Our food supply has become largely processed and higher in sugars than it was in previous generations. A significant amount of research has examined the association between excessive sugar consumption and the risk of obesity and an array of other health issues. This has included consideration of both regular dietary sugars (sucrose, fructose, etc.) and alternative sweeteners, encompassing artificial sweeteners (aspartame, sucralose, etc.) and natural low or zero energy sweeteners (stevia, sugar alcohols, etc.), that are abundant in the food supply. However, the concept of sugars and alternative sweeteners as environmental exposures that can have secondhand effects on the developing infant has not been widely explored.
Secondhand sugars include regular dietary sugars and alternative sweeteners in the food supply that individuals do not actively choose to consume but are inadvertently exposed to. This concept is similar to that of secondhand tobacco smoke, where non-smokers can be exposed to and harmed by the smoke produced by those around them. Exposure to secondhand sugars can occur during various life stages. Even before birth, fetuses can be exposed to sugars and sweeteners that their mothers consume via the placenta. There is also evidence that maternal and paternal diet prior to conception can epigenetically affect the germline, and influence offspring growth and development. After birth, babies are exposed to sugars through breast milk and/or in infant formula and other products. As growing children and adolescents are usually not responsible for their own food choices, instead of relying on adults to prepare their meals, they are often also exposed to secondhand sugars.
Arguably the most vulnerable population to consider when it comes to secondhand sugar exposure is fetuses. Fetuses are completely reliant on nutrients supplied by their mothers for healthy growth and development. Exposure to harmful substances during these important phases can derail healthy development and potentially lead to irreversible future health problems. The purpose of this review is to summarise the existing literature related to how prenatal exposure to secondhand sugars and alternative sweeteners impact child development and health. We will also discuss how high sugar consumption in pregnancy impacts maternal health, as these effects, such as excessive weight gain, can also cause pregnancy complications that impact the fetus. Finally, we will highlight specific gaps in the research in this arena that we believe should be priorities for future work.
Sugar consumption during pregnancy and maternal weight gain
While an adequate amount of maternal weight gain in pregnancy is important for ensuring the healthy growth and development of the fetus, it is estimated that nearly 50 % of women gain more weight than is recommended during pregnancy(Reference Goldstein, Abell and Ranasinha1). As we will discuss later, excessive gestational weight gain can contribute to various health issues for both mother and fetus. There is no clear consensus in the literature about which dietary factors contribute the most to excessive weight gain during pregnancy. Review papers that cover this topic(Reference Tielemans, Garcia and Santos2–Reference Streuling, Beyerlein and Rosenfeld4) conclude that excess energy intake is a risk factor, but that the evidence for other macronutrients and food groups has been mixed.
Although few studies have specifically evaluated the effects of excessive sugar consumption on gestational weight gain, there is emerging evidence suggesting a positive association. To our knowledge, the largest study to evaluate this relationship was a prospective cohort study of 46 262 women in Denmark(Reference Maslova, Halldorsson and Astrup5). The authors found that consumption of added sugars during pregnancy was strongly and positively associated with excessive gestational weight gain. In contrast, a higher protein to carbohydrate ratio was inversely associated with weight gain(Reference Maslova, Halldorsson and Astrup5). Furthermore, through statistical modelling, they found that replacing carbohydrates with protein reduced weight gain, and this was driven specifically by a reduction in added sugars. Translating their results, the authors estimated that women in the highest v. lowest quintiles of added sugar intake (89 (sd 26) v. 19 (sd 5) g/d) gain an extra 1·4 kg weight during pregnancy on average. In secondary analyses, they found that the intake of sweets (primarily chocolate and mixed candy) was directly associated with gestational weight gain, but data for other foods or beverages was not presented.
There have also been observational studies conducted in other countries including Germany, South Africa, the Netherlands, the USA and Iceland. These studies found similar associations between sugar consumption in pregnancy and excess maternal weight gain, either through examination of single nutrients(Reference Diemert, Lezius and Pagenkemper6), food groups(Reference Olafsdottir, Skuladottir and Thorsdottir7), or dietary patterns that include a high intake of added sugars(Reference Wrottesley, Pisa and Norris8–Reference Starling, Sauder and Kaar10). Observational studies evaluating the effects of alternative sweeteners on weight gain in pregnancy have been more limited. One study showed that consuming diet beverages in pregnancy increases the risk for excess pregnancy weight gain(Reference Renault, Carlsen and Norgaard11), which is consistent with findings from the general population(Reference Swithers12).
