Introduction
The metabolic syndrome is a global public health problem( Reference Nolan, Carrick-Ranson and Stinear 1 ). Approximately 25 % of the world’s population has the metabolic syndrome( Reference O’Neill and O’Driscoll 2 ) although the prevalence varies from < 10 to 84 % depending on the region, urban or rural environment, population demographics such as sex, age, race and ethnicity, and the definition of the syndrome used( Reference Kaur 3 ). Reduced HDL-cholesterol was the most prevalent component of the metabolic syndrome, followed by elevated blood pressure, abdominal obesity, high TAG and high fasting glucose( Reference Nolan, Carrick-Ranson and Stinear 1 ).
CVD are chief consequences of the metabolic syndrome( Reference Tune, Goodwill and Sassoon 4 ). Each component of the metabolic syndrome is an independent risk factor for CVD and the combination of these risk factors elevates the incidence and severity of CVD( Reference Tune, Goodwill and Sassoon 4 ). However, a wide spectrum of other morbidities occurs concurrent with or consequent on the metabolic syndrome. These include, but not limited to, type 2 diabetes, non-alcoholic fatty liver disease( Reference Lim and Bernstein 5 ), polycystic ovary syndrome( Reference Lim, Kakoly and Tan 6 ), several types of cancer( Reference Micucci, Valli and Matacchione 7 ), inflammatory bowel syndrome( Reference Michalak, Mosińska and Fichna 8 ) and chronic kidney disease( Reference Nashar and Egan 9 ). Accordingly, the metabolic syndrome is an important risk factor for all-cause mortality( Reference Wu, Liu and Ho 10 ). A meta-analysis of prospective cohort studies showed that individuals with the metabolic syndrome have a 46 % increased risk of mortality compared with individuals without the syndrome( Reference Wu, Liu and Ho 10 ).
Dietary modification and physical activity are suggested as the safest and most effective strategy for the prevention of the incidence or fundamental correction of the components of the metabolic syndrome( Reference Finicelli, Squillaro and Di Cristo 11 ). The best dietary modifications focus on correction of all items of the diet, what is called a dietary pattern. Instead of correcting individual nutrients or foods, dietary patterns suggest overall diet change which provides a firmer effect on the prevention of diseases. By definition, dietary patterns are the quantities, proportions, variety, or combination of different foods and drinks in diets, and the frequency with which they are habitually consumed( 12 ).
Dietary Approaches to Stop Hypertension (DASH), a dietary pattern which was initially proposed for the treatment of hypertension, is rich in fruits, vegetables and low-fat dairy products, and low in total and saturated fat and cholesterol( Reference Appel, Moore and Obarzanek 13 ). DASH has similarities with the Mediterranean diet, which is a dietary pattern with proved benefits against CVD( Reference Liyanage, Ninomiya and Wang 14 , Reference Salas-Salvadó, Becerra-Tomás and García-Gavilán 15 ). The cardioprotective effect of DASH has been examined less than the Mediterranean diet in epidemiological studies and randomised controlled trials (RCT). Due to emphasis on the consumption of whole grains and low-fat dairy products and caution in consuming red meats and salt, DASH may provide a better diet composition than the Mediterranean dietary pattern against metabolic diseases( Reference Schulze, Martínez-González and Fung 16 ).
In the present review, we discuss evidence on the effect of each dietary component of the DASH diet on risk factors of the metabolic syndrome. It is worth noting that consuming DASH has other profitable consequences relative to the metabolic syndrome and CVD that are not mentioned in this article. For instance, antioxidant vitamins and phytochemicals present in fruit and vegetables may reduce oxidative stress, improve antioxidant capacity and impede inflammatory responses, all of which are important in instigation or development of the atherosclerosis process( Reference Lopes, Martin and Nashar 17 , Reference Asemi, Samimi and Tabassi 18 ). Also, fruit and vegetables provide great quantities of folic acid, which is essential for optimising levels of homocysteine( Reference Craddick, Elmer and Obarzanek 19 ), a known risk factor associated with CVD and the metabolic syndrome( Reference Catena, Colussi and Nait 20 ).
Definition
The metabolic syndrome is a cluster of abnormalities including abdominal obesity, hypertriacylglycerolaemia, low HDL-cholesterol, high blood pressure and high fasting glucose( Reference Ricci, Pirillo and Tomassoni 21 ). During the years between 1998 and 2009, a number of definitions were proposed by different organisations based on various criteria and cut-off points( Reference McCracken, Monaghan and Sreenivasan 22 ). For instance, in earlier definitions proposed by the WHO, European Group for Study of Insulin Resistance, and American Association of Clinical Endocrinologists, insulin resistance or impaired glucose tolerance was suggested to be the fixed item in the diagnosis of the metabolic syndrome( Reference McCracken, Monaghan and Sreenivasan 22 ). In 2009, however, when the latest definition currently being used was proposed, the International Diabetes Federation, National Heart, Lung, and Blood Institute, American Heart Association, World Heart Federation, International Atherosclerosis Society and International Association for the Study of Obesity agreed on a definition, in which there is no compulsory component, but waist circumference may be considered as a useful screening tool( Reference Alberti, Eckel and Grundy 23 ). The harmonised criteria for clinical diagnosis of the metabolic syndrome are as follows: elevated waist girth according to population- and country-specific definitions, serum. The last four components will also be considered positive in the case of pharmacological treatment( Reference Alberti, Eckel and Grundy 23 ).
Pathophysiology
Abdominal obesity is supposed to play a pivotal role in the development of abnormalities associated with the metabolic syndrome( Reference Phillips and Prins 24 ). Abdominal fat comprises fat depots accumulated in subcutaneous and visceral areas. Compared with subcutaneous fat, visceral adipose tissue is more metabolically active, more insulin resistant and more prone to lipolysis, the latter mainly through stimulation by catecholamines( Reference Ibrahim 25 ). The critical impact of visceral adipose tissue on metabolism is due to its proximity to the liver which allows direct drainage of visceral tissues, including NEFA and adipokines, into the portal vein.
In slim individuals, small adipocytes function as a sink for absorbed NEFA and TAG. In obese individuals, however, adipocytes become large and disordered. Large adipocytes are insulin-resistant and hyperlipolytic. This type of adipocytes is found more in visceral adipose tissue while subcutaneous fat contains rather insulin-sensitive small adipocytes( Reference Ibrahim 25 ).
Excessive release of NEFA from adipose tissue induces insulin resistance in the peripheries and the liver( Reference Rachek 26 , Reference Ebbert and Jensen 27 ). Hence, there may be a vicious cycle between the level of NEFA and the extent of insulin resistance( Reference Asrih and Jornayvaz 28 ). Insulin resistance in turn prevents uptake of glucose and fatty acids by cells, thus increasing their half-life in the circulation( Reference Ibrahim 25 ). Hepatic insulin resistance as well as high levels of NEFA stimulate gluconeogenesis which consequently causes hyperglycaemia, one of the features of the metabolic syndrome( Reference Samson and Garber 29 ). High concentrations of unused glucose and fatty acids in the blood expedite oxidative reactions and consequently instigate release of inflammatory cytokines from adipose tissue( Reference Standl 30 , Reference Chen, Yu and Xiong 31 ). Inflammatory cytokines are also involved in insulin resistance( Reference Paragh, Seres and Harangi 32 ).
One the other hand, excessive influx of NEFA into the liver results in fat deposition in hepatic cells, leading to fatty liver( Reference Milić, Lulić and Štimac 33 ). Also, hepatic overload of NEFA can result in overproduction of VLDL and subsequently hypertriacylglycerolaemia, another component of the metabolic syndrome( Reference Subramanian and Chait 34 ). Hypertriacylglycerolaemia activates cholesteryl ester transfer protein, the enzyme involved in the transfer of TAG from VLDL to HDL and LDL in exchange for cholesteryl esters( Reference Tenenbaum, Klempfner and Fisman 35 ). This results in increased TAG content of HDL and LDL particles. TAG-enriched HDL is cleared from blood more rapidly, leading to decreased concentration of HDL particles in blood. Likewise, TAG-enriched LDL particles are more susceptible to lipolytic activity of lipoprotein lipase and hepatic lipase, thus decreasing the size of LDL particles, which have a higher atherogenic activity.
