kishi and Fushimi38).
This study therefore provides some compelling evidence that vinegar as a source of acetate may influence appetite. The acute effects of vinegar supplementation on appetite and satiety in human subjects have been investigated in a few studies (summarised in Table 1) that will be reviewed later(Reference Hlebowicz, Lindstedt and Björgell35–Reference Kondo, Kishi and Fushimi38).
In an acute four-way crossover study, Ostman et al. provided human volunteers (n 12) bread (containing 50 g carbohydrate) soaked in 0 g (control), 18, 23 and 28 g vinegar (equivalent to 0, 18, 23 and 28 mmol acetic acid) for their breakfast following an overnight fast. They reported a dose–response increase in the area under curve (AUC) for satiety ratings (Fig. 2), with a significantly higher AUC following the 28 g dose relative to control(Reference Ostman, Granfeldt and Persson37).

Fig. 2. Postprandial satiety score area under curve (AUC) following ingestion of bread (containing 50 g carbohydrate) soaked in 0, 18, 23 and 28 g white wine vinegar, supplying 0, 18, 23 and 28 mmol acetate, respectively. A dose–response increase in the satiety AUC was observed. Values are mean with error bars representing the SEM. Taken from Ostman et al. (Reference Ostman, Granfeldt and Persson37).
The same group also investigated the effects of vinegar and form of wheat grain (refined, milled or wholegrain). In this four-way crossover study, participants (n 15, 13 completed) consumed a test breakfast comprising white, milled grain or wholegrain bread soaked in 28 g vinegar (supplying 28 mmol acetate) compared to white bread without vinegar (control). The postprandial satiety score AUC was significantly higher following wholegrain bread soaked in vinegar relative to the other three treatments, with none of the other treatments altering satiety(Reference Hlebowicz, Lindstedt and Björgell35). However, as wholegrain bread without added vinegar was not investigated, it is not possible to conclude whether the increased satiety arose from the wholegrain structure of the bread alone or it was an additive effect of the grain structure with the vinegar.
A two-by-two crossover study by a different group investigated the additive effects of acetic acid (28 mmol) and cinnamon mixed into a glucose drink and milk rice pudding. This study reported the satiety incremental AUC did not significantly differ between treatments, although a main effect of acetic acid approaching significance (P=0·064) was found(Reference Mettler, Schwarz and Colombani36).
However, none of these previous studies investigated quantitative effects on appetite. In addition, subjective effects on appetite were investigated using a single rating bipolar scale(Reference Hlebowicz, Lindstedt and Björgell35, Reference Ostman, Granfeldt and Persson37) or point scale(Reference Mettler, Schwarz and Colombani36), rather than a variety of appetite-related visual analogue scale (VAS) questionnaires.
In our own laboratories, we carried out an acute three-way crossover study with two main objectives: to investigate the acute effects of acetate supplementation on subjective and quantitative measures of appetite, and to investigate the influence of product palatability. Subjective appetite effects were assessed using a variety of appetite-related VAS questionnaires. Alongside a standard breakfast (jam sandwiches) normal weight unrestrained eaters (n 16) were provided a drink of sugar-free orange squash containing 25 g vinegar (supplying 25 mmol acetate) in a more palatable (Pal, vinegar divided across two drinks) and unpalatable (Unpal, vinegar in a more concentrated form in one drink) form compared to control (drink with no added vinegar). Palatability ratings for the breakfast were significantly lower for the Unpal treatment compared to both control and Pal.
Vinegar treatment significantly lowered subjective appetite VAS ratings for hunger (P=0·045) and the desire to eat (P=0·036) and increased ratings for fullness (P<0·0001). Mean ad libitum EI of a large pre-weighed homogenous pasta meal provided 3 h postprandially was significantly influenced by vinegar treatment (P=0·022). The mean intake was lowest with Unpal treatment, followed by Pal, with the highest mean intake occurring with the control treatment, which was significantly higher than with Unpal treatment. Furthermore, a pooled correlation analysis found significant correlations between breakfast palatability ratings and various appetite measures including 24 h VAS AUC and ad libitum EI (J Darzi, GS Frost and MD Robertson, unpublished results). Our findings therefore indicate that while vinegar as a source of acetate may reduce appetite, this effect may be due, at least in part, to palatability effects of the vinegar-containing test products.