There have also been a few intervention studies that reported on changes in sugar intake and their relationship with gestational weight gain(Reference Renault, Carlsen and Norgaard11, Reference Poston, Bell and Croker13, Reference Petrella, Malavolti and Bertarini14), although not all were designed specifically to reduce sugar intake. For example, in a study of 342 obese women in Denmark, participants were randomised to either a control group, a physical activity intervention group, or a physical activity plus dietary intervention group(Reference Renault, Carlsen and Norgaard11). The physical activity plus dietary intervention group received instruction from trained dietitians on how to adhere to a hypoenergetic diet that was low in saturated fat and Mediterranean in style and were advised to aim for 11 000 steps daily (measured by a pedometer). No details were published regarding if women were given specific guidance on dietary sugars. Compared with the controls, the diet plus physical activity group had significant increases in the intake of protein and PUFA, as well as decreases in intake of added sugars and saturated fat. The diet plus physical activity intervention group also showed a lower relative risk of excessive weight gain as compared with the controls (0·73 (95 % CI 0·57, 0·94)), while the physical activity intervention group alone showed no difference in gestational weight gain compared with controls. The authors also conducted a pooled analysis evaluating potential associations between baseline dietary variables and change in gestational weight gain, regardless of randomisation group. They found that the intake of added sugars in foods was the only dietary variable associated with gestational weight gain. In an analysis of specific food groups, baseline intake of sweets, snacks and cakes was associated with an increased risk of excessive weight gain (>9 kg). Women consuming two or more servings of sweets daily (such as chocolates, caramels, licorice, gummy and jelly sweets) gained an additional 5·5 kg weight compared with women who consumed them less than once weekly. The results for soft drinks were more mixed: intake of sugar-sweetened sodas was inversely associated with weight gain at baseline but was not associated with weight gain at the endpoint measurement (36–37 weeks gestation), whereas intake of diet sodas was positively associated with weight gain only for consumption levels at the endpoint measurement. The authors concluded that reducing intakes of these (sugary) foods may be more relevant for limiting gestational weight gain than encouraging strict compliance to more specific diets.
Maternal sugar consumption and common pregnancy complications
There is also evidence to suggest that, independent of gestational weight gain and/or obesity, excessive sugar consumption during pregnancy is associated with pregnancy complications. Of the various types of sugar, fructose is thought to be particularly damaging(Reference Regnault, Gentili and Sarr15). One study that investigated the effects of maternal carbohydrate and sugar consumption and their relation to gallbladder disease in 3070 pregnant women reported that fructose was associated with adverse outcomes, whereas other types of sugars were not(Reference Wong and Ko16). These results held strong even after controlling for overall carbohydrate intake and weight-related variables. Animal studies have also shown that increased fructose intake during pregnancy increases the risk of maternal fatty liver disease(Reference Zou, Arentson and Teegarden17–Reference Clayton, Vickers and Bernal19), insulin resistance/glucose intolerance(Reference Zou, Arentson and Teegarden17, Reference Shortliffe, Hammam and Han18, Reference Alzamendi, Del Zotto and Castrogiovanni20), as well as hypertension(Reference Shortliffe, Hammam and Han18) and/or preeclampsia(Reference Alzamendi, Del Zotto and Castrogiovanni20). While the majority of studies conducted in human subjects have not evaluated the specific effects of fructose, the literature suggests that an overall increased consumption of sugars is associated with increased risk for common pregnancy complications, including gestational diabetes, preeclampsia and premature delivery, all of which we will discuss later.
Gestational diabetes
A high intake of added sugars during pregnancy is one of the dietary variables that has been shown to be related to the development of gestational diabetes mellitus (GDM). A study that used National Health and Nutrition Examination Survey data to look at dietary patterns of pregnant women and risk for gestational diabetes found that a diet characterised by high added sugar and organ meats; low fruits, vegetables and seafood was the pattern with the highest risk for GDM(Reference Shin, Lee and Song21). The authors concluded that this risk was largely explained by the high consumption of added sugars and low consumption of fruit and vegetables. Another article using data from the Nurses’ Health Study II reported that intake of sugar-sweetened beverages before pregnancy was associated with the development of GDM(Reference Chen, Hu and Yeung22). More specifically, consuming >5 sugar-sweetened cola drinks per week increased the risk of gestational diabetes by 22 %. Note that this study did not include an examination of juices in the analysis, and the findings were very specific to sugar-sweetened cola drinks in particular. Another study also examined the relationship between pre-pregnancy sugar-sweetened soft drink consumption and future risk for GDM and found a positive association which persisted even after controlling for potential confounders including family history of diabetes, BMI, total energy intake and fibre intake(Reference Donazar-Ezcurra, Lopez-Del Burgo and Martinez-Gonzalez23).
Preeclampsia
While research is limited, there is some evidence to suggest that drinking sugar-sweetened beverages in pregnancy can increase a woman's risk of preeclampsia. A large study conducted in Norway that examined the diets of over 32 000 pregnant women showed that increased consumption of sugar-sweetened carbonated and noncarbonated drinks was associated with a higher risk for preeclampsia, after adjustment for total energy intake and other confounding variables (OR for the combined beverages 1·27 (95 % CI 1·05, 1·54) for high intake (>125 ml/d) compared with no intake)(Reference Borgen, Aamodt and Harsem24). In contrast, eating fresh or dried fruit was associated with a lower risk, perhaps due to their fibre content. Interestingly, the authors found that while the positive association between sugar-sweetened beverage consumption and preeclampsia was significant in both normal and overweight women, the risk was higher in women with a BMI <25 as compared with 25 or above (crude OR 1·32 v. 1·28). A smaller Norwegian study (n 3133) also showed a positive relationship between high sucrose consumption (>25 % of total energy) and preeclampsia(Reference Clausen, Slott and Solvoll25). While potential relationships with other types of sugars were not presented, the authors did evaluate specific food items and found that consumption of sugar-sweetened soft drinks was related to increased risk.