Compared with other components, the mechanisms of metabolic syndrome-related hypertension are less recognized, but a role for the renin–angiotensin–aldosterone system has been suggested. Although the liver is the major source of angiotensinogen under normal conditions, in obese individuals adipocytes may also produce angiotensinogen( Reference Putnam, Shoemaker and Yiannikouris 36 ). Also, hyperglycaemia stimulates renin release and increases the expression of renin receptor, angiotensin and angiotensin-converting enzyme in animal kidneys. Hyperinsulinaemia may additionally increase blood pressure through stimulation of the sympathetic nervous system( Reference Samson and Garber 29 ). Hypertension may also occur as a result of endothelium malfunction due to destroying NO by reactive oxygen species produced following hyperglycaemia and high plasma NEFA( Reference Samson and Garber 29 ). Adipose tissue-derived cytokines may contribute to high blood pressure, as well( Reference Kang 37 ). Fig. 1 depicts the sequence and interconnections between events that lead to the development of the metabolic syndrome.
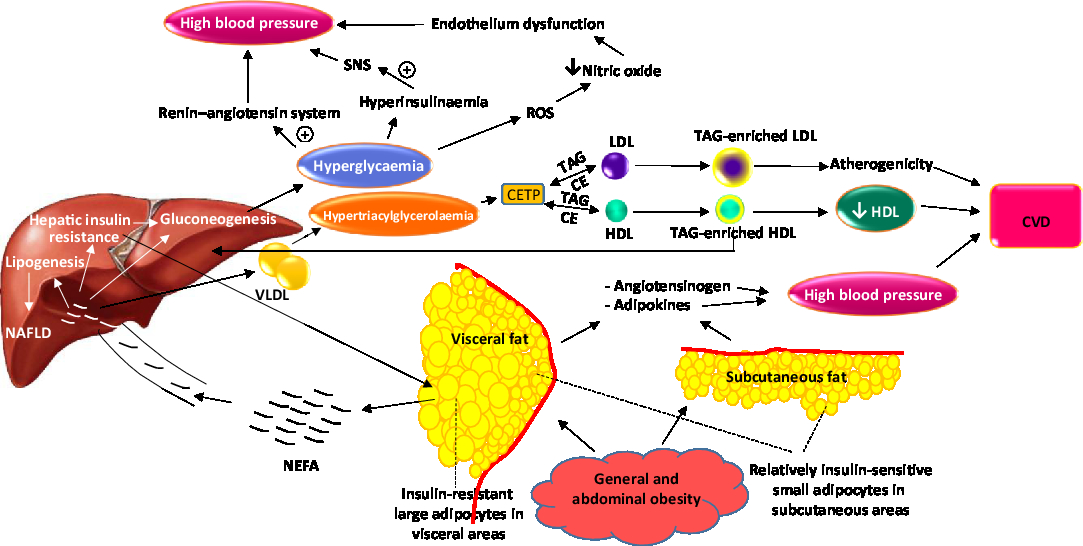
Fig. 1. Overview of pathological events which successively occur and lead to components of the metabolic syndrome. SNS, sympathetic nervous system; CETP, cholesteryl ester transfer protein; CE, cholesteryl esters; NAFLD, non-alcoholic fatty liver disease. For a colour figure, see the online version of the paper.
Dietary Approaches to Stop Hypertension (DASH) diet
The DASH diet was proposed for the first time in 1997 for the control of blood pressure( Reference Appel, Moore and Obarzanek 13 ). The diet was rich in fruits (5·2 servings/d), vegetables (4·4 servings/d) and low-fat dairy foods (2 servings/d) and with reduced total (25·6 % of energy) and saturated (7 % of energy) fat. The diet also had higher quantities of nuts, seeds and legumes (0·7 servings/d), whole grains (3·8 servings/d) and fish (0·5 servings/d), and lower amounts of red meats (0·5 servings/d), sweets and sugar-sweetened beverages (0·7 servings/d). There was primarily no restriction on Na. Na content of the original DASH diet was approximately 3 g/d (equal to 8 g salt/d). However, complementary investigations revealed that dietary Na restriction to less than 6 g/d enhances DASH benefits on blood pressure( Reference Sacks, Svetkey and Vollmer 38 ).
Due to possessing specific food items, the DASH diet provides good amounts of fibre, K, Ca, Mg and antioxidants, and limited quantities of total fat, saturated fat, cholesterol and Na (Fig. 2)( Reference Najafi, Faghih and Akhlaghi 39 ). Consumption of each of the beneficial dietary components and limiting ingestion of each of the unfavourable elements have proved to be advantageous for the prevention of hypertension( Reference Nguyen, Odelola and Rangaswami 40 ). However, the combination of these dietary components in the form of a dietary pattern provides more substantial benefits( Reference Chen, Maruthur and Appel 41 ).

Fig. 2. Major nutrients provided by Dietary Approaches to Stop Hypertension (DASH) components. For a colour figure, see the online version of the paper.
Although the DASH diet was initially designed for the prevention or control of hypertension, using a DASH diet has other metabolic rewards. For instance, epidemiological studies have shown benefits of DASH on the metabolic syndrome. In a large-scale cross-sectional study in Korea, the number of individuals with the metabolic syndrome was greatest in the DASH first quartile, which had the lowest consumption of protein, fibre, Ca and K, and the highest consumption of fat and Na( Reference Kang, Cho and Do 42 ). Also, in a 3·6-year cohort study on children and adolescents, OR of developing the metabolic syndrome in the highest, compared with the lowest, quartile of DASH score was 0·36( Reference Asghari, Yuzbashian and Mirmiran 43 ). The incidence of hypertension, high fasting glucose and abdominal obesity decreased along with strengthening adherence to the DASH diet( Reference Asghari, Yuzbashian and Mirmiran 43 ). Also, a 24-year prospective cohort study showed that adherence to the DASH diet was associated with a lower risk of CHD and stroke( Reference Fung, Chiuve and McCullough 44 ).
RCT have confirmed the findings of observational studies. For instance, 8-week consumption of DASH by overweight and obese individuals decreased body weight, serum TAG, VLDL-cholesterol, total to HDL-cholesterol ratio, insulin levels and insulin resistance, and increased the insulin sensitivity index( Reference Razavi Zade, Telkabadi and Bahmani 45 ). Likewise, in type 2 diabetes patients, DASH reduced body weight, waist circumference, fasting blood glucose levels, HbA1c, LDL-cholesterol, systolic and diastolic blood pressure, and inversely increased HDL-cholesterol( Reference Azadbakht, Fard and Karimi 46 ). Also, in a large-scale 8-week trial, the DASH diet reduced estimated 10-year CHD risk by 18 and 11 %, in comparison with regular and fruit and vegetable-rich diets, respectively( Reference Chen, Maruthur and Appel 41 ). In agreement, a meta-analysis predicted a 13 % reduction in the 10-year Framingham CVD risk score following consumption of DASH( Reference Siervo, Lara and Chowdhury 47 ). DASH decreased systolic and diastolic blood pressure, total and LDL-cholesterol, but no change in HDL-cholesterol and TAG was observed( Reference Siervo, Lara and Chowdhury 47 ).
Effect of Dietary Approaches to Stop Hypertension (DASH) on components of the metabolic syndrome
Waist circumference
Although reducing energy intake is not among the guidelines of the DASH diet, weight loss strategies are always recommended with DASH to improve its effectiveness( Reference Blumenthal, Babyak and Sherwood 48 ). In a clinical trial on hypertensive overweight patients, addition of energy restriction and aerobic exercise to the DASH diet led to lower glucose levels after an oral glucose load, improved insulin sensitivity, and lower total cholesterol and TAG compared with the DASH diet alone( Reference Blumenthal, Babyak and Sherwood 48 ). However, a meta-analysis of RCT showed that compared with non-DASH diets with equal energy, individuals on a DASH diet lose more weight, BMI and waist circumference( Reference Soltani, Shirani and Chitsazi 49 ). Like any other nutritional intervention, long treatments may not function as effectively as short interventions. A 1-year home-delivered DASH meal did not affect BMI in a group of mostly overweight or obese older adults with hypertension and/or hyperlipidaemia( Reference Racine, Lyerly and Troyer 50 ).
Subcutaneous fat responds more rapidly than visceral fat to weight loss programmes. A meta-analysis using different strategies for weight loss (diet/physical activity, hypoenergetic diets, promoting drugs, testosterone and bariatric surgery) showed that the decrease of subcutaneous fat was greater than visceral fat with no difference between different strategies( Reference Merlotti, Ceriani and Morabito 51 ). No intervention preferentially targets visceral fat. Visceral adipose tissue is lost with moderate weight loss, but the effect is attenuated with greater weight losses( Reference Chaston and Dixon 52 ). The decrease in weight, visceral adipose tissue, and less significantly subcutaneous fat, is associated with improved metabolic conditions, in particular decreased insulin levels( Reference Merlotti, Ceriani and Morabito 51 ).
Fasting glucose and insulin
The DASH diet has also been effective on glucose and insulin levels. In an interventional study, the DASH diet plus weight loss and physical activity improved fasting insulin and glucose( Reference Ard, Grambow and Liu 53 ). Similarly, in pregnant women with gestational diabetes, the DASH diet for 4 weeks resulted in decreased fasting plasma glucose, serum insulin levels and insulin resistance index( Reference Asemi, Samimi and Tabassi 18 ). However, meta-analyses have not shown the effect of DASH on fasting blood glucose and insulin resistance( Reference Shirani, Salehi-Abargouei and Azadbakht 54 , Reference Siervo, Lara and Chowdhury 47 ), but a decreasing effect on fasting insulin levels was observed( Reference Shirani, Salehi-Abargouei and Azadbakht 54 ).
Blood lipids
In the original DASH study, the DASH diet decreased total cholesterol, LDL-cholesterol and, to a lesser extent, HDL-cholesterol but no change in TAG levels was observed( Reference Obarzanek, Sacks and Vollmer 55 ). A meta-analysis of RCT similarly demonstrated the beneficial effect of DASH on total and LDL-cholesterol but no change in HDL-cholesterol and TAG( Reference Siervo, Lara and Chowdhury 47 ). The effect of DASH on total and LDL-cholesterol is probably the result of a decreased intake of saturated fat because DASH contains three times less red meat than a control diet( Reference Appel, Moore and Obarzanek 13 ). The Ca content of DASH may also contribute to the cholesterol-lowering effect of DASH considering that the amount of dairy products (low-fat + regular fat) in DASH is five times more than a control diet( Reference Appel, Moore and Obarzanek 13 ). The reduction in HDL-cholesterol is an unfavourable consequence of DASH which could be due to decreasing total dietary fat( Reference Obarzanek, Sacks and Vollmer 55 ).
Blood pressure
Na restriction is one of the components of DASH for reducing blood pressure. In fact, Na restriction was added to the initially designed DASH diet in order to augment its blood pressure-lowering effect. Decreased consumption of saturated fats may also control blood pressure( Reference Vafeiadou, Weech and Altowaijri 56 ) at least partially through enhancement of serum concentrations of angiotensin-converting enzyme( Reference Schüler, Osterhoff and Frahnow 57 ). In addition, high amounts of fruit and vegetables in DASH provide great quantities of K, Mg and fibre, all of which have been shown to reduce blood pressure. These dietary components were associated with lower blood pressure in observational and interventional studies( Reference Houston and Harper 58 , Reference Aleixandre and Miguel 59 ). However, K, Mg and fibre supplements were less effective in lowering blood pressure of obese hypertensive patients than DASH, indicating that there are other components in the DASH diet which help in blood pressure control( Reference Al-Solaiman, Jesri and Mountford 60 ).
Possible mechanisms of Dietary Approaches to Stop Hypertension (DASH) protection against the metabolic syndrome
Potassium
Blood pressure
Fruits and vegetables of DASH provide ample quantities of K. Epidemiological, observational and interventional studies have proposed a protective effect of K on blood pressure and the prevention of hypertension( Reference Houston 61 ). Epidemiological studies show that blood pressure is lower in populations with higher fruit and vegetable consumption( Reference Khaw and Rose 62 ). In the INTERSALT study( 63 ), a worldwide epidemiological study of large sample size from thirty-two countries, K intake (as measured by 24 h urinary K excretion) was an important determinant of population blood pressure, independent of Na( 63 ). A meta-analysis of RCT showed that K supplementation lowered blood pressure in hypertensive patients and those who did not use antihypertensive medications( Reference Binia, Jaeger and Hu 64 ). The American Heart Association( Reference Appel, Brands and Daniels 65 ) and American Society of Hypertension( Reference Appel, Giles and Black 66 ) suggest 4·7 g dietary K per d. This amount is equal to the amount of K provided in the DASH diet( Reference Appel, Moore and Obarzanek 13 ).
In addition to K intake, the ratio of dietary Na to K is also important for blood pressure control. In high K consumptions, elevated dietary Na may not lead to increased blood pressure( Reference Rodrigues, Baldo and Machado 67 ). A large cross-sectional study estimated adjusted OR for hypertension as 1·40 (95 % CI 1·07, 1·83), 0·72 (95 % CI 0·53, 0·97) and 1·30 (95 % CI 1·05, 1·61), respectively, for the highest v. the lowest quartiles of intake of Na, K or Na:K ratio( Reference Zhang, Cogswell and Gillespie 68 ). A high dietary Na:K ratio was also positively associated with cardiovascular risk and mortality( Reference Cook, Obarzanek and Cutler 69 ). Genetics may also affect blood pressure response to dietary Na and K. A family-based study in China showed that a genetic polymorphism in the adiponectin gene may play a role in blood pressure change in response to dietary Na and K although mechanisms of this contribution have not been discovered( Reference Chu, Wang and Ren 70 ).
K causes vasodilation through hyperpolarisation of vascular smooth muscle cells through stimulation of Na+/K+ ATPase pumps, increased activity of the Na–Ca exchanger, and subsequently decreased cytosolic Ca (Fig. 3)( Reference Haddy, Vanhoutte and Feletou 71 ). Other possible mechanisms by which K affects blood pressure include increased Na excretion, reduced sensitivity to vasoconstrictive activity of noradrenaline and angiotensin II, modulation of baroreceptor sensitivity, and improved insulin sensitivity( Reference Houston 61 , Reference Stone, Martyn and Weaver 72 ). However, K also benefits the cardiovascular system and prevents mortality independent of its effect on blood pressure through mechanisms such as inhibition of vascular smooth muscle cell proliferation, thrombosis and macrophage adhesion to the vascular wall( Reference He and MacGregor 73 ).
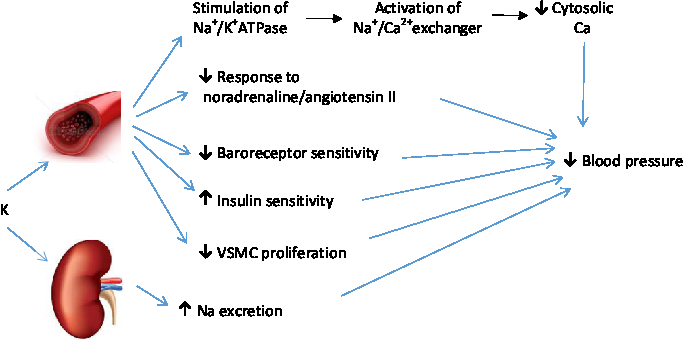
Fig. 3. Mechanisms by which potassium prevents hypertension. VSMC, vascular smooth muscle cells. For a colour figure, see the online version of the paper.
Glucose tolerance
K has beneficial effects other than blood pressure reduction. A large cohort study with 9 years of in-person follow-up and 17 years of telephone follow-up indicated that serum K level is an independent predictor of type 2 diabetes mellitus incidence( Reference Chatterjee, Yeh and Shafi 74 ). Compared with individuals with normal to high serum K levels (5·0–5·5 mEq/l), those with serum K levels < 4·0 mEq/l, 4·0 to < 4·5 mEq/l, and 4·5 to < 5·0 mEq/l had an adjusted hazard ratio of incident diabetes mellitus of 1·64, 1·64 and 1·39, respectively. This type of association was also observed for dietary and urinary K in African Americans but not whites( Reference Chatterjee, Colangelo and Yeh 75 ). A meta-analysis of prospective cohort studies confirmed the inverse association between serum K level and diabetes risk but no association between dietary or urinary K and the risk of diabetes was observed( Reference Peng, Zhong and Mi 76 ). K may be involved in glucose-dependent insulin secretion from pancreatic β-cells. In the resting state, β-cell membranes are polarised by K efflux from ATP-sensitive K channels. Upon a glucose load, uptake of glucose by β-cells leads to production of ATP and inactivation of ATP-sensitive K channels. Subsequently, depolarisation of β-cell membranes leads to opening voltage-gated Ca2+ channels, Ca influx, a rise in intracellular Ca concentrations and insulin secretion( Reference Ashcroft 77 ). Besides the proposed insulin secretagogic effect of K, a protective effect of K against salt-induced insulin resistance has been reported through suppression of IL-17A, an inflammatory cytokine involved in the metabolic syndrome, especially in salt loading states( Reference Wen, Wan and Zhou 78 ). In addition to the direct effect of K on insulin secretion, an inverse association between serum K and fasting insulin levels was observed( Reference Chatterjee, Yeh and Shafi 74 ). This inverse association is proposed to be due to stimulation of K entry to cells by insulin, leading to reduced serum K levels( Reference Sterns, Grieff and Bernstein 79 ).
Magnesium
Blood pressure
Due to containing green leafy vegetables, nuts, legumes, seeds and whole grains, the DASH diet has a high Mg content. A large cross-sectional study showed that a higher DASH score is associated with higher urine concentrations of K and Mg( Reference Taylor, Stampfer and Mount 80 ). Many health benefits have been attributed to Mg, but Mg is famous for its beneficial effects on the cardiovascular system, particularly hypertension. Atherosclerosis, hypertension, arrhythmias, dyslipidaemia, impaired glucose tolerance, insulin resistance and increased risk of the metabolic syndrome are reported in Mg deficiency( Reference Gröber, Schmidt and Kisters 81 ). A meta-analysis of prospective cohort studies supported the inverse dose–response relationship between dietary Mg intake and the risk of hypertension( Reference Han, Fang and Wei 82 ). Meta-analyses of RCT have also reported benefits of Mg supplementation on blood pressure( Reference Kass, Weekes and Carpenter 83 , Reference Zhang, Li and Del Gobbo 84 ).
Various mechanisms have been suggested for the antihypertensive effect of Mg. Mg inhibits the renin–angiotensin–aldosterone system, by counteracting angiotensin II and inhibiting aldosterone production. This inhibition may be exerted through activation of Na+/K+ ATPase and decreasing intracellular Na and Ca levels( Reference Schutten, Joosten and de Borst 85 ). Mg also acts as a Ca antagonist by raising the excitation threshold of voltage-gated Ca2+ channels, thus decreasing vascular smooth muscle cell contractility( Reference Kostov and Halacheva 86 , Reference Sontia and Touyz 87 ). Moreover, the production of vasoactive agents, such as endothelin-1, in vascular cell membranes is decreased in the presence of Mg. Furthermore, along with ATP, Mg mitigates catecholamine release from the adrenal gland in response to Ca. Mg may also reduce vascular stiffness( Reference Joris, Plat and Bakker 88 ), probably through regulating the synthesis of structural molecules in the vascular extracellular matrix( Reference Kostov and Halacheva 86 ). A part of the vasodilatory potential of Mg may be exerted through inhibiting systemic inflammation( Reference Song, Li and van Dam 89 ). Interestingly, in salt-induced hypertension a decrease in intracellular Mg along with accumulation of intracellular Ca is observed( Reference Barbagallo, Dominguez and Resnick 90 ).
Glucose tolerance
Prospective cohort studies and meta-analyses have also shown Mg to be advantageous in other metabolic disorders. For instance, in a prospective cohort study with 15 years follow-up, individuals in the highest quartile of Mg had lower risk (hazard ratio 0·69) for the development of the metabolic syndrome compared with those in the lowest quartile( Reference He, Liu and Daviglus 91 ). Also, a longitudinal study with 15·6 years of follow-up on a non-diabetic Japanese population showed decreased incidence of type 2 diabetes with a hazard ratio of 0·63 in the highest quartile of Mg intake compared with the lowest( Reference Hata, Doi and Ninomiya 92 ). Moreover, a meta-analysis of cohort studies indicated a significant inverse association between Mg intake and diabetes risk( Reference Schulze, Schulz and Heidemann 93 ). In another meta-analysis of prospective cohort studies an inverse correlation between plasma Mg levels and incidence of hypertension, CHD and type 2 diabetes was observed( Reference Wu, Xun and Tang 94 ).
Mg is also involved in insulin function. Mg is necessary for all reactions in which high-energy phosphate bonds are transferred. It functions as a cofactor for phosphorylation of tyrosine kinase at the insulin receptor( Reference Kostov and Halacheva 86 ). Therefore, decreased Mg may impair insulin signalling. Mg may also improve insulin secretion as reported by cross-sectional( Reference Rodríguez-Morán and Guerrero-Romero 95 ) and interventional( Reference Guerrero-Romero and Rodríguez-Morán 96 ) studies. Individuals with the metabolic syndrome generally have inadequate dietary Mg intake, and thus Mg supplementation may help in correction of their metabolic disturbances( Reference Wang, Persuitte and Olendzki 97 , Reference Morais, Severo and Santos 98 ). Meta-analyses of controlled trials have supported the effectiveness of Mg supplementation in reducing fasting glucose( Reference Song, He and Levitan 99 , Reference Veronese, Watutantrige-Fernando and Luchini 100 ) and raising HDL-cholesterol levels in patients with type 2 diabetes( Reference Song, He and Levitan 99 ). Another meta-analysis revealed that Mg supplementation for more than 4 months improved insulin resistance and fasting glucose in both diabetic and non-diabetic individuals( Reference Simental-Mendía, Sahebkar and Rodríguez-Morán 101 ).
It is worth noting that it is not only Mg which affects glucose tolerance but hyperglycaemia, even in transient form as occurs in non-diabetic subjects following consumption of meals, also decreases intracellular Mg and increases intracellular Ca, indicating that Mg depletion can be both a cause and consequence of hyperglycaemia( Reference Barbagallo, Dominguez and Resnick 90 ). In addition, insulin resistance may impair Mg reabsorption in the kidney resulting in urinary Mg excretion( Reference Gommers, Hoenderop and Bindels 102 ).
Calcium and dairy products
Weight
One of the important ingredients of DASH is Ca. In the DASH diet, Ca is mainly supplied by dairy products and to a less extent by green leafy vegetables, soya, nuts, particularly almonds, and fish when the bones are eaten. Ca and dairy products protect against the metabolic syndrome through a variety of mechanisms. Reduced adiposity is one of the mechanisms of Ca against the metabolic syndrome. A meta-analysis of cross-sectional studies showed the inverse association between dairy product consumption and decreased risk of obesity( Reference Wang, Wu and Zhang 103 ). However, meta-analyses of randomised clinical trials did not show a beneficial effect of dairy products on the weight of overweight/obese adults but advantages were reported when dairy products were consumed in combination with energy-restricted diets( Reference Chen, Pan and Malik 104 – Reference Abargouei, Janghorbani and Salehi-Marzijarani 106 ). The evidence suggests that individuals with a low Ca intake may benefit from dairy products for decreasing waist circumference and sagittal abdominal diameter( Reference Wennersberg, Smedman and Turpeinen 107 ). The reductions in weight and waist circumference may eventually lead to lower blood pressure and control of hypertension( Reference Park, Lee and Kim 108 ).
There are mechanisms by which Ca may engage in weight control. Dietary Ca may decrease fat absorption through formation of insoluble fatty acid soaps in the intestine( Reference Christensen, Lorenzen and Svith 109 ). On the other hand, stimulation of parathyroid hormone in response to low serum Ca concentrations results in the elevation of intracellular Ca concentration and the triggering of adiposity through stimulation of enzymes involved in lipogenesis and inhibition of lipolysis( Reference Zemel, Teegarden and Loan 110 ). Sufficient ingestion of dietary Ca can hinder this process through optimising serum Ca levels and inhibiting stimulation of parathormone, leading to reduced adipogenesis and body fat mobilisation and oxidation.
Compared with supplemental Ca, dairy Ca has a greater effect on weight, suggesting that dairy components other than Ca may be effective( Reference Zemel, Thompson and Milstead 111 , Reference Zemel 112 ). For instance, dairy proteins may help in the suppression of appetite. Whey is supposed to have a stronger effect than casein on appetite control but each of whey and casein executes distinct mechanisms. While the casein fraction postpones gastric emptying following coagulation in the stomach, whey induces satiety due to rapid digestion, absorption and elevation of amino acids in the blood( Reference Bendtsen, Lorenzen and Bendsen 113 ). Bioactive compounds, low-glycaemic index carbohydrates and Ca content of dairy foods may also be implicated in appetite control( Reference Dougkas, Reynolds and Givens 114 ). It is worthwhile to note that the beneficial effect of dairy products on weight occurs when low-fat dairy products are consumed, but whole-fat dairy products may promote weight gain( Reference Alonso, Zozaya and Vázquez 115 ).
Glucose tolerance
Decreased weight has positive metabolic consequences such as improved insulin sensitivity. A meta-analysis of observational studies indicates a relatively consistent association between low Ca or dairy product intake and prevalent type 2 diabetes or the metabolic syndrome( Reference Pittas, Lau and Hu 116 ). Similarly, a meta-analysis of cohort studies suggested an inverse association between the intake of dairy products, low-fat dairy products and cheese and the risk of type 2 diabetes( Reference Aune, Norat and Romundstad 117 ). A prospective cohort study showed an association of higher consumption of dairy products and Ca with lower 9-year incidence of the metabolic syndrome and impaired fasting glucose or type 2 diabetes( Reference Fumeron, Lamri and Abi Khalil 118 ). Also, a prospective cohort study with 20 years of follow-up revealed the inverse association of milk and dairy product consumption and prevalence of the metabolic syndrome( Reference Elwood, Pickering and Fehily 119 ). A possible mechanism for dairy product protection against type 2 diabetes is the low-glycaemic index of milk and other dairy foods which suppresses postprandial hyperglycaemia( Reference Ballard and Bruno 120 ). The beneficial effect of dairy products on weight may also contribute to the prevention of type 2 diabetes by dairy foods. Moreover, milk proteins, in particular whey, have insulinotropic activity when consumed with meals( Reference Nilsson, Stenberg and Frid 121 ). Ca is also essential for insulin secretion by pancreatic β-cells (Fig. 4)( Reference Pittas, Lau and Hu 116 ).

Fig. 4. The role of calcium in secretion of insulin by pancreatic β-cells. For a colour figure, see the online version of the paper.
Blood pressure
Blood pressure is also affected by Ca and dairy product intake. Meta-analyses of prospective cohort studies support the inverse association between low-fat dairy products and risk of elevated blood pressure( Reference Soedamah-Muthu, Verberne and Ding 122 , Reference Ralston, Lee and Truby 123 ). The pooled relative risk (RR) per 200 g/d was 0·97 (95 % CI 0·95, 0·99) for total dairy products, 0·96 (95 % CI 0·93, 0·99) for low-fat dairy products and 0·96 (95 % CI 0·94, 0·98) for milk( Reference Soedamah-Muthu, Verberne and Ding 122 ). The protective effect may be greater in an at-risk population( Reference Toledo, Delgado-Rodríguez and Estruch 124 ). On the other hand, high-fat dairy products may have an adverse effect on blood pressure due to the increasing effect on weight( Reference Alonso, Zozaya and Vázquez 115 ). Both casein and whey constituents of milk protein have been effective in reducing blood pressure and arterial stiffness( Reference Fekete, Givens and Lovegrove 125 – Reference Boelsma and Kloek 127 ). The effect is supposed to be exerted mainly by bioactive tripeptides which are produced in the gut following digestion of milk proteins. A part of the beneficial effect of milk on blood pressure may result from its K. Dairy products have a relatively high K content which contributes largely to daily K intake( Reference Ballard and Bruno 120 ). Furthermore, through prevention of transient postprandial hyperglycaemia, dairy products as a low-glycaemic index food prevent hyperglycaemia-induced oxidative stress, thus improving NO bioavailability and vascular function( Reference Ballard and Bruno 120 ).
Sodium
Blood pressure
Although reducing Na intake was not initially among the principles of the DASH diet( Reference Appel, Moore and Obarzanek 13 ), Na restriction is recommended along with DASH in order to augment its blood pressure-lowering effect( Reference Sacks, Svetkey and Vollmer 38 ). However, reduced blood pressure following consumption of DASH does not occur due to Na restriction only, but other DASH components are also effective in the regulation of blood pressure. In fact, in the absence of salt restriction, a reduction in blood pressure is still observed following DASH consumption( Reference Appel, Moore and Obarzanek 13 ). Reducing Na intake from a high-Na ordinary diet (about 3·5 g/d Na) to a low-Na DASH diet (1·8 g/d Na) resulted in mean systolic blood pressure decreases of 7·1 and 11·5 mmHg in normotensive and hypertensive individuals, respectively( Reference Sacks, Svetkey and Vollmer 38 ). The American Heart Association and American College of Cardiology recommend no more than 2·4 g Na per d (equal to 6 g salt per d)( Reference Eckel, Jakicic and Ard 128 ). A further decrease to 1·5 g/d may be required for high-risk patients, for instance, for those at risk of stroke( Reference Van Horn 129 ). On the other hand, very intensive Na restriction is also not recommended because a low Na intake is associated with increased cardiovascular mortality, indicating that a U-shaped relationship may exist between Na intake and health outcomes( Reference Graudal, Jürgens and Baslund 130 ).
Mechanisms by which Na increases blood pressure include expansion of extracellular fluid volume and increased cardiac output( Reference Feng, Dell’Italia and Sanders 131 ), impaired renin–angiotensin–aldosterone system( Reference Shimosawa 132 ), activation of the sympathetic nervous system( Reference Gavras and Gavras 133 ), augmented vascular smooth muscle cell proliferation( Reference Liu, Hitomi and Rahman 134 ) and reduced NO bioavailability, which decreases endothelium-dependent vasodilation( Reference Boegehold 135 ). Kidneys and the vascular system play pivotal roles in modifying the haemodynamic changes caused by Na, but their function is impaired in salt sensitivity( Reference Feng, Dell’Italia and Sanders 131 ).
Glucose tolerance
There are other benefits with Na restriction which may help against the metabolic syndrome. For instance, low dietary Na reduces insulin secretion without effecting insulin sensitivity, probably through interruption of the renin–angiotensin–aldosterone system( Reference Luther, Byrne and Yu 136 ). High aldosterone concentrations may impair β-cell function( Reference Mosso, Carvajal and Maiz 137 ). Also, a direct relationship between aldosterone, insulin resistance and hyperinsulinaemia( Reference Colussi, Catena and Lapenna 138 ) has been suggested.
Fibre
Weight
Prospective studies on cereal fibre and whole grains reported small but significant reductions in weight gain( Reference Cho, Qi and Fahey 139 ). A meta-analysis of RCT also showed reductions in weight, BMI and body fat in overweight and obese adults consuming soluble fibre supplements( Reference Thompson, Hannon and An 140 ). Due to resistance to gastrointestinal enzymes, dietary fibre is devoid of energy, and fibre-containing foods such as whole grains, vegetables and fruit have low energy density. In addition, because of their bulking properties, fibre-containing foods stimulate satiety signals without adding much into daily energy intake( Reference Papathanasopoulos and Camilleri 141 ). As a result of their viscosity and formation of gel in the stomach, soluble fibres delay gastric emptying, thus slowing down food transition through the small intestine which results in decelerating glucose absorption( Reference Weickert and Pfeiffer 142 ). Slow glucose absorption suppresses the insulin response and prevents episodes of hypoglycaemia, thus minimising hunger sensations. Moreover, SCFA produced as a result of colonic fermentation of soluble fibres have shown potential in regulating appetite through suppressing hunger hormones and stimulating satiety hormones although large doses of fibre are required to exhibit such effects( Reference Nilsson, Johansson and Ekström 143 ). SCFA may also increase energy expenditure through increased thermogenesis and fat oxidation although this effect has not been investigated in human studies( Reference Byrne, Chambers and Morrison 144 ).
Glucose tolerance
A meta-analysis of prospective cohort studies indicated that a two servings/d increase in whole grain consumption was associated with a 21 % decrease in the risk of type 2 diabetes( Reference de Munter, Hu and Spiegelman 145 ). Another meta-analysis of cohort studies showed a reduced risk of diabetes with higher intake of cereal fibre (RR for extreme categories 0·67), but no significant association for fruit was observed( Reference Schulze, Schulz and Heidemann 93 ). Later meta-analyses showed the inverse association between intake of total dietary fibre and cereal fibre as well as insoluble fibre and fruit and risk of type 2 diabetes( Reference Yao, Fang and Xu 146 ). In a large case–control study, lower risk of diabetes was associated with the intake of cereal and vegetable fibre, but not fruit fibre( 147 ). Meta-analyses of RCT have also shown benefits of low-glycaemic index foods in the prevention of diabetes( Reference Barclay, Petocz and McMillan-Price 148 ) or improvement of glycaemic control( Reference Thompson, Hannon and An 140 , Reference Brand-Miller, Hayne and Petocz 149 – Reference Bajorek and Morello 153 ).
The anti-diabetic effect of fibre may be partly due to its beneficial effect on weight. In a meta-analysis of cohort studies, the inverse association of fibre intake with diabetes risk diminished after adjustment for BMI( 147 ). Fibre also has a direct impact on gastric emptying which increases intestinal transit time. SCFA that are produced in the colon as a result of colonic bacteria fermentation can also delay gastric emptying and decelerate glucose absorption through stimulating secretion of anorectic hormones, peptide YY and glucagon-like peptide-1 (GLP-1)( Reference Scheithauer, Dallinga-Thie and de Vos 154 , Reference Canfora, Jocken and Blaak 155 ). There are also additional mechanisms by which SCFA may contribute to the prevention of diabetes. These include anti-inflammatory effect of SCFA, balancing composition and activity of gut microbiota, and reducing hepatic glucose production by suppressing gene expression of the gluconeogenic enzymes glucose 6-phosphatase and phosphoenolpyruvate carboxykinase( Reference den Besten, van Eunen and Groen 156 – Reference Kuo 158 ). The above-mentioned mechanisms are suggested for soluble fibre. Nevertheless, benefits of fibre on diabetes are related to both types of soluble and insoluble fibre. In fact, data for the anti-diabetic potential of insoluble fibre are stronger and more consistent than soluble fibre although the mechanisms of the protection are less recognised( Reference Davison and Temple 159 ). Insoluble fibre may exert its effect through accelerating secretion of glucose-dependent insulinotropic polypeptide (GIP) and insulin response following meals, thus reducing the postprandial glucose rise( Reference Weickert, Mohlig and Koebnick 160 ). In addition, the consumption of high-cereal fibre diets may prevent high-protein diet-induced insulin resistance by interfering with protein absorption( Reference Weickert, Roden and Isken 161 ). High-protein diets are generally applied in weight loss programmes and impair insulin signalling through phosphorylation of serine kinase-6-1( Reference Weickert, Roden and Isken 161 ).
Lipids
In an umbrella review, thirty-one meta-analyses reported reductions in the RR of CVD mortality (RR 0·77–0·83), the incidence of CVD (RR 0·72–0·91), CHD (RR 0·76–0·93) and stroke (RR 0·83–0·93) in the highest v. the lowest dietary fibre intake( Reference McRae 162 ). The evidence also suggests effectiveness of fibre on risk factors of CVD. Meta-analyses on supplementation studies using viscous soluble fibres, β-glucan, psyllium or konjac glucomannan also reported statistically significant reductions in both total and LDL-cholesterol concentrations( Reference McRae 162 – Reference Ho, Jovanovski and Zurbau 164 ). Similarly, a systematic review revealed that a breakfast based on oats, barley or psyllium may lower cholesterol concentrations( Reference Williams 165 ). In another meta-analysis, TAG levels did not change but HDL-cholesterol concentrations were increased slightly by fibre( Reference Hartley, May and Loveman 166 ). The mechanisms involved include: (1) interfering with enterohepatic circulation through prevention re-absorption of bile acids in the ileum, thus enhancing utilisation of blood cholesterol for de novo synthesis of bile acids; (2) slowing glucose absorption and subsequently suppressing insulin response, thereby reducing stimulation of hepatic cholesterol synthesis by insulin; (3) increasing hepatic LDL receptors; and (4) diminution of intestinal cholesterol absorption and its hepatic synthesis by SCFA( Reference Papathanasopoulos and Camilleri 141 , Reference Gunness and Gidley 167 , Reference Chen, Xu and Huang 168 ). Compared with soluble fibres, insoluble fibres exhibit a smaller reduction on blood cholesterol, but binding bile acids has been mentioned as their mechanism of action against blood cholesterol( Reference van Bennekum, Nguyen and Schulthess 169 ).
Blood pressure
A meta-analysis of RCT showed a lowering effect of viscous soluble fibre on systolic and diastolic blood pressure( Reference Khan, Jovanovski and Ho 170 ). Psyllium had a stronger effect than β-glucan, guar gum, konjac and pectin. Another meta-analysis indicated the effectiveness of dietary fibre in trials conducted on hypertensive patients and in trials with an intervention duration of ≥ 8 weeks but reduction in normotensive subjects was less conclusive( Reference Whelton, Hyre and Pedersen 171 ). However, compared with other components of the metabolic syndrome, the mechanisms of the effect of fibre on blood pressure are less recognised. The beneficial effect of fibre on blood pressure may have resulted from of its impact on weight. Also, some of the antihypertensive effect of fibre may be exerted through amelioration of insulin resistance and reducing insulin concentrations( Reference Aleixandre and Miguel 59 , Reference Sarafidis and Bakris 172 ). Insulin has an antinatriuretic potential by which it stimulates renal Na reabsorption( Reference Sarafidis and Bakris 172 ). Therefore, reduction of insulin concentrations could be beneficial for blood pressure. Furthermore, fermentation of soluble fibre in the distal intestine and colon produces acidic metabolites which may improve the absorption of minerals that are advantageous for blood pressure( Reference Aljuraiban, Griep and Chan 173 ). Since hypercholesterolaemia and hypertension are closely related, fibre may hinder hypertension by preventing hypercholesterolaemia( Reference Tuñón, Martín-Ventura and Blanco-Colio 174 ).
Antioxidants
Oxidative stress is a common feature of the metabolic syndrome( Reference Ford 175 ). Reactive oxygen species are produced during normal metabolism by mitochondria and extra-mitochondrial systems. However, the production of reactive oxygen species is increased in pathological conditions including obesity and the metabolic syndrome( Reference Ilkun and Boudina 176 ). In oxidative stress conditions, antioxidants are depleted in cellular and extracellular compartments. A case–control study showed that plasma levels of vitamins A, C and E are significantly lower in patients with the metabolic syndrome than in healthy subjects( Reference Godala, Materek-Kuśmierkiewicz and Moczulski 177 ). Therefore, consumption of antioxidants such as polyphenols, vitamin C, vitamin E and carotenoids may correct metabolic syndrome-associated oxidative stress( Reference Gregório, De Souza and de Morais Nascimento 178 ). Fruit, vegetables and nuts of DASH provide good quantities of antioxidant vitamins and polyphenols.
Weight
A systematic review of observational studies showed that obese individuals in any age group possess lower concentrations of antioxidants( Reference Hosseini, Saedisomeolia and Allman-Farinelli 179 ). Plasma levels of carotenoids, vitamins E and C, as well as Zn, Mg and Se were inversely correlated with obesity and body fat mass( Reference Hosseini, Saedisomeolia and Allman-Farinelli 179 ). In addition to antioxidant vitamins and minerals, polyphenols present in fruits and vegetables can prevent obesity. For instance, meta-analyses of RCT have shown the anti-obesity effect of flavanols( Reference Akhlaghi, Ghobadi and Mohammad Hosseini 180 ) and isoflavones( Reference Akhlaghi, Zare and Nouripour 181 ). The anti-obesity effect of polyphenols may be exerted through increasing β-oxidation of fatty acids, induction of satiety, stimulating thermogenesis in brown adipose tissue, increasing lipolysis, control of adipocyte differentiation, down-regulation of fatty acid synthase gene expression, and functioning as a prebiotic for gut microbiota (Fig. 5)( Reference Castro-Barquero, Lamuela-Raventós and Doménech 182 – Reference Lin and Lin-Shiau 186 ). Similarly, carotenoids may deter adiposity by enhancing fat oxidation and increasing energy waste in brown and white adipocytes( Reference Bonet, Canas and Ribot 187 ). Likewise, vitamin C may inhibit adipocyte differentiation, increase lipolysis and prevent glucose uptake by adipocytes( Reference Garcia-Diaz, Lopez-Legarrea and Quintero 188 ).
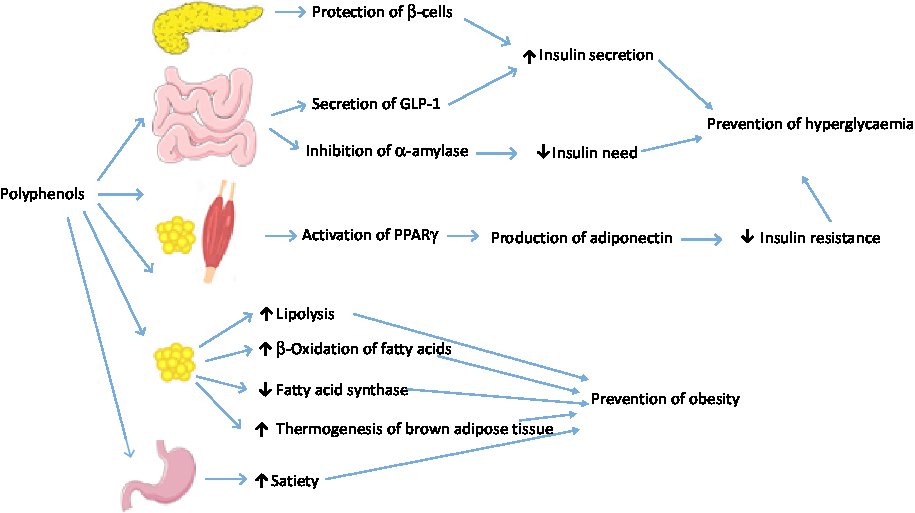
Fig. 5. Mechanisms of polyphenols against hyperglycaemia and obesity. GLP-1, glucagon-like peptide 1. For a colour figure, see the online version of the paper.
Glucose tolerance
The association between antioxidants and risk of diabetes has also been reported. A large retrospective cohort study with 5 years of follow-up on twenty-five communities across Japan showed an inverse association between consumption of green tea, coffee and total caffeine and the risk for type 2 diabetes( Reference Iso, Date and Wakai 189 ). Also, in a prospective cohort study with 10 years of follow-up in Japan, the hazard ratio for development of type 2 diabetes in the highest v. the lowest tertiles of serum α-carotene, β-cryptoxanthin and total carotenoids was 0·35, 0·43 and 0·41, respectively( Reference Sugiura, Nakamura and Ogawa 190 ). Various mechanisms have been suggested for the effect of polyphenols on glucose tolerance and insulin sensitivity (Fig. 5 ). Polyphenols may increase insulin secretion through protection of β-cell integrity( Reference Dragan, Andrica and Serban 191 ). They may also stimulate secretion of GLP-1, a hormone involved in quick postprandial insulin response, increase GLP-1 half-life, stimulate β-cells to secrete insulin, and increase insulin sensitivity in peripheral tissues( Reference Domínguez Avila, Rodrigo García and González Aguilar 192 ). In peripheral tissues, polyphenols may activate PPARγ, thus inducing adiponectin production and improving insulin resistance( Reference Umeno, Horie and Murotomi 193 ). In addition, cell culture studies indicate that green tea polyphenols inhibit gluconeogenesis in hepatocytes and stimulate glucose uptake in rat skeletal muscle cells by using a phosphatidylinositol 3-kinase-dependent mechanism that mimics metabolic actions of insulin( Reference Munir, Chandrasekaran and Gao 194 ). Polyphenols have also potential to decrease starch digestion by inhibiting α-amylase activity( Reference Xu, Shao and Xiao 195 ).
No meta-analysis has ever examined the effect of vitamin C or vitamin E on the risk of type 2 diabetes but meta-analyses on the effect of these antioxidants on patients with type 2 diabetes have produced conflicting results. Two meta-analyses showed no beneficial effect of vitamin E supplementation on glycaemic control of patients with type 2 diabetes but results were more promising for individuals with poor glycaemic control or low serum vitamin E levels( Reference Suksomboon, Poolsup and Sinprasert 196 , Reference Xu, Zhang and Tao 197 ). Another meta-analysis indicated that supplementation with vitamin C, vitamin E or their combination did not improve insulin resistance of type 2 diabetes patients( Reference Khodaeian, Tabatabaei-Malazy and Qorbani 198 ), but a limited number of RCT showed that a single dose of vitamin C may be beneficial in reducing fasting blood glucose of these patients( Reference Tabatabaei-Malazy, Nikfar and Larijani 199 ). Another meta-analysis revealed that vitamin C did not modify glucose, HbA1c and insulin concentrations in a population containing both diabetic and non-diabetic participants but subgroup analyses indicated that vitamin C significantly reduced glucose concentrations in patients with type 2 diabetes and in interventions longer than 30 d; also vitamin C administration had a greater effect on fasting compared with postprandial insulin concentration( Reference Ashor, Werner and Lara 200 ).
Mechanisms of the possible effect of vitamin C and vitamin E in the management of diabetes are largely unknown but the beneficial effect of these antioxidants in establishing glycaemic control may be exerted through a direct effect on pancreatic β-cells by protecting them from oxidative stress-induced cell damage( Reference Suksomboon, Poolsup and Sinprasert 196 ). Hyperglycaemia-associated oxidative stress is also suggested to be involved in the development of insulin resistance( Reference Henriksen, Diamond-Stanic and Marchionne 201 , Reference Ceriello and Motz 202 ). Thus, a part of antioxidants’ protection against type 2 diabetes may be delivered by suppression of oxidative stress. Carotenoids may reduce insulin resistance by induction of PPARγ as well as inhibiting c-Jun NH2-terminal kinase (JNK) and inhibitor κB kinase β (IKKβ) which induce insulin resistance through phosphorylation of insulin receptor substrate-1 (IRS-1)( Reference Roohbakhsh, Karimi and Iranshahi 203 ).
Lipids
There is limited evidence for the effect of antioxidants on blood lipids. A meta-analysis of RCT showed that vitamin C supplements may decrease TAG and LDL-cholesterol but the increase in HDL-cholesterol was not significant( Reference McRae 204 ). Similarly, meta-analyses of RCT showed that consumption of green tea catechins was associated with a significant reduction in total and LDL-cholesterol levels without causing significant changes in HDL-cholesterol or TAG levels( Reference Kim, Chiu and Barone 205 , Reference Zheng, Xu and Li 206 ). Another meta-analysis revealed beneficial effects of dark chocolate and cocoa products on total and LDL-cholesterol with no major effect on HDL-cholesterol and TAG( Reference Tokede, Gaziano and Djoussé 207 ). The cholesterol-lowering effect of polyphenols may be due to interfering with cholesterol absorption( Reference Bursill, Abbey and Roach 208 ), inhibiting cholesterol synthesis( Reference Kobayashi, Nishizawa and Inoue 209 ), and inducing expression and activity of LDL receptors( Reference Dávalos, Fernández-Hernando and Cerrato 210 ). Moreover, by preventing LDL oxidation, antioxidants such as vitamin C improve recognition of LDL particles by hepatic LDL receptors and thus expedite their removal from blood( Reference McRae 204 ). In addition, by preventing oxidation of HDL, antioxidants may improve reverse cholesterol transport which is a process that exchanges cholesterol from peripheral tissues and circulating lipoproteins with TAG of HDL, thereby facilitating elimination of cholesterol from blood( Reference Millar, Duclos and Blesso 211 ).
Blood pressure
Meta-analyses of RCT have shown benefits of polyphenols( Reference Serban, Sahebkar and Zanchetti 212 – Reference Ras, Zock and Draijer 215 ) and vitamin C on blood pressure( Reference Juraschek, Guallar and Appel 216 ). Dietary polyphenols have shown a vasoprotective effect by augmentation of endothelial synthesis of NO and endothelium-derived hyperpolarising factor, inhibition of angiotensin-converting enzyme, suppression of endothelin-1 synthesis and increased bioavailability of NO by scavenging free radicals( Reference Medina-Remón, Estruch and Tresserra-Rimbau 217 , Reference Hügel, Jackson and May 218 ). There is also evidence that polyphenols promote vasodilation through an endothelium-independent mechanism by a direct effect on vascular smooth muscle cells via blocking Ca channels( Reference Larson, Symons and Jalili 219 ).
Low total fat
Weight
Although less important than total energy content, the macronutrient composition of the diet is also believed to affect weight. Low-fat diets may have potential in weight control attempts( Reference Peters 220 ); however, meta-analyses of controlled trials suggest that the effect of low-fat diets on weight depends on the diet of the control group( Reference Tobias, Chen and Manson 221 ). As an example, a meta-analysis of RCT indicated that low-fat diets lead to weight reduction only when compared with usual diet, but not in comparison with other dietary compositions such as low-carbohydrate or high-fat interventions( Reference Tobias, Chen and Manson 221 ).
Glucose tolerance
Large-scale cohort studies on healthy populations have shown a positive association between total and saturated fat intake and the development of type 2 diabetes; however, the association disappeared after adjustment for BMI( Reference van Dam, Willett and Rimm 222 ). Nonetheless, individuals in the prediabetic state or those who are genetically vulnerable to metabolic disorders may develop type 2 diabetes following the consumption of high-fat diets( Reference Nagao, Asai and Sugihara 223 ). In fact, the susceptibility of individuals to insulin resistance is affected by genes, and dietary factors can alter this susceptibility( Reference López-Miranda, Pérez-Martínez and Marin 224 ). High-fat diets increase concentration of TAG constituents such as diacylglycerols and ceramides in muscle and adipose tissue. Such compounds phosphorylate serine residues of insulin receptor substrate through activation of serine kinases, leading to an impaired insulin function, decreased translocation of insulin-dependent GLUT-4, and eventually decreased glucose uptake( Reference Galgani, Uauy and Aguirre 225 ). On the other hand, as high-fat diets have low carbohydrate content, they may benefit patients with type 2 diabetes. In this regard, a meta-analysis of RCT suggested that high-fat diets reduce fasting blood glucose in type 2 diabetes patients, but not prediabetic individuals( Reference Schwingshackl and Hoffmann 226 ).
Blood pressure
The quantity of dietary fat has less impact on blood pressure than fatty acid composition of the diet( Reference Hall 227 ). However, an increased concentration of NEFA in blood as occurs following consumption of high-fat meals can impair endothelial function. This postprandial acute effect may potentiate stronger chronic impacts on endothelium if high-fat meals are consumed persistently( Reference Hall 227 ). An increased serum concentration of angiotensin-converting enzyme is proposed as a mechanism of a high-fat diet (45 %) on blood pressure( Reference Schüler, Osterhoff and Frahnow 57 ).
Low saturated fats
Weight
The ratio of saturated:unsaturated fats also affects weight. Saturated fats are probably more obesogenic than unsaturated fats( Reference Krishnan and Cooper 228 ). Compared with saturated fats, unsaturated fatty acids induce greater energy expenditure, diet-induced thermogenesis and fat oxidation( Reference Krishnan and Cooper 228 ). Gene studies revealed that polyunsaturated fats up-regulate expression of PPARα, a transcription factor involved in lipid oxidation, and down-regulate expression of PPARγ, a transcription factor involved in lipogenesis( Reference Georgiadi and Kersten 229 ). Saturated fats have the opposite effect on these genes( Reference Staiger, Staiger and Haas 230 ).
Glucose tolerance
The quality of consumed fats is more important than their quantity for the incidence of diabetes and insulin sensitivity. Saturated fats are associated with an increased risk of type 2 diabetes while unsaturated fats are associated with insulin sensitivity( Reference Galgani, Uauy and Aguirre 225 ). In a multinational study, newly diagnosed diabetes was observed more frequently in patients with higher consumption of total and animal fat and lower plant to animal fat ratio( Reference Thanopoulou, Karamanos and Angelico 231 ). It is worthwhile to note that high quantities of saturated fat (for instance > 15 %) probably increase the risk of insulin resistance and type 2 diabetes, but moderate quantities may not have such an effect( Reference Morio, Fardet and Legrand 232 ).
In diabetes patients, serum cholesteryl esters are mainly composed of SFA. In contrast, a number of studies reported the association of high concentration of unsaturated fatty acids in serum and muscle of healthy individuals and insulin sensitivity( Reference Riccardi, Giacco and Rivellese 233 ). Fatty acids which are received through the diet incorporate into the cell membrane and affect its activity. The ratio of unsaturated fatty acids to SFA present in membrane phospholipids affects membrane fluidity and has a direct relevance to insulin function and glucose transport efficiency( Reference Weijers 234 , Reference Risérus, Willett and Hu 235 ). Apart from the direct effect on insulin sensitivity of peripheral tissues, PUFA also improve hepatic insulin sensitivity through the suppression of lipogenesis and stimulation of fat oxidation( Reference Risérus, Willett and Hu 235 , Reference Georgiadi and Kersten 229 ).
Lipids
Despite the general notion for the atherogenicity of saturated fats, cohort studies do not consistently support the link between saturated fats and CVD. A meta-analysis of prospective studies with follow-up periods of 5 to 23 years showed no significant relationship between dietary saturated fat and risk of CHD or CVD( Reference Siri-Tarino, Sun and Hu 236 ). Another meta-analysis of prospective cohort studies supported a causal relationship between CHD and intake of high-glycaemic index foods and trans-fatty acids and an inverse association between CHD and consumption of vegetables, nuts and MUFA, and healthy dietary patterns, but no association was observed between saturated fats and CHD( Reference Mente, de Koning and Shannon 237 ). The lack of the association of saturated fats with CHD in cohort studies is probably due to substitution of dietary carbohydrates for saturated fats which reduces concentrations of total, LDL- and HDL-cholesterol in a way that total to HDL-cholesterol ratio, an indicator of CVD risk, does not change( Reference Siri-Tarino, Sun and Hu 238 ). In contrast, replacement of saturated fats with unsaturated fats lowers total and LDL-cholesterol, resulting in a decreased total:HDL-cholesterol ratio( Reference Siri-Tarino, Sun and Hu 238 , Reference Schwab, Lauritzen and Tholstrup 239 ). Such substitution has reduced the incidence of cardiovascular events when embedded in an eating pattern for a period of more than 2 years( Reference Hooper, Abdelhamid and Moore 240 ). In this regard, a prospective cohort study with 14 years of follow-up indicated that replacement of 5 % energy from saturated fats with unsaturated fats would reduce the risk of CHD by 42 %( Reference Hu, Stampfer and Manson 241 ). Also, a meta-analysis of RCT suggested that each 5 % energy increase from polyunsaturated fats in place of saturated fats led to a 10 % reduction in CHD( Reference Mozaffarian, Micha and Wallace 242 ). Nevertheless, evidence on the effect of substitution of polyunsaturated fats for saturated fats on risk factors of CVD is still lacking. For instance, insufficient available data did not allow a meta-analysis of RCT to find significant reductions in total cholesterol, LDL-cholesterol and TAG following replacement of unsaturated fats for saturated fats( Reference Hannon, Thompson and An 243 ).
Blood pressure
Studies on the relationship between blood pressure and dietary fats are rather scarce and no meta-analysis has been performed based on the current evidence. The available evidence suggests that saturated fats have unfavourable and unsaturated fats have beneficial impact on blood pressure and vascular function( Reference Hall 227 ). A cross-sectional study revealed that saturated fat intake was independently and strongly associated with hypertension( Reference Beegom and Singh 244 ). Also, in a RCT, substitution of 10 % saturated fats with either mono- or polyunsaturated fats decreased blood pressure and E-selectin without affecting flow-mediated dilation and other measures of vascular function( Reference Vafeiadou, Weech and Altowaijri 56 ). n-3 Fatty acids are the most advantageous fatty acids for blood pressure control. These fatty acids inhibit angiotensin-converting enzyme activity and increase NO bioavailability through augmentation of endothelial NO synthase activity, suppression of pro-inflammatory cytokines and inhibition of cyclo-oxygenase activity( Reference Das 245 ).
Clinical relevance
Based on original reports and meta-analyses, the magnitude of alterations by DASH in risk factors of CVD and the metabolic syndrome is rather small. A meta-analysis by Siervo et al. ( Reference Siervo, Lara and Chowdhury 47 ) indicates that DASH causes small reductions in systolic (–5·2 mmHg) and diastolic (–2·6 mmHg) blood pressure, total cholesterol (–7·73 mg/dl; –0·20 mmol/l) and LDL-cholesterol (–3·87 mg/dl; –0·10 mmol/l)( Reference Siervo, Lara and Chowdhury 47 ). Evidence is also promising for fasting insulin levels (–0·16 mU/l) and fasting blood glucose (–3·42 mg/dl; –0·19 mmol/l), although the effect on glucose has been marginally significant (P=0·07)( Reference Siervo, Lara and Chowdhury 47 , Reference Shirani, Salehi-Abargouei and Azadbakht 54 ). The magnitude of the change in any single risk factor is small and far to be clinically important per se. But the cumulative alterations in these risk factors can produce noticeable effects. For instance, Siervo et al. ( Reference Siervo, Lara and Chowdhury 47 ) predicted that the above-mentioned changes lead to approximately 13 % decrease in the 10-year Framingham risk score for CVD( Reference Siervo, Lara and Chowdhury 47 ). Also, a meta-analysis of cohort studies showed that a DASH-like diet reduces the risk of CVD, CHD, stroke and heart failure by 20, 21, 19 and 29 %, respectively( Reference Salehi-Abargouei, Maghsoudi and Shirani 246 ). On the other hand, the risk reduction in a single individual may be negligible but small risk reductions in individuals in a population become clinically important( Reference D’Agostino, Vasan and Pencina 247 ). Interventions that promote healthy dietary patterns like DASH or the Mediterranean diet can effectively improve health status and reduce the risk of metabolic diseases( Reference Lara, Hobbs and Moynihan 248 ).
Conclusions
As a healthy diet, DASH contains food items and nutrient composition that help in the prevention of metabolic diseases and control of their risk factors. Epidemiological, observational and interventional studies as well as meta-analyses performed have shown benefits of DASH dietary constituents including K, Mg, Ca, fibre and antioxidants and limited content of total fat, saturated fats and Na on components of the metabolic syndrome. Although implementation of each of the DASH dietary guidelines into the diet can help in the prevention of the metabolic syndrome, combination of these instructions augments the benefits. Nonetheless, randomised clinical trials have not examined the effect of DASH on the metabolic syndrome, in some parts the available data for the effect of DASH dietary items on components of the metabolic syndrome are insufficient to allow performing valuable meta-analyses, and mechanisms of the effects are largely unknown. These areas of research call for further investigations in the future.
Acknowledgements
There are no acknowledgements or funding to declare.
There are no conflicts of interest.