The burgeoning field of phylogenetic paleoecology (Lamsdell et al. Reference Lamsdell, Congreve, Hopkins, Krug and Patzkowsky2017) represents a synthesis of the related but differently focused fields of macroecology (Brown Reference Brown1995) and macroevolution (Stanley Reference Stanley1975). Through a combination of the data and methods of both disciplines, phylogenetic paleoecology leverages phylogenetic theory and quantitative paleoecology to explain the temporal and spatial variation in species diversity, distribution, and disparity. Phylogenetic paleoecology is ideally situated to elucidate many fundamental issues in evolutionary biology, including the generation of new phenotypes and occupation of previously unexploited environments; the nature of relationships among character change, ecology, and evolutionary rates; determinants of the geographic distribution of species and clades; and the underlying phylogenetic signal of ecological selectivity in extinctions and radiations. This is because phylogenetic paleoecology explicitly recognizes and incorporates the quasi-independent nature of evolutionary and ecological data as expressed in the dual biological hierarchies (Eldredge and Salthe Reference Eldredge and Salthe1984; Congreve et al. Reference Congreve, Falk and Lamsdell2018; Fig. 1), incorporating both as covarying factors rather than focusing on one and treating the other as error within the dataset.

Figure 1. Depiction of the genealogical and ecological hierarchies. Organisms act as the sole interactors within the system, occurring in both the ecological and the genealogical hierarchies, and therefore act as the only unit to be under the direct influence of both historically contingent (genealogical) processes and deterministic (matter/energy) factors. This highlighted relationship between the hierarchies is otherwise known as the process of natural selection. (In color online.)
As well as making use of ecological and phylogenetic data, phylogenetic paleoecology incorporates modern statistical methods from paleoecology and biogeographic studies (Legendre Reference Legendre, Garbary and South1990; Croft et al. Reference Croft, Su and Simpson2018) along with phylogenetic comparative methods (Cornwell and Nakagawa [Reference Cornwell and Nakagawa2017], which can be readily applied to paleontological data; Bapst Reference Bapst and Garamszegi2014) to determine whether similarity in traits between species is due to close evolutionary relationships or selective processes. Combining methods and multiple lines of data (Fig. 2) permits analysis of evolutionary dynamics to determine drivers of evolutionary change and the relative import of abiotic, biotic, and contingent historical processes in guiding evolutionary and ecological shifts across space and time.
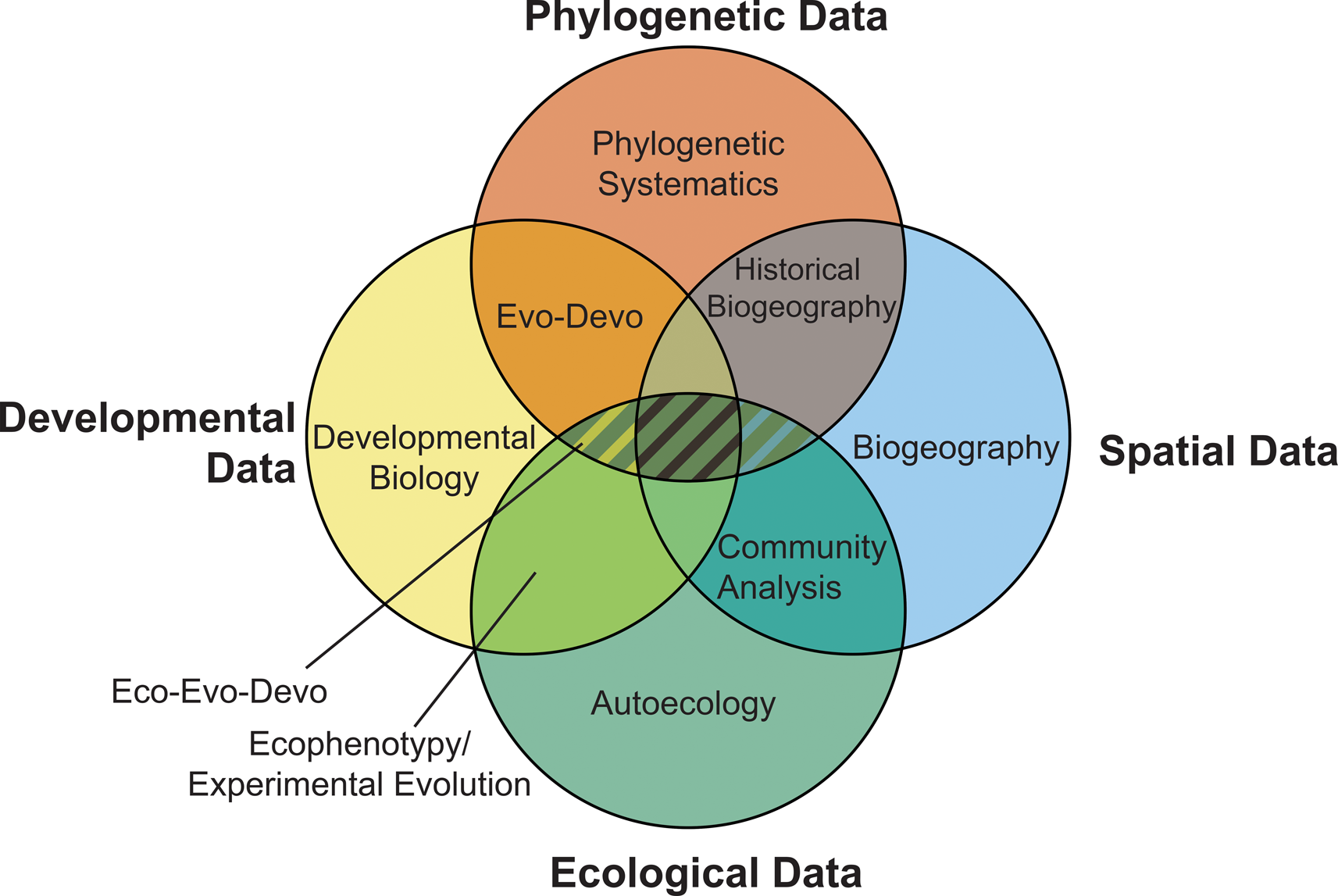
Figure 2. The four primary sources of data used in evolutionary study and the potential relationships between them. Fields of study utilizing a given data source are labeled, with fields that incorporate multiple sources of data accommodated within the regions of overlap. Phylogenetic paleoecology occupies the intersection between phylogenetic and ecological data and can incorporate data and aspects from each of the fields of study encompassed within the hatched region. (In color online.)
Although only recently synthesized into a distinct research field, phylogenetic paleoecology owes its genesis to the advent of paleobiology. Many of the methodologies used in phylogenetic paleoecology parallel those used in biology, and a number of paleobiological studies have incorporated what we would now recognize as a phylogenetic paleoecology approach. Pioneering work by Elizabeth Vrba and Blaire Van Valkenburgh explicitly considered macroecological phenomena within the framework of species relationships. Vrba's theoretical work on sorting and selection (Vrba Reference Vrba, Ho and Saunders1984b,Reference Vrbac, Reference Vrba1989; Vrba and Eldredge Reference Vrba and Eldredge1984; Vrba and Gould Reference Vrba and Gould1986), as exemplified by her exploration of the ecological and phylogenetic determinants of survivorship and species longevity in bovids (Vrba Reference Vrba, Eldredge and Stanley1984a), demonstrated the quasi-independence of ecological and evolutionary data and how phylogeny can be critical in identifying contingent historical or ecological determinants of macroevolutionary change. Van Valkenburgh's extensive study of carnivoran evolution (Van Valkenburgh Reference Van Valkenburgh1991, Reference Van Valkenburgh1995, Reference Van Valkenburgh1999) and recognition of a macroevolutionary ratchet in canids (Van Valkenburgh et al. Reference Van Valkenburgh, Wang and Damuth2004) established the importance of an accurate understanding of species relationships for recognizing evolutionary trends. While these initial studies used taxonomy as a proxy for phylogenetic relationships, later work emphasized the use of phylogenetic topologies in macroecological studies (Wang et al. Reference Wang, Tedford, Van Valkenburgh, Wayne, Macdonald and Sillero-Zubiri2004; Friscia et al. Reference Friscia, Van Valkenburgh and Beknevicius2007; Slater and Van Valkenburgh Reference Slater and Van Valkenburgh2008; Slater et al. Reference Slater, Dumont and Van Valkenburgh2009). More recently, an increasing number of studies have analyzed ecological trends within a phylogenetic context, applying the methodology to diverse groups such as chelicerate arthropods (Lamsdell and Braddy Reference Lamsdell and Braddy2010; Lamsdell Reference Lamsdell2016), ankylosaurian dinosaurs (Arbour et al. Reference Arbour, Zanno and Gates2016), early vertebrates (Sallan et al. Reference Sallan, Friedman, Sansom, Bird and Sansom2018), and crocodyliform archosaurs (Melstrom and Irmis Reference Melstrom and Irmis2019).
