Introduction
Infections in free-ranging African wildlife with pathogens normally associated with wild animals or domestic livestock can arbitrarily be assigned to four or possibly even five categories, as follows.
Wildlife-maintained infections: African indigenous infections without disease expression in sylvatic hosts, such as South African Territory (SAT) types of foot and mouth disease (FMD), African theileriosis, African swine fever, African horse sickness, bovine malignant catarrh fever and African trypanosomiasis.
Alien, exotic or foreign animal infections: some of the best examples of this category are certain pathogens historically alien to sub-Saharan Africa that were probably introduced onto the African continent with the importation of domestic livestock from Eurasia during the colonial era. Indigenous African free-ranging mammals, within similar taxonomic groupings to these domesticated hosts, are generally immunologically naïve to these foreign pathogens, and may suffer significant morbidity and mortality when exposed to these disease agents. Examples include rinderpest (may it rest in peace), bovine tuberculosis, bovine brucellosis, peste des petits ruminants (PPR), bovine papillomatosis and canine distemper, which may impact both wildlife and domestic species.
Multi-species infections: these infections usually occur on most continents and may affect both wildlife and domestic livestock. Transmission can thus occur in both directions, although certain regional dominant role players have been identified. These infections are generally cyclical in nature, and the epidemic cycles appear to be related to population densities of one or more host species, as well as climatic factors. Uniquely, these infections generally have a fatal outcome in both wildlife and domestic livestock and are frequently zoonotic. Some of the these diseases, such as anthrax and rabies, have been documented in African buffalo (Syncerus caffer).
Newly emerging or recently detected infections: these infections have been detected only recently, such as encephalomyocarditis in elephants (Loxodonta africana), arthropod-borne flavi- and bunyaviruses in several wildlife species, parafilariasis in African buffalo and feline immunodeficiency virus infection in wild felids. They also include infections that have relatively recently crossed the species barrier, such as canine distemper in lions (Panthera leo) and bovine papillomatosis in giraffe (Giraffa camelopardalis), zebra (Equus zebra), sable antelope (Hippotragus niger) and buffalo.
Finally, truly novel diseases such as spongiform encephalopathies seen in cervids in North America and more recently in Norway and Sweden, but not yet seen in African wildlife.
Infectious Diseases Impacting African Buffalo
Rinderpest
Rinderpest, the great cattle plague pandemic of 1889–1905, was introduced with infected cattle from southern Asia to feed troops on military expeditions around the Horn of Africa (Hutcheon, Reference Hutcheon1902). Much has been written about the massive die-offs of both indigenous cattle, wild ruminants and suids across most regions of Africa, and the massive social, political and economic repercussions resulting from the huge livestock losses (Mack, Reference Mack1970). The livelihoods of nomadic pastoralists were devastated. Countless wild artiodactyls also perished, with buffalo, tragelaphs (spiral horned antelope), wildebeest, hippotragines and wild suids being the most severely affected (Kock, Reference Kock, Barret, Pastoret and Taylor2006). Being social ruminants that occur in large herds, buffalo were massively affected by this close-contact transmitted morbillivirus, and in many cases, only small relict populations survived in remote pockets or were entirely extirpated from their former ranges (Stevenson-Hamilton, Reference Stevenson-Hamilton1957). Impacts were also seen on some keystone species, including migrating East African wildebeest (Connochaetes taurinus). This disease had major impacts on the scale of migration and the habitat, with resultant transformation in vegetation types and distribution (Holdo et al., Reference Holdo, Sinclair and Dobson2009). This disease may have contributed to certain wildlife distribution anomalies, such as the formation of isolated metapopulations of species such as sable antelope, roan antelope (Hippotragus equinus), greater kudu (Tragelaphus strepsiceros) and nyala (Tragelaphus angasii). Rinderpest was declared officially eradicated in 2011, the first animal disease to be so (FAO report, 2011).