Premature delivery
While many factors can contribute to risk for premature delivery, and often times the cause for its occurrence is unknown, maintaining a healthy diet in pregnancy can serve as an additional safeguard(Reference Abu-Saad and Fraser26). There is specific evidence to suggest that increased intake of sweetened beverages is related to risk for premature delivery. For example, Englund-Ogge et al. examined the relationship between sweetened beverage consumption in pregnancy and preterm delivery in 60 000 women in Norway(Reference Englund-Ögge, Brantsæter and Haugen27). They found that one daily serving of sugar-sweetened beverages increased the risk of preterm delivery by 25 %. In addition, daily one serving of diet beverages increased the risk by 11 %. In a study of the same size from Denmark, researchers found that just one daily serving of diet beverage consumption increased the risk of a preterm delivery by 38 %, and four daily servings increased the risk by 78 %(Reference Halldorsson, Strøm and Petersen28). Interestingly, in this study, which used slightly different methods, there was no effect of regular sugar-sweetened beverages on preterm delivery. In contrast, a smaller study from the UK showed that consumption of sugar-sweetened cola beverages did increase the risk of preterm delivery, but did not show a relationship with artificial sweeteners(Reference Petherick, Goran and Wright29).
Possible mechanisms that could explain the observed effects of sugar-sweetened beverages on the pregnancy complications presented here include pathways related to insulin sensitivity and inflammation. Normal pregnancy is characterised by reduced maternal insulin sensitivity in peripheral tissues, but this is more pronounced in overweight individuals or those with gestational diabetes(Reference Catalano, Huston and Amini30). These effects can be exacerbated by a high sugar diet. Decreased insulin sensitivity can lead to glucose intolerance, which is a known risk factor for preeclampsia and preterm delivery and the defining feature of GDM(Reference Lao and Ho31–33). Additionally, increased sugar consumption is associated with increased circulating pro-inflammatory cytokine concentrations, particularly in individuals with impaired glucose tolerance(Reference Esposito, Nappo and Marfella34). Increased circulating pro-inflammatory cytokine concentrations, in turn, are associated with GDM, preeclampsia and preterm delivery(Reference Wenstrom, Andrews and Hauth35). Interestingly, in the study discussed in the previous paragraph, Englund-Ogge et al.(Reference Englund-Ögge, Brantsæter and Haugen27) found that the consumption of sugar-sweetened beverages was more highly correlated with early preterm delivery, while consumption of artificially sweetened beverages was correlated with late preterm delivery. The authors hypothesised that this could be due to the fact that younger fetuses are more sensitive to inflammatory interleukins.
In terms of alternative sweeteners, the exact mechanism of how they may contribute to preterm delivery is not known. One possible explanation is the way these compounds are broken down by the body. Aspartame, for example, is metabolised into aspartic acid, phenylalanine and methanol. The methanol is then converted into formaldehyde and formic acid, which is toxic in high doses. In animal studies, exposure to even a low dose of methanol can result in pregnancy complications including preterm delivery(Reference Burbacher, Grant and Shen36). Another possible explanation relates to how these alternative sweeteners affect the gut microbiome. Animal studies have demonstrated that artificial sweeteners disrupt the normal/healthy composition of bacteria that reside in the gut(Reference Suez, Korem and Zilberman-Schapira37). Alteration of gut bacteria can increase numbers of unhealthy bacteria that promote inflammation and increased gut permeability and reduce numbers of healthy bacteria that assist in fermentation and satiety hormone production(Reference Taylor, Woodfall and Sheedy38, Reference Tilg and Moschen39). However, it must be noted that this evidence is reliant on animal studies and its applicability to human biology remains unknown.
Effects of sugar consumption before pregnancy on fetal development and offspring health
While the majority of this review focuses on the impact of maternal diet during pregnancy on maternal and offspring outcomes, there is mounting evidence for a role of diet prior to conception(Reference Nicholas, Rattanatray and MacLaughlin40). Animal studies have demonstrated that overfeeding prior to pregnancy (and not during pregnancy) can have long-lasting programming effects on offspring(Reference Shankar, Harrell and Liu41). For example, sheep embryos from dams overfed in the periconceptional period and transferred to the wombs of non-obese dams show increased fat mass at 4 months(Reference Rattanatray, MacLaughlin and Kleemann42). While it is more difficult to separate the effects of preconception and prenatal nutrition in human subjects, some researchers have developed methods of doing so. Dominguez-Salas et al. used seasonal differences in nutrition in Gambian women to pinpoint the influence of the preconception period. They reported that maternal nutrition around the time of conception influenced DNA methylation in lymphocytes and hair follicles from infants postnatally(Reference Dominguez-Salas, Moore and Baker43) Another study found that maternal lipid profile at conception successfully predicted preterm birth, regardless of whether the women were provided essential fatty acid supplementation during pregnancy(Reference Ogundipe, Johnson and Wang44). Indeed, it may be the case that by the time sugar-related pregnancy complications are realised, the optimal window for intervention has passed. Therefore, pre-pregnancy sugar intake may be just as or more influential than intake during pregnancy.