From these beginnings, phylogenetic paleoecology has the potential to contribute to a diverse set of subdisciplines within paleobiology and evolutionary biology. As in any field, the directions that the research takes will be in a large part dictated by the experiences and interests of the people who practice it, and one of the main strengths of phylogenetic paleoecology is the diverse nature of the researchers applying the method with regard to their backgrounds, research interests, and taxonomic expertise. We recognize the potential for exciting future developments in phylogenetic paleoecology that include contributions to a variety of interdisciplinary fields. Particularly, we expect phylogenetic paleoecology will synergize strongly with: (1) ecological evolutionary developmental biology (eco-evo-devo; Gilbert et al. Reference Gilbert, Bosch and Ledón-Rettig2015) in providing a comprehensive framework for evaluating links between ecology, development, adaptation, and the occurrence of evolutionary novelty (Erwin Reference Erwin2015, Reference Erwin2020) through deep time; (2) studies into the causal factors underlying evolutionary radiations, through combining sources of data to differentiate between models of hierarchical interactions (the “sloshing bucket”; Eldredge Reference Eldredge, Crutchfield and Shuster2003, Reference Eldredge2008) and biogeographic complexity (Stigall et al. Reference Stigall, Bauer, Lam and Wright2017) or biogeographic hotspots (e.g., Crame Reference Crame2002), which act as a potential merger between the two approaches; and (3) conservation paleobiology (Dietl et al. Reference Dietl, Kidwell, Brenner, Burney, Flessa, Jackson and Koch2015; Kosnik and Kowalewski Reference Kosnik and Kowalewski2016), which will benefit from the integration of nonanalogous communities and ecosystems into modern conservation models, as well as facilitating discrimination of the impact of environmental preference, evolutionary history, and developmental constraints on current, past, and future species distributions. The interdisciplinary nature of these types of investigation and the need for well-vetted data means that the future of the field is likely not composed of studies confined to single papers focusing on a full synthesis of a given taxonomic group or historical event, but rather multiple individual (but integrated) publications working toward an overarching synthesis. This type of work will require concerted collaboration between research groups with different skill sets sharing information and methods to publish a suite of interrelated studies that build upon one another.
In 2018, a session was convened as part of the Geological Society of America Annual Meeting in Indianapolis, Indiana, to bring together paleobiologists utilizing phylogenetic paleoecology and showcase cutting-edge research within the field. This special issue compiles the results of this meeting and subsequent collaborations.
The papers in this special issue represent the full range of disciplines encompassed within the field of phylogenetic paleoecology and can broadly be divided into three intergrading sets (Fig. 3). The first set of papers focus on incorporating geographic data with phylogenetic hypotheses. Lawing (Reference Lawing2021) begins the special issue with a synthesis and review of the utility of PaleoPhyloGeographic species distribution models in understanding how species and communities respond to climate change. Lam et al. (Reference Lam, Sheffield and Matzke2021) then present a study of the biogeography of diploporan blastozoan echinoderms across the great Ordovician biodiversification event, using the phylogeny of the clade to infer ancestral areas of occupation and showing that diploporan biogeography evolved through episodes of dispersal throughout the Ordovician, with dispersal events driven by changes in oceanic and epicontinental currents. Next, Bauer (Reference Bauer2021) examines the macroevolutionary trends of eublastoid echinoderms within a phylogenetically informed biogeographic framework, showing that, once again, dispersal events predominate throughout their evolutionary history. Finally, Friend et al. (Reference Friend, Anderson and Allmon2021) complete the paper set by bridging the topics of geography and hierarchical sorting, reexamining a classic macroevolutionary study in volutid gastropods and showing that, when placed in a phylogenetic and geographic context, shifts to non-planktotrophy within the group were the result of geographic contingency rather than species sorting.

Figure 3. Data flow diagrams for studies within each set of papers in this special issue, demonstrating how the papers combine different types of data within phylogenetic paleoecology. Each source of data or analysis is color coded to the relevant region of Fig. 2. Fossil and extant species represent the source from which all data are derived, and as such they are represented as the central overlap between all four data types. A, Data flow diagram for Bauer (Reference Bauer2021), who combines biogeographic, phylogenetic, and ecological data of extinct blastoid echinoderms to analyze how these factors shaped the clade's evolutionary history. B, Data flow diagram for Falk et al. (Reference Falk, Lamsdell and Gong2021), who combine ecological data from extinct and extant bird species with a phylogenetic framework and morphological data from avian hindlimbs to explore the relative impacts of ecology and phylogenetic history on morphology. C, Data flow diagram for Lamsdell (Reference Lamsdell2021), who incorporates ontogenetic data from extant and extinct horseshoe crab species with ecological data within a phylogenetic framework to determine the influence of ecological shifts and importance of evolutionary history in mediating mode of evolution. (In color online.)
The second set of papers broadly focus on the complex relationship between the evolutionary and ecological hierarchies (sensu Congreve et al. Reference Congreve, Falk and Lamsdell2018) as expressed by patterns of ecological conservatism and convergence and their impact on survivorship. Cole (Reference Cole2021) examines the evolutionary history of diplobathrid crinoids, utilizing a genus-level phylogeny to determine controls of extinction selectivity as indicated by genus longevity. An analysis of the combined effects of species richness, habitat preference, body size, filtration fan density, and food size selectivity shows that ecology has an important but complex role in determining crinoid genus duration, with traits exhibiting varying degrees of phylogenetic signal. Hughes and Lamsdell (Reference Hughes and Lamsdell2021) explore the phylogenetic distribution of prey size in stylonurine eurypterids and the role that prey specialization may have had on the survivorship of certain eurypterid groups during the Late Devonian biotic crisis, determining that prey availability may have mediated their transition to freshwater environments but that survivorship was most likely influenced by other traits, such as geographic range. Sclafani et al. (Reference Sclafani, Congreve and Patzkowsky2021) tackle the relationship between evolutionary history and ecology directly by comparing metrics for ecological distance and phylogenetic distance between strophomenid brachiopods. Ecological distance and phylogenetic distance are shown not to correlate, reinforcing the conflicting relationship between the two biological hierarchies. Chang and Skipwith (Reference Chang and Skipwith2021) take a community approach to studying ecological and phylogenetic relatedness through the lens of competitive exclusion. A dataset of bivalve communities from the Cenozoic of the San Joaquin Basin reveals that neither competitive exclusion nor environmental filtering have predominantly influenced community composition within the basin over the last 27 Myr. To conclude the set, Falk et al. (Reference Falk, Lamsdell and Gong2021) link to the final pair of papers in exploring patterns of morphological convergence in relation to the phylogenetic distribution of ecological life habit. Potential links between phylogeny, ecology, and morphology are analyzed through a morphometric analysis of avian hindlimb measurements, suggesting that while changes in morphospace occupation are visible through time as avians shift to a modern hindlimb structure, the interactions between phylogeny and ecology are largely complex.
The final set comprises two papers that incorporate aspects of evolutionary developmental biology (Raff Reference Raff2000; Hall Reference Hall2003; Müller Reference Müller2007; de Robertis Reference De Robertis and M2008) and its extension, ecological evolutionary developmental biology (Gilbert et al. Reference Gilbert, Bosch and Ledón-Rettig2015), to determine the underlying biological processes leading to morphological change and the role of ecological pressure as a mediating factor. Pietsch et al. (Reference Pietsch, Anderson, Maistros, Padalino and Allmon2021) study the evolution of the extreme parietal sinus of gastropods, using shell microstructure and growth patterns to show that the convergent evolution of this feature within some groups may be due to parallelism. Finally, Lamsdell (Reference Lamsdell2021) presents a novel method for quantifying heterochronic trends within evolutionary lineages and applies it to xiphosuran arthropods, exploring patterns of paedomorphosis and peramorphosis in relation to shifts in ecological occupation. The data reveal convergent patterns of heterochrony in response to occupation of nonmarine environments but divergent responses in the type of heterochronic change, once again demonstrating the complex nature of the relationship between genealogical and ecological hierarchies.
The collection of papers included in this special issue addresses important macroevolutionary and macroecological phenomena through leveraging phylogenetic and ecological data with the aim of determining how fundamental evolutionary processes mediate taxonomic, ecological, and morphological diversity. These papers demonstrate not only the current state of the art of research within phylogenetic paleoecology, but also the potential for the discipline to tackle wide-ranging questions critical to understanding the evolution of both ancient and modern clades and ecosystems. We hope this special issue will encourage researchers to continue developing the methods presented here and to apply them to new taxonomic groups, geological events, and evolutionary questions.
Acknowledgments
The guest editors first and foremost thank the authors for their valuable contributions to this special issue. Together, they have produced a compilation of exciting, cutting-edge research that showcases the full potential of the field of phylogenetic paleoecology. We also express our gratitude to M. Patzkowsky for his encouragement and support in proposing this special issue and to the entire Paleobiology editorial board for accepting our proposal. We are also grateful to all the referees who provided insightful reviews of the submitted manuscripts. This special issue would not have been possible without the tireless work of J. Kastigar, who ensured everything functioned smoothly behind the scenes. J.C.L. appreciates the advice of B. Anderson, E. Hughes, N. Machida, and S. Ocon on earlier drafts of the figures. Finally, we also thank our colleagues who helped develop and formalize the concept of phylogenetic paleoecology as a distinct field, particularly M. Hopkins, M. Patzkowsky, and A. Z. Krug, our coauthors on the original phylogenetic paleoecology paper. J.C.L. is supported by National Science Foundation CAREER award EAR-1943082 “Exploring Environmental Drivers of Morphological Change through Phylogenetic Paleoecology.”