Bovine Tuberculosis
Bovine tuberculosis (bTB) in wildlife in sub-Saharan Africa is probably a foreign animal disease introduced into southern Africa with colonial cattle breeds imported from Europe during the eighteenth and nineteenth centuries. The first report of bTB in wildlife in southern Africa was in greater kudu and common duiker (Silvicapra grimmia) in the eastern Cape Province of South Africa (Paine and Martinaglia, Reference Paine and Martinaglia1929), and then again in greater kudu in the same region in 1940 (Thorburn and Thomas, 1940). This region apparently had a high prevalence of bTB in cattle at that time. The first report of bTB in African buffalo came from the Queen Elizabeth National Park (NP) in Uganda (Guilbride et al., Reference Guilbride, Rollinson, McAnulty, Alley and Wells1963), and confirmed in 1965 (Thurlbeck et al., Reference Thurlbeck, Butas, Mankiewicz and Laws1965). The disease spilled over into common warthogs (Phacochoerus africanus) and has persisted to this day (Woodford, Reference Woodford1982a, Reference Woodford1982b). BTB was also discovered in free-ranging Kafue lechwe (Kobus leche kafuensis) in Zambia in 1972 and 1977 (Gallagher et al., Reference Gallagher, Macadam, Sayer and van Lavieren1972; Clancey, Reference Clancey1977), and confirmed later (Munyeme et al., Reference Munyeme, Muma and Siamudaala2010). Subsequent screening of buffalo from the Greater Kafue NP yielded nothing (Munang’andu et al., Reference Munang’andu, Siamudaala and Matandiko2011). In South Africa, the disease was first detected in buffalo in the Hluhluwe/Imfolosi Park in Kwazulu-Natal Province in the 1980s, and in Kruger (KNP) in 1990 (Bengis et al., Reference Bengis, Kriek and Keet1996). It appears as though these wildlife populations became infected by contact with infected cattle. The outbreak in Kruger followed recorded outbreaks of bTB in cattle on farms south of the Crocodile River (southern boundary of KNP) during the early 1960s, and again in the 1980s (State veterinary reports). During these periods, outbreaks of buffalo-associated theileriosis were also reported in cattle on these farms, indicating that buffalo and cattle had shared range and vector ticks. Subsequently in Kruger, the disease spread through all of the buffalo herds from the extreme south to the far north of the park, and eventually into Zimbabwe (Gonarezhou National Park), over a period of 19 years (de Garine-Wichatitsky et al., Reference de Garine-Wichatitsky, Caron and Gomo2010; Figure 9.1). Systematic sampling of buffalo herds in the different regions of the KNP between 2003 and 2011 demonstrated a gradient of the prevalence of infection, with a 35 per cent prevalence in the south where the disease first entered, 20 per cent in the central district and 3 per cent in the far north. Spillover infection also has been detected in 15 other species. Only buffalo and lechwe appear to have become true sylvatic maintenance hosts of bTB, while greater kudu and warthog appear to have maintenance host potential, if their population densities are high enough. The long-term effects of this chronic progressive disease on African wildlife host populations at sustained high prevalence rates are unknown. Initially there was concern that bTB may negatively affect the population dynamics and structure of buffalo herds and lion prides in KNP. To date, however, no negative impact has been detected at the population level in Kruger, illustrating the resilience of these species. However, due to the veterinary control measures imposed, this disease has definitely had an impact on translocation of wildlife out of what are now endemic bTB areas in South Africa. The disease has also been reported in buffalo, warthogs, Uganda kob (Kobus kob thomasi) and olive baboon (Papio anubis) in Uganda, and in buffalo, Masai giraffe (Giraffa camelopardalis tippelskirchi) and topi (Damaliscus lunatus jimela) in the greater Serengeti Ecosystem and in Kenya (Meunier et al., Reference Meunier, Sebulime, Whiteand and Kock2017). These are unfenced systems and the prevalence of disease appears to be relatively stable (Meunier, Reference Meunier2017), although no intensive sampling has been reported. In Ethiopia, although bTB is endemic in cattle, no infection was detected in a small sample of buffalo from Omo/Mago NP, also an open system.

Figure 9.1 Advanced pulmonary tuberculosis in African buffalo.
Brucellosis
In the African wildlife context, only Brucella abortus bovis and B. melitensis have been recorded in wildlife (Simpson et al., Reference Simpson, Thompson and Saegerman2021). Both these organisms have zoonotic potential, although no cases of human infection from buffalo have been reported.
Bovine brucellosis caused by B. abortus bovis is a foreign chronic animal disease thought to have been introduced into sub-Saharan Africa by the importation of European cattle breeds by colonial settlers during the eighteenth and nineteenth centuries. This chronic disease has also crossed the interface with wildlife, and is now endemic in wildlife in several countries. In sub-Saharan Africa, Brucella sero-positivity has been demonstrated in bovids, 12 antelope species, four carnivore species, baboons, black and white rhinoceros, hippopotamus (Hippopotamus amphibius) and zebras. Of all of these, only African buffalo and possibly Kafue lechwe appear to be reservoir species that are able to maintain infection in the absence of infected cattle (Simpson et al., Reference Simpson, Thompson and Saegerman2021).
In KNP, Brucella sero-positivity has been detected in most buffalo herds (De Vos and van Niekerk, Reference De Vos and van Niekerk1969), and at sero-prevalence rates of up to 23 per cent (Herr and Marshall, Reference Herr and Marshall1981). Similar to the disease in cattle, experimental infection with brucellosis causes late-term abortions in buffalo heifers, as well as in buffalo cows during the first pregnancy after infection (Gradwell et al., Reference Gradwell, Schutte, Van Niekerk and Roux1977). In addition, it was observed that calves born through the following pregnancy tend to be weak and poor survivors. Brucellosis in buffalo may also affect synovial structures causing arthritic conditions such as carpal hygromata, and may cause severe lameness (Figure 9.2). This has been observed in both eastern and southern African buffalo populations (Kock, personal communication). In Zimbabwe, clinical disease also has been reported in eland and waterbuck (Condy and Vickers, Reference Condy and Vickers1972). Brucellosis caused by B. melitensis has only been detected in farmed sable antelope on several Eastern Cape wildlife ranches (Glover et al., Reference Glover, Macfarlane and Bengis2020).