Importantly, the relevance of preconception nutrition does not appear to be limited to mothers. There are now a series of animal studies to show that poor diet quality in fathers can be transmitted to offspring through the germ-line. Carone et al., demonstrated that a high sucrose, low protein diet consumed by male mice affects the expression of key metabolic genes in offspring, including upregulation of cholesterol biosynthesis genes. The mechanisms underlying this appear to be epigenetic, as paternal diet was highly correlated with cytosine methylation of the enhancer of lipid transcription factor Ppara (Reference Carone, Fauquier and Habib45). However, direct examination of sperm methylation patterns did not show an effect of diet, suggesting alternative epigenetic information carriers such as RNA. Indeed, a subsequent study reported that direct injection of naive one-cell embryos with sperm or testis RNA from high sugar/high fat-fed donors induced obesity and metabolic dysfunction in the resulting progeny(Reference Grandjean, Fourré and De Abreu46). To the best of our knowledge, there do not yet exist studies examining the impact of preconception maternal and paternal alternative sweetener intake on offspring health. However, these existing studies suggest that both maternal and paternal preconception diet are important contributors to the health of offspring, and if possible, nutritional interventions should not be limited to pregnancy alone.
Effects of sugar consumption in pregnancy on fetal development and offspring health
Transfer of regular sugars across the placenta and effects on fetal development
While studies conducted in human subjects are limited due to ethical issues, it is well established that both glucose and fructose cross the human placenta(Reference Holmberg, Kaplan and Karvonen47), and therefore reach and can affect the developing baby. Human studies also suggest that fructose concentrations are higher in the fetal bloodstream relative to that of the mother, suggesting active transport of fructose across the placenta(Reference Hagerman and Villee48) and animal studies have confirmed this(Reference Regnault, Gentili and Sarr15). For example, one study in rats showed that gestational exposure to fructose led to pups who were hyperglycemic at birth(Reference Jen, Rochon and Zhong49).
The developing fetus lacks the ability to undergo gluconeogenesis, and thus relies on the transport of glucose from the maternal bloodstream(Reference Bibee, Illsley and Moley50). One key player in the placental transport of both glucose and fructose is facilitative GLUT-9, which consists of the isoforms GLUT-9a and 9b(Reference Doblado and Moley51). GLUT-9 is unique in that it can also transport fructose and uric acid. The protein expression of GLUT-9 is directly associated with blood glucose concentrations; hyperglycemia increases expression and hypoglycemia decreases expression(Reference Doblado and Moley51). Thus, insulin-resistant and diabetic individuals will tend to have increased expression of GLUT-9. This increased expression has also been observed in the placental tissue of pregnant diabetic women(Reference Bibee, Illsley and Moley50). This has been suggested as playing a key role in fetal pathologies frequently seen in diabetic pregnancies, likely due to increased placental and fetal exposure to glucose and fructose(Reference Bibee, Illsley and Moley50).
The exact effects of exposure to increased fructose levels on placental and embryonic tissue are not fully understood. Rodriquez et al. demonstrated that fetuses from fructose-fed pregnant rats had hypertriglyceridemia and higher hepatic TAG content(Reference Rodríguez, Panadero and Roglans52). These fetuses also had higher expression of genes related to lipogenesis and a low expression of genes related to fatty acid catabolism. Additionally, it was noted that these fetuses had an impairment in the leptin signalling pathway. Vickers et al. similarly demonstrated markers of impaired metabolic function including hyperglycemia and hyperleptinemia in fetuses and neonates of fructose-fed mothers, particularly in female offspring(Reference Vickers, Clayton and Yap53). Together, these effects could predispose pups to obesity early in life. Further studies are necessary to determine if similar outcomes hold true in human subjects. Additionally, it has been demonstrated that elevated fructose levels are associated with increased levels of reactive oxygen species, In fact, it has been suggested that reactive oxygen species-related oxidative damage could be a ‘unifying mechanism’ to explain diabetic complications(Reference Brownlee54).
Maternal transfer of alternative sugars and their by-products to the fetus
It is known that saccharin crosses the placenta(Reference Cohen-Addad, Chatterjee and Bekersky55), as do the breakdown products of aspartame(Reference Sturtevant56), and it is hypothesised that sucralose and acesulfame-K cross the placenta as well(Reference Rodero, Batig á lia and Azoubel57, Reference Zhang, Chen and Liu58). While animal studies have not found these products to have toxic effects on the fetus(Reference Pope, Koren and Bozzo59), research has been limited and results from animal studies must be interpreted with caution due to differences in physiology as compared with human subjects. Therefore, the complete effects of these products in human subjects are not known. It has been previously concluded that moderate consumption of artificial sweeteners is safe during pregnancy(Reference Pope, Koren and Bozzo59). However, given the observational research we describe in this review that suggests negative effects of maternal consumption on outcomes such as premature birth(Reference Englund-Ögge, Brantsæter and Haugen27, Reference Halldorsson, Strøm and Petersen28) and future offspring adiposity(Reference Zhu, Olsen and Mendola60, Reference Azad, Sharma and de Souza61), it is possible that there are mechanistic links and risks that are not yet fully understood. Studies on the effects of other alternative sweeteners such as stevia and sugar alcohols are also lacking.
Effects of maternal sugar and alternative sweetener intake on future childhood obesity
Regular sugars
A variety of studies conducted in human subjects have shown that excess maternal sugar consumption during pregnancy can increase the chances that a child will become overweight or obese(Reference Phelan, Hart and Phipps62–Reference Gillman, Rifas-Shiman and Fernandez-Barres65). For example, a study conducted in Singapore with 910 mother/child pairs found that higher sugar and carbohydrate intakes during late pregnancy were associated with the higher BMI in the children at ages 2–4 years, whereas fat and protein intake were not related to these markers(Reference Chen, Aris and Bernard63). Another study, conducted in the Netherlands, evaluated 3312 mother–child pairs and found that daily one additional serving of a sugar-sweetened beverage during pregnancy resulted in a 0.05 standard deviation score higher fat mass index in the child at age 6 years. Furthermore, when types of drinks were evaluated separately, intakes of 100 % fruit juice, but not of soda or sweetened juice concentrate drinks, were associated with a higher child fat mass. These findings were independent of gestational weight gain, birth weight, and children's insulin concentrations. Similarly, an American study conducted with 1078 mother–child pairs found that maternal sugar-sweetened beverage consumption was related to future childhood obesity. Specifically, each serving of sugar-sweetened beverage consumed daily during the second trimester was related to measures of child overweight at age 7 years, including BMI, fat mass index and waist circumference(Reference Gillman, Rifas-Shiman and Fernandez-Barres65). Finally, a smaller study in the USA of 285 mothers/children found that in overweight or obese mothers, consumption of sweets was a predictor of birthweight, such that each 1 % increase in percentage of energy consumed from sweets early in pregnancy increased the odds of macrosomia by 10 % and weight for age >90th percentile by 20 %(Reference Phelan, Hart and Phipps62). Also, in the overweight or obese group, at 6 months, the strongest predictors of higher weight for age z-scores were a greater percentage of energy from sweets early in pregnancy. In normal-weight women, higher intake of sugary drinks was the strongest predictor of birth weight but was not related to infant weight at 6 months.
In addition to dietary sugar content, the overall glycemic load of the maternal diet during pregnancy has been linked to increased risk of offspring obesity. A study conducted in the UK with 906 mother–child pairs found that both maternal dietary glycemic index and glycemic load in early pregnancy (11 weeks) were positively associated with child fat mass at age 4 and 6 years, whereas maternal glycemic index during late pregnancy (34 weeks) was not(Reference Okubo, Crozier and Harvey66). Another study that evaluated the effects of the maternal glycemic index on neonatal adiposity in 542 mother–child pairs in the UK found that maternal glycemic load in the second trimester was associated with neonatal central adiposity as measured by waist to length ratio(Reference Horan, McGowan and Gibney67). Additional studies are required to help clarify the role of the maternal glycemic index on parameters of offspring obesity and in particular which trimester of pregnancy is the most influential.
Alternative sweeteners
The effects of maternal consumption of alternative sweeteners during pregnancy on infant and child outcomes have not been extensively studied. One detailed study in 3033 mother–infant dyads in Canada showed that 30 % of women consumed artificial sweeteners during pregnancy(Reference Azad, Sharma and de Souza61). Women who consumed artificial sweeteners daily had a 2-fold higher risk of an infant being overweight by age 1 year. Another study conducted in Denmark with 918 mother–child dyads found that approximately half of the mothers consumed artificially sweetened beverages and 9 % consumed them on a daily basis. Compared with those participants who never consumed artificial sweeteners, the daily consumers were more likely to have children who were large for gestational age at birth (relative risk 1·57; 95 % CI 1·05, 2·35) and who were overweight or obese by age 7 years (relative risk 1·93; 95 % CI 1·24, 3·01). Furthermore, they found that substituting artificially sweetened beverages with water reduced risk for overweight at age 7 years, but substituting regular sweetened beverages with artificially sweetened alternatives did not confer a reduced risk.
Effects of maternal sugar consumption on future child feeding behaviours and metabolism
One of the ways that exposure to excess sugars during gestation can promote future obesity is through fetal programming(Reference Ojha, Fainberg and Sebert68). While the brain, vital organs and adipose tissue are developing in utero, they are particularly vulnerable to nutritional insults. As we will explain later, a maternal diet that is high in sugar may lead to structural and functional alterations that can predispose a child to poor feeding behaviours and a metabolism that favours fat storage.
During early gestation, the structures and pathways of the brain that are responsible for feeding behaviour are already developing. The hypothalamus, which begins to develop as early as 9 weeks gestation, plays a key role in energy and glucose homeostasis and disruption of its healthy development is thought to create a predisposition for metabolic diseases(Reference Dearden and Ozanne69). Although research in human subjects has been limited, studies in animals suggest that a maternal diet that is high in sugar is associated with altered gene expression, hyperphagia and disrupted glucose homeostasis in the offspring(Reference Dearden and Ozanne69). The central reward system is also important in determining feeding behaviour. Studies in human subjects, while limited, suggest that dopamine and opioids are expressed in the fetal striatum by 12 weeks gestation and their associated receptors can be detected by about 20 weeks gestation(Reference Gugusheff, Ong and Muhlhausler70). A maternal diet that is high in sugars stimulates the synthesis and release of these compounds that are related to pleasure and reward and can predispose a child to prefer sweet tastes and even to become addicted to them(Reference Gugusheff, Ong and Muhlhausler70).
While much of the evidence for the association between a high sugar diet and alterations in feeding behaviours and metabolism come from animal studies, there have been observational studies conducted in human subjects that support the same conclusions. For example, Brion et al. evaluated maternal dietary data both during pregnancy and at 47 weeks postpartum, paternal dietary data at 47 weeks and child dietary data at age 10 years(Reference Brion, Ness and Rogers71). They found that maternal gestational intakes of protein, fat and carbohydrates were positively associated with the child's intakes at age 10 years, whereas paternal diet was not strongly associated. Furthermore, for protein and fat intake, maternal prenatal diet was more strongly associated with the child's future intakes than were the mother's postnatal intakes. These results suggest a programming effect and that the in utero food environmental may have a larger influence on the child's diet than the eventual family food environment. There has also been research to suggest that a child's taste preferences are shaped in utero by the maternal diet(Reference Ventura and Worobey72). In a classic study, it was shown that when infants were tested before weaning, those infants who were exposed to carrot flavour via maternal consumption in pregnancy exhibited more liking for carrot flavour compared with those infants who were not exposed in utero (Reference Mennella, Jagnow and Beauchamp73). While to our knowledge, a similar study has not been conducted with a maternal exposure to sugar, we do know that a preference for sweet tastes is present at birth and probably before(Reference Ventura and Mennella74). For example, a study in preterm infants found they sucked stronger and more frequently on a nipple that had been soaked in sucrose compared with one that had not(Reference Maone, Mattes and Bernbaum75). One potential reason for this is that an innate desire for sweetness is advantageous in evolutionary terms.
Of the various types of sugars, fructose, in particular, should be considered as a prenatal exposure that has the potential for negative programming effects on offspring metabolism(Reference Goran, Dumke and Bouret76). There is only limited data available on the long-term effects of high fructose exposure during gestation. However, there are some studies, mostly in animals that suggest that high fructose consumption in pregnancy can lead to persistent neuroendocrine and metabolic alterations in the baby related to the development of feeding behaviour and propensity to develop obesity later in life(Reference Regnault, Gentili and Sarr15, Reference Goran, Dumke and Bouret76). Several potential mechanisms could explain the adverse effects of high fructose exposure during these periods. For example, we know that even very low levels of fructose can directly promote the process of building new fat cells during critical periods of development(Reference Du and Heaney77). High fructose intake during development might also promote obesity by disrupting the normal signalling that occurs between the brain and adipose tissue(Reference Regnault, Gentili and Sarr15, Reference Rodríguez, Panadero and Roglans52, Reference Goran, Dumke and Bouret76, Reference Teff, Elliott and Tschöp78). Results from a well-controlled human study evaluated changes in appetite-related hormone concentrations over 24 h in response to meals containing either glucose or fructose. Levels of insulin and leptin were significantly lower after the fructose meal than after the glucose meal, and fructose failed to suppress post-meal ghrelin levels as effectively as glucose, which suggests that consumption of fructose could disrupt energy balance signalling to the brain and result in excess energy consumption and obesity(Reference Teff, Elliott and Tschöp78).
Alternative sugars are also important to consider in terms of their effects on fetal programming of feeding behaviours and future metabolic health(Reference Araújo, Martel and Keating79). In animals, prenatal exposure to artificial sweeteners leads to higher selection and taste preference for sweet foods in adulthood(Reference Zhang, Chen and Liu58, Reference Toigo, Huffell and Mota80). Beyond influencing behaviour, these exposures also can have metabolic consequences. Chronic exposure to aspartame in mice in utero and early life has also been shown to be associated with elevated fasting blood glucose as well as reduced insulin sensitivity in later life(Reference Collison, Makhoul and Zaidi81).
A summary diagram of the secondhand effects of sugars and alternative sweeteners is depicted in Fig. 1.
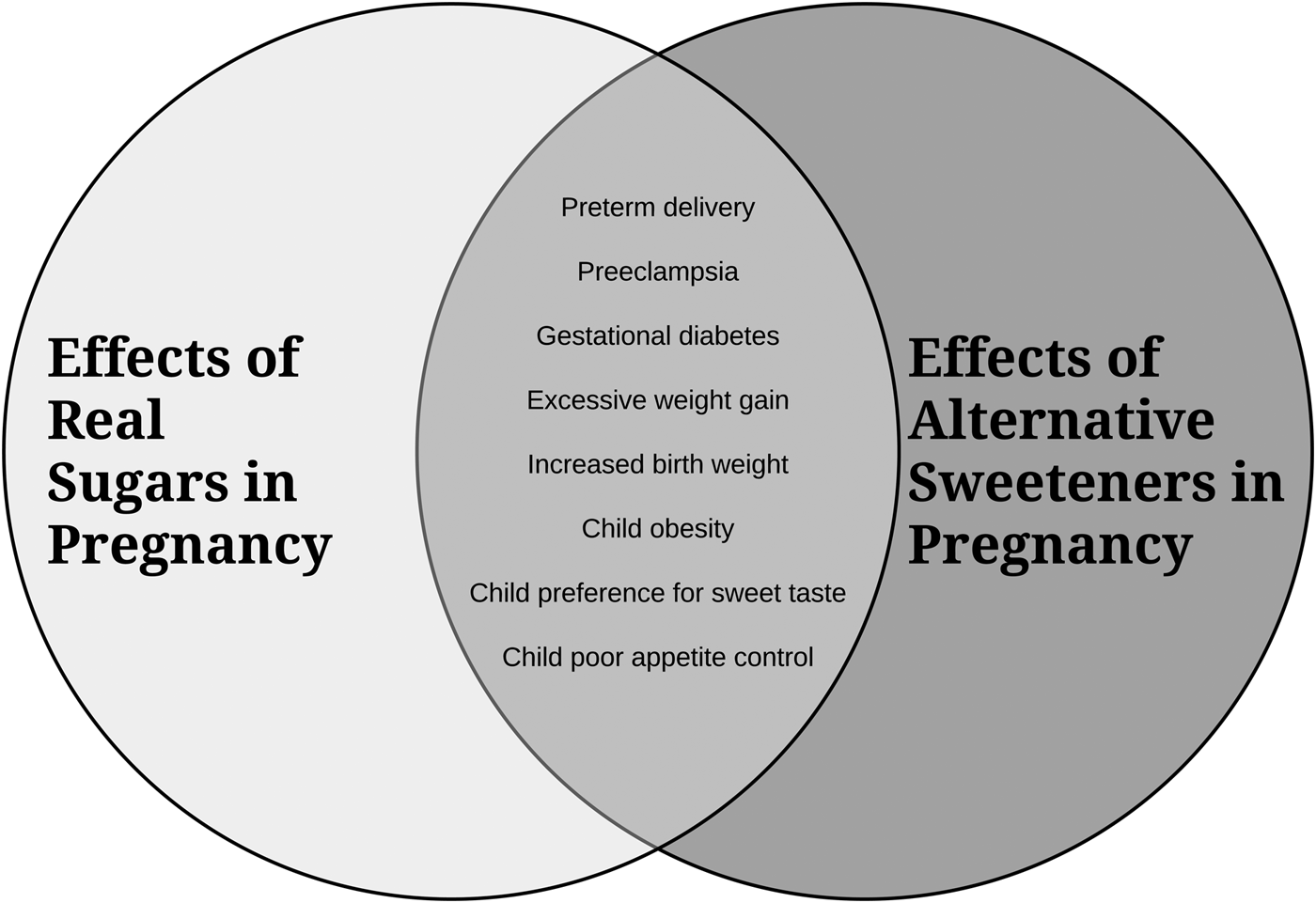
Fig. 1. An overview of how consumption of excess sugars and alternative sweeteners in pregnancy impacts maternal and infant/child health.
Future research priorities/needs
From our review of the literature, we suggest that future research is required in three categories to further our understanding of how exposure to sugars in pregnancy can affect maternal and child health: (1) further observational studies with more rigorous dietary assessment during pregnancy combined with detailed assessment of infant/child changes in body composition during growth; (2) additional mechanistic studies to understand exactly how gestational sugars impact fetal growth and development including effects on infant brain development, food intake regulation, taste preference and microbiome; and, (3) novel intervention studies that focus specifically on examining the effects of reduction of dietary sugars during pregnancy on future offspring behaviour, metabolism and health. In each of these categories, there is a need in particular for an additional focus on fructose and artificial sweeteners, both of which are hypothesised to have detrimental effects, as well as other alternative sweeteners, such as sugar alcohols or stevia, which have not yet been rigorously studied.
The first category relates to the increased need for observational studies that would help to further our understanding of the associations between consumption of various sugars in pregnancy and specific maternal and child health outcomes. For example, of the three studies that we are aware of that examined the relationship between sugar intake during pregnancy and risk for preterm delivery, results were mixed. One showed an effect of both regular and artificially sweetened beverages, while one found an association with just sugar-sweetened beverages and the other with just artificially sweetened beverages. Additional studies are required to confirm these findings and also to explore the effects of other specific types of sugars, sweeteners and categories of foods and beverages that contain them. Many of the studies conducted to date have not evaluated the effects of specific types of sugars, such as fructose, and/or specific alternative sweeteners. In addition, only a few studies have evaluated the effects of maternal glycemic index/load. Additional studies that evaluate these specific exposures using more precise dietary methods, as well as specific child outcomes measures such as detailed body composition and fat distribution outcomes, fatty liver disease and insulin resistance, would help to deepen our understanding. Finally, studies that include longer follow-up periods would help us to further understand the distal effects of secondhand sugar exposure on offspring health.
The second category of future research priorities pertains to studies that increase our understanding of the mechanisms that drive the observed associations between maternal sugar consumption in pregnancy and adverse child outcomes. Some of the mechanistic gaps in the literature are difficult to address because of the ethical and logistical challenges of conducting maternal–infant research. However, additional, targeted animal studies would be useful. As an example, we still only have a limited understanding of how exactly exposure to various types of sugars and sweeteners may influence the development of the hypothalamus and the wiring of the central reward system. We also do not know much about the specific effects of in utero exposure to some of the popular artificial sweeteners, such as sucralose, or popular non-energetic natural alternatives, such as stevia, on future offspring health.
The third category for future research is a need for additional intervention studies conducted with women who are either trying to conceive and/or already pregnant that focus specifically on reducing sugar intake. Programmes have been designed and tested to improve maternal diet in pregnancy and test their effects on maternal and child outcomes, but few have focused specifically or exclusively on sugar consumption. For example, a study conducted in the UK involved an intervention designed to reduce glycemic load, sugar-sweetened beverage consumption and saturated fat intake in obese pregnant women (n 1555) in order to reduce the incidence of gestational diabetes and large-for-gestational-age infants(Reference Poston, Bell and Croker13). While there were no significant effects on the primary study outcomes, the authors did report significant reductions in maternal glycemic index values and gestational weight gain. Future studies could be more targeted and focus solely on the reduction of sugar consumption in pregnancy. Once we have a better understanding of what types of programmes are effective, they can be translated for use in larger settings and serve as preventive measures for secondhand sugar exposures.
Limitations
Limitations of the available evidence discussed in this review include the potential for reverse causality, the fact that the food vehicles mostly (but not exclusively) studied were beverages, and the challenges of isolating effects of specific types of sugars and alternative sweeteners (e.g. fructose independently of glucose etc.). Further, the evidence presented is mostly (and understandably, for ethical reasons) observational, making it difficult to determine causality. Where available, prospective cohort studies usually relied on FFQ over the more rigorous 24-h dietary recall or provided meals, and sugars were not always considered separately from other dietary factors such as a high-fat diet. We must also acknowledge that it is difficult to assess sugar intake, especially considering the different forms available, (e.g. added, free, total sugars), which can also vary based on geographical location (for example, the USA often utilises high fructose maize syrup in beverages, while the equivalent beverage in other countries may comprise sucrose). There are also several forms of alternative sweeteners on the market, including artificial and natural sweeteners, which are used at different dosages and intensities, and may have differential effects in a secondhand sugar context. Quantifying alternative sweetener intake is also very difficult because the exact amounts used in products are not usually reported. Finally, we would like to acknowledge that it will be a challenge for nutrition science to disentangle sugar as a food ingredient, considering its use for functional properties such as mouthfeel, bulk, taste and food preservation in addition to its use as a nutrient. However, given the available evidence for detrimental effects of excessive sugar intake, including that of a secondhand sugar effect presented in the present paper, we believe the ongoing pervasiveness of sugar and alternative sweeteners in the food supply requires careful consideration.
Conclusion
In summary, although research has been limited, especially in human subjects, the available evidence suggests that there can be detrimental secondhand effects of in utero exposure to sugars from the maternal diet on offspring health. These exposures may have adverse consequences for both the mother and fetus, shape feeding behaviours and taste preferences in the offspring, and increase future risk for obesity and related metabolic diseases. As depicted in Fig. 1, the available research suggests that alternative sugars may be equally or more harmful than regular sugars. While further research including well-controlled prospective cohort trials is required, we suggest that sugars be viewed as an environmental health hazard for developing babies and that public health initiatives should be developed to help raise awareness and assist pregnant women to monitor and reduce their consumption of both regular and alternative sugars.
Financial Support
Dr Goran's research is supported by The Dr Robert C and Veronica Atkins Foundation, The Gerber Foundation and the National Institutes of Health (RO1 DK 110793; RO1 DK 109161; and RO1 MD 10358).
Conflict of Interest
None.
Authorship
M. G. and E. V. provided equal contribution to the authorship of this paper. J. P. reviewed, edited and wrote the fetal development/offspring health section of the paper.