Orally delivered propionate and appetite effects
Two acute crossover studies from the same group have examined effects on appetite of bread baked with added Na propionate when consumed as part of a mixed meal for breakfast. In the first study, participants (n 11) attended on six occasions and were provided breakfast made using wholemeal bread (control) or the same bread with added Na propionate (low and high dose), sourdough, lactic acid or Ca lactate(Reference Liljeberg, Lonner and Bjorck41). In the second study, participants (n 12) attended on three occasions and were provided breakfast prepared using wholemeal bread (control) or the same bread with added Na propionate (high dose only) or lactic acid(Reference Liljeberg and Bjorck40). The actual dose of Na propionate was not reported by the authors, but is calculated as 15 and 45 mmol for low- and high-dose breads, respectively. In both studies, the addition of Na propionate was reported to increase the postprandial satiety rating AUC in a dose–response manner (Fig. 3(A)), with a significantly higher-satiety AUC reported following the high dose of Na propionate bread relative to control(Reference Liljeberg and Bjorck40, Reference Liljeberg, Lonner and Bjorck41). However, at the same time, the acceptability rating score was reduced by the addition of Na propionate in a dose–response manner (Fig. 3(B))(Reference Liljeberg, Lonner and Bjorck41).

Fig. 3. (A) Postprandial satiety score area under curve (AUC) and (B) acceptability score following ingestion of a mixed breakfast made with wholemeal bread (control) or bread baked with added Na propionate in a low (approximately 15 mmol) and high (approximately 45 mmol) dose. A dose–response increase in satiety was found, which was accompanied by a dose–response decrease in the acceptability score. *Mean values for the high-dose bread differed significantly from control (P<0·05). Values are mean with error bars representing the SEM. Adapted from Liljeberg et al. (Reference Liljeberg, Lonner and Bjorck41).
The provision of Na propionate is also associated with increased nausea. When volunteers (n 10) ingested 3 g Na propionate (equivalent to 31 mmol) combined with 30 g sunflower oil (as a source of PUFA) mixed into a pasta meal at breakfast, postprandial nausea ratings were significantly increased(Reference Frost, Brynes and Dhillo39). In this study, the postprandial incremental AUC for the appetite gut hormone GLP-1 was significantly increased by Na propionate and PUFA treatment, although subjective appetite ratings and EI of an ad libitum test meal served 4 h postprandially did not differ from the control (pasta with no added Na propionate/PUFA)(Reference Frost, Brynes and Dhillo39). However, as PUFA was delivered alongside the Na propionate, it was not possible to determine whether the observed effects were attributed to the added PUFA, propionate, or both and if the higher energy content of the Na propionate/PUFA pasta test meals had an effect.
In our own laboratories we carried out an acute two-way crossover study to investigate the effects of providing a palatable propionate-rich sourdough bread compared to an equally palatable and visually identical non-sourdough control bread on subjective and quantitative measures of appetite. Prior sensory evaluation of the bread products determined the sourdough and control breads to be equally acceptable. Healthy, normal weight unrestrained eaters (n 20) were provided a breakfast comprising jam sandwiches made using the palatable sourdough bread (supplying a total of 6·0 mmol propionate) or the non-sourdough control bread. In this study, we found that the palatable propionate-rich sourdough bread did not influence appetite, with no significant differences between treatments on postprandial appetite VAS ratings, ad libitum EI 3 h postprandially or on 24 h intake. Our findings therefore indicate that when provided in a palatable form, propionate does not appear to influence appetite(Reference Darzi, Frost and Robertson42). This implies that previous reports of Na propionate ingestion being associated with increased satiety(Reference Liljeberg and Bjorck40, Reference Liljeberg, Lonner and Bjorck41) may be a test product palatability effect, at least in part.
Oral SCFA and appetite summary
In summary, initial studies indicate that oral SCFA may enhance satiety(Reference Hlebowicz, Lindstedt and Björgell35–Reference Liljeberg, Lonner and Bjorck41). However, our findings suggest that product palatability may explain the appetite effects of oral SCFA rather than being a physiological effect of SCFA (J Darzi, GS Frost and MD Robertson, unpublished results).
Studies investigating the link between sensory properties of food and appetite suggest that sensory properties influence food choice and quantities consumed(Reference Sørensen, Møller and Flint43). A pooled analysis of data from studies in which investigators manipulated food palatability concluded there was a strong linear relationship between the change in rated palatability and difference in food intake following food manipulation (Fig. 4)(Reference Yeomans44). This provides a rationale for our hypothesis that oral SCFA palatability explains effects on appetite and satiety, most likely via mechanisms mediated at the cephalic phase.
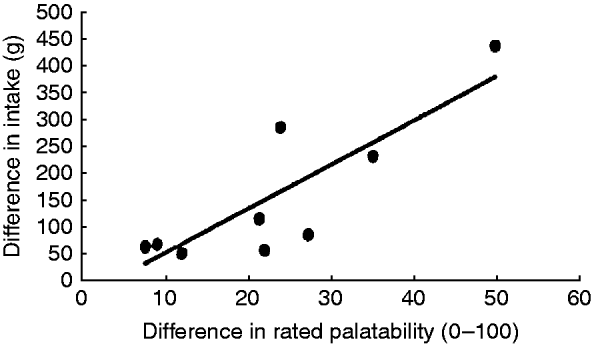
Fig. 4. Relationship between change in palatability ratings and food intake when palatability is manipulated. Each data point represents a published study. Taken from Yeomans(Reference Yeomans44).
Colonic delivery of SCFA to investigate appetite effects, focusing on inulin-type fructans
There is growing evidence to indicate dietary fibre and other NDC may have a role in BW regulation, with a number of studies suggesting the intake of dietary fibre and/or wholegrains are inversely associated with BW and/or BMI(Reference Burton-Freeman4–Reference Lutsey, Jacobs and Kori16). Dietary fibre and other NDC may therefore potentially have a role in the prevention and treatment of obesity.
Furthermore, various studies have demonstrated dietary fibre may enhance satiety and reduce appetite, thus helping to control subsequent food intake(Reference Burton-Freeman4–Reference Howarth, Saltzman and Roberts6, Reference Pereira and Ludwig10, Reference Slavin11, Reference Berti, Riso and Brusamolino45). However, the mechanisms by which this may occur are not fully understood. It is possible that SCFA generated during colonic fermentation may mediate effects on appetite via interaction with the SCFA-activated receptors FFA2 and FFA3 in colonic enteroendocrine l-cells, and FFA3 in adipocytes to modulate PYY and leptin production, respectively, as discussed earlier.
This review will now focus on a particular group of fermentable NDC, the inulin-type fructans, which as well as being classified as a dietary fibre is also classified as being a prebiotic.
What are inulin-type fructans? Inulin-type fructans are linear oligo- and polysaccharides comprising mainly β-(2→1) fructosyl–fructose glycosidic linkages (Fig. 5)(Reference Roberfroid46). As digestive enzymes are specific for α-glycosidic bonds, inulin-type fructans resist enzymatic hydrolysis in the small intestine, and therefore proceed undigested to the colon where they are fermented(Reference Kelly47). Inulin-type fructans are considered long chain with a degree of polymerisation (DP)≥10, medium chain with a DP between five and nine and short chain with a DP between two and four. Various inulin-type fructan preparations with differing physical, chemical and physiological properties are available commercially including(Reference Kelly47, Reference Niness48):
High DP inulin (e.g. Inulin HP): Long-chain only inulin-type fructan preparation with a DP≥10 and an average DP of 25;
Oligofructose (OF) (e.g. Raftilose P95): Short- and medium-chain preparation with a DP ranging from 2 to 10 and an average DP of 4;
Mixed inulin-type fructan (e.g. Synergy 1): A mixture of low-, medium- and high-DP inulin-type fructans.

Fig. 5. Structure of inulin-type fructans. Inulin-type fructans are composed of β-(2→1) linked fructose units with either a terminal (A) glucose or (B) fructose unit.
Inulin-type fructans and effects on appetite
Initial rodent model studies give evidence that inulin-type fructans may enhance satiety(Reference Cani, Knauf and Iglesias49–Reference Cani, Neyrinck and Maton54). Chronic OF supplementation in rodents significantly decreases EI(Reference Cani, Dewever and Delzenne50, Reference Cani, Hoste and Guiot51, Reference Cani, Neyrinck and Maton54), suppresses BW gain(Reference Cani, Knauf and Iglesias49, Reference Cani, Hoste and Guiot51, Reference Cani, Neyrinck and Maton54) (although not in all cases(Reference Cani, Dewever and Delzenne50, Reference Kok, Morgan and Williams53)), suppresses fat mass gain(Reference Cani, Knauf and Iglesias49–Reference Cani, Hoste and Guiot51, Reference Cani, Neyrinck and Maton54), increases proximal colon GLP-1 (7–36) amide concentrations(Reference Cani, Knauf and Iglesias49–Reference Cani, Hoste and Guiot51,Reference Kok, Morgan and Williams53, Reference Cani, Neyrinck and Maton54), increases portal plasma GLP-1 (7–36)(Reference Cani, Dewever and Delzenne50, Reference Cani, Hoste and Guiot51, Reference Cani, Neyrinck and Maton54) and gastric-inhibitory polypeptide(Reference Kok, Morgan and Williams53) concentrations, reduces plasma ghrelin concentrations(Reference Cani, Dewever and Delzenne50) and increases the caecum mass(Reference Cani, Dewever and Delzenne50, Reference Cani, Hoste and Guiot51, Reference Kok, Morgan and Williams53). Most studies examined OF, with few investigating higher DP inulin-type fructans (e.g. inulin HP)(Reference Cani, Dewever and Delzenne50, Reference Daubioul, Rousseau and Demeure52). Supplementing rats diets with Synergy 1 (mixture of low- and high-DP inulin-type fructans) resulted in significantly suppressed BW gain(Reference Daubioul, Rousseau and Demeure52) and fat mass gain(Reference Cani, Dewever and Delzenne50), reduced EI(Reference Cani, Dewever and Delzenne50, Reference Daubioul, Rousseau and Demeure52), reduced proximal colon GLP-1 (7–36) concentrations(Reference Cani, Dewever and Delzenne50) and reduced caecum mass(Reference Cani, Dewever and Delzenne50). Furthermore, supplementation with inulin HP significantly suppressed BW gain and increased caecum mass, but did not influence proximal colon GLP-1 (7–36) concentrations or fat mass gain(Reference Cani, Dewever and Delzenne50).
Following on from these promising data from animal models, a few human studies have investigated acute (Table 2) and more have investigated chronic (Table 3) effects of supplementing with inulin-type fructans on appetite and/or anthropometrics. A review of these studies now follows.
Table 2. Summary of human clinical studies investigating acute effects of inulin-type fructan supplementation on appetite

E, energy; EI, energy intake; OF, oligofructose; AUC, area under curve.
Table 3. Summary of human clinical studies investigating chronic effects of inulin-type fructan supplementation on appetite

GERD, gastrooesophageal reflux disease; ↑ higher; ↓, lower; GLP, glucagon-like peptide; AUC, area under curve; OF, oligofructose; PYY, peptide YY; CCK, cholecystokinin; GIP, gastric-inhibitory polypeptide; BW, body weight.
Three different studies have been published that investigated acute effects of ingesting inulin-type fructans on subsequent appetite (Table 2). Peters et al. provided volunteers (n 21) with a meal replacement bar containing 8 g OF or a non-OF control to be eaten in the free-living setting at breakfast and lunch on day 1 and breakfast only on day 2. No significant effects of treatment were found on satiety ratings or EI at an ad libitum buffet meal provided 4 h postprandially(Reference Peters, Boers and Haddeman55).
By contrast, Archer and colleagues reported 24 h EI was significantly lowered by supplementation with 24 g inulin HP when supplied to volunteers (n 33) as a fat replacer in a sausage patty, relative to a full-fat patty (control). Satiety ratings were not significantly altered(Reference Archer, Johnson and Devereux56). However, control and inulin-containing sausage patties were not matched for energy in this study.
Similarly, consumption of yoghurt containing 6 g inulin-type fructans (type used not specified) significantly decreased subsequent ad libitum EI in a controlled setting relative to ‘no preload’ (control) in healthy volunteers (n 38)(Reference Perrigue, Monsivais and Drewnowski57). However, the overall EI including the preload and the ad libitum intake was more than the ‘no preload’ condition.
A larger number of chronic supplementation studies have been published investigating effects on appetite (Table 3), again with variable results and study limitations, as reviewed below.
Let us first take a look at the chronic effects of OF supplementation on subjective and quantitative appetite measures. In a two-way crossover study, Cani and co-workers reported that daily supplementation of healthy volunteers (n 10) with 16 g OF for 2 weeks significantly lowered ad libitum EI at breakfast and lunch and the mean daily EI relative to control (16 g maltodextrin)(Reference Cani, Joly and Horsmans58). Relative to control, OF supplementation also significantly increased satiety ratings after breakfast and dinner, and reduced hunger and prospective consumption ratings following dinner(Reference Cani, Joly and Horsmans58). However, as preload test meals were provided ad libitum rather than being a fixed, standardised preload, the postprandial appetite ratings are difficult to interpret.
Similarly, a recent parallel study reported supplementation of volunteers (n 51) with 14 g Jerusalem Artichoke concentrate (containing OF) daily for 12 weeks reduced rated hunger(Reference Antal, Regöly-Mérei and Biró59). However, it was unclear whether effects on hunger were significant.
By contrast, in a two-way parallel study, Parnell and Reimer reported daily supplementation of healthy overweight volunteers (n 48) for 12 weeks with 21 g OF did not significantly alter satiety ratings and EI relative to control (7·89 g maltodextrin), although the PYY AUC was significantly higher(Reference Parnell and Reimer60). However, this parallel study was not sufficiently powered to assess subjective appetite effects(Reference Flint, Raben and Blundell61).
Effects of OF supplementation on gut peptide production have also been investigated by Piche and colleagues in patients with gastrooesophageal reflux disease. In a two-way crossover study, participants (n 9) ingested 19·8 g OF or sucrose (control) for 7 d. The postprandial plasma GLP-1 AUC was significantly higher in response to a meal tolerance test following OF supplementation relative to control; however both PYY and cholecystokinin were not significantly influenced by treatment(Reference Piche, des Varannes and Sacher-Huvelin62).
Let us now take a look at the effects of mixed inulin-type fructans. In a two-way parallel chronic study, Cani and co-workers asked volunteers to supplement their usual diet with 16 g Synergy-1 (n 5) or 16 g Maltodextrin (control) (n 5) daily for 12 weeks. At the end of the supplementation period volunteers attended a meal tolerance test challenge. The authors reported postprandial subjective appetite ratings did not differ between the two treatments during the meal tolerance test, although the postprandial GLP-1 and PYY response was significantly higher with Synergy-1 treatment(Reference Cani, Lecourt and Dewulf63). However, this study was underpowered and the meal tolerance test was supplied ad libitum making interpretation of postprandial data difficult.
In a two-way crossover study Whelan and colleagues asked healthy volunteers (n 11) to consume a standard enteral formula (control) or a modified enteral formula with added pea fibre, inulin and OF for 2 weeks as their sole nutrition source(Reference Whelan, Efthymiou and Judd64). Significantly increased daily mean and minimum fullness and minimum satiety ratings were observed when the modified enteral formula was consumed relative to control(Reference Whelan, Efthymiou and Judd64). However, as pea fibre was also included in the active treatment, observed results may not have been solely due to the inulin-type fructans.
Less compelling is anthropometric and food intake data from chronic intervention studies, although these were not the main outcomes of interest for all studies(Reference Alles, de Roos and Bakx65–Reference Luo, Van Yperselle and Rizkalla72) except for two(Reference Antal, Regöly-Mérei and Biró59, Reference Parnell and Reimer60). One study reported daily supplementation with 14 g Jerusalem Artichoke concentrate (containing OF) for 12 weeks significantly reduced BMI and % body fat levels(Reference Antal, Regöly-Mérei and Biró59), while another reported daily supplementation with 21 g OF significantly reduced BW, fat mass and central fat mass(Reference Parnell and Reimer60). However, none of the other studies found a significant influence of inulin-type fructan supplementation on BW post-intervention(Reference Antal, Regöly-Mérei and Biró59, Reference Alles, de Roos and Bakx65, Reference Giacco, Clemente and Luongo66, Reference Forcheron and Beylot68–Reference Luo, Van Yperselle and Rizkalla72) or on food intake during the intervention(Reference Alles, de Roos and Bakx65–Reference Forcheron and Beylot68).
In our own laboratories, we carried out an acute three-way crossover study to investigate the effects on subjective and quantitative measures of appetite of providing inulin HP or another NDC l-rhamnose as a component of breakfast and lunch compared to a visually identical control (no NDC) following a 6 d run-in period. l-rhamnose was investigated as previously published data suggest propionate production is favoured during l-rhamnose fermentation, both in vitro (Reference Fernandes, Rao and Wolever73) and in vivo (Reference Vogt, Pencharz and Wolever74). Propionate is the most potent ligand for the SCFA receptor FFA3 and appears to promote leptin expression(Reference Xiong, Miyamoto and Shibata26, Reference Covington, Briscoe and Brown27) and the production of PYY(Reference Karaki, Mitsui and Hayashi28–Reference Cuche and Malbert34) as discussed earlier, providing a rationale l-rhamnose may enhance appetite. Inulin HP was investigated due to the lack of previous data regarding effects on appetite.
During an acute meal challenge, healthy, normal weight unrestrained eaters (n 13) ingested 22·4 g inulin HP or 25·5 g l-rhamnose as a split dose at a standard breakfast and 180 min later at a standard lunch. We found neither treatment influenced appetite, with no significant differences between treatments for postprandial appetite VAS ratings, ad libitum EI 7 h postprandially, intake during the 24 h period following the breakfast preload nor on the mean daily intake during the run-in period (J Darzi, GS Frost and MD Robertson, unpublished results).
Colonic SCFA and appetite summary
While a number of studies have investigated the influence of supplementation with inulin-type fructans on markers of appetite in human subjects, the results to date are contradictory making it difficult to draw firm conclusions. This is in part due to variable dosages and differing choices of inulin-type fructan supplements.
Conclusion
The presence of the SCFA-activated G-coupled protein receptors, FFA2 and FFA3 in adipocytes and the colonic mucosa provide a rationale that SCFA may influence appetite and energy homeostasis. Initial data from observational studies that found dietary fibre and wholegrain intake is inversely related to BW and/or BMI, and from animal studies that found ingestion of inulin-type fructans on reduced EI and BW, provided initial evidence that colonically derived SCFA may influence appetite. However, as reviewed in this paper, these findings are not equivocally corroborated in human intervention studies, and our findings from oral SCFA supplementation studies are suggestive that product palatability may explain the observed effects of oral SCFA ingestion on appetite via cephalic-phase-initiated mechanisms. Therefore, in conclusion, the findings from this review do not support a role for SCFA in appetite regulation.
Acknowledgements
We are grateful to all the volunteers who participated in our studies. J. D. wrote the paper and had primary responsibility for final content. M. D. R. and G. S. F. were involved in refining the paper. All authors read and approved the final manuscript. The authors declare no conflicts of interest. J. D. was supported by an educational fellowship from Premier Foods.