Figure 9.2 Brucella Carpel Hygroma in African buffalo.
Anthrax
Anthrax is historically one of the oldest documented diseases, and the life cycle of the causative bacterium, Bacillus anthracis, has both biotic and abiotic components. The abiotic component is the resistant dormant spore phase, which occurs in regions with predominantly alkaline soils and high calcium and moisture content. These spores can survive almost indefinitely in this dormant state in the soil. The biotic component is the exponential amplification phase, which takes place within the mammalian body, and appears to be the essential reproductive phase (De Vos and Turnbull, Reference De Vos, Turnbull, Coetzer and Tustin2004), although germination and amplification of anthrax bacteria in certain soil-dwelling Amoebas has been reported (Dey et al., Reference Dey, Hoffman and Glomski2012). Anthrax outbreaks have been documented in most domestic species in the absence of a wildlife link. Similarly, localized to extensive outbreaks have occurred in various wildlife populations with no livestock link. Large-scale outbreaks may cross the interface, especially where livestock and wildlife share ranges and resources (Mukarati et al., Reference Mukarati, Matope and de Garine-Wichatitsky2020). With regard to buffalo, anthrax has been reported in buffalo populations in KNP, in Gonarezhou NP in Zimbabwe, in the Okavango ecosystem in Botswana, in the Caprivi system in Namibia and in Luangwa valley NP in Zambia. In East Africa, anthrax has been recorded in Uganda, Tanzania, Kenya and Ethiopia. In Kenya, an anthrax outbreak in 2015 at Lake Nakuru NP resulted in the death of 745 out of the 4500 buffalo in the park, with a species-specific mortality rate of 17 per cent (Muturi et al., Reference Muturi, Gachohi and Mwatondo2018). An analysis of temporal and spatial distribution of anthrax outbreaks among Kenya wildlife revealed that out of the 51 outbreaks, 23.5 per cent involved buffalo (Gachohi et al., Reference Gachohi, Gakuya and Lekolool2019).
In Mago NP in Ethiopia, anthrax outbreaks originating from cattle and sheep from nearby pastoralists killed more than 1617 (in 1999) and 563 (in 2000) wild animals from 21 species, including buffalo. About 20 people, including one scout, from the area who handled or ate the carcasses, including one scout, were infected and developed severe skin lesions, but recovered after treatment (Shiferaw et al., Reference Shiferaw, Abditcho, Gopilo and Laurenson2002).
In a review of seven documented anthrax outbreaks in KNP between 1960 and 2010, buffalo featured as the second most common species affected in an analysis of the carcass counts. Greater kudu were the most frequently affected species in these Kruger outbreaks (Bengis, personal communication; Figure 9.3).
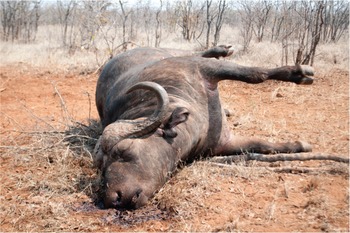
Figure 9.3 Anthrax in African buffalo. Note blood coming from nose and generalized putrefactive swelling.
In Africa, human anthrax is generally contracted when people handle or consume carcasses of infected livestock or of any wildlife that have succumbed during an outbreak, and this would include buffalo in light of the susceptibility of this species as mentioned.
Buffalo appear to become infected mainly by ingestion of contaminated water, contaminated grazing or by mechanical transmission by biting flies. Buffalo are exquisitely sensitive to the exotoxins produced during the exponential replication phase of the anthrax organisms, and unlike other ruminants, will often die before developing substantial bacteremia. This makes it important for laboratories to microscopically scan buffalo diagnostic blood smears thoroughly if this disease is suspected. The post-mortem signs commonly seen with buffalo that have died of anthrax include sudden death, tarry black blood that does not clot exuding from the natural orifices and swollen carcasses from rapid putrefaction. Suspected anthrax carcasses should not be opened.
Other Bacterial Diseases
There are several other bacterial diseases that have been sporadically reported in African buffalo. Localized bacterial abscesses caused by a variety of organisms do occur, usually as a result of bacterial infection of penetrating wounds. With the exception of anthrax, bacterial infections that cause systemic disease have rarely been reported in free-ranging buffalo. Those that have been detected include haemorrhagic septicaemia caused by Pasteurella multocida and bacterial entero-colitis caused by entero-pathogenic Escherichia coli or Salmonella spp. (Mitchell et al., Reference Mitchell, Steyl and Woodburn2021). In addition, malignant oedema/gas gangrene, caused by toxigenic Clostridia, have been sporadically reported following blunt traumatic injury and bruising.
Buffalo and Other Wildlife-Maintained Infections
In this discussion, it is important to realize that only a few wildlife species have been identified and positively implicated in the maintenance and transmission of these diseases. These include the African savanna buffalo, wild suids including warthogs and bush pigs, brindled wildebeest, black wildebeest (Connochaetes gnou), bushbuck, the various species of zebra and possibly greater kudu.
Foot-and-Mouth Disease
In the case of FMD in sub-Saharan Africa, cattle-derived imported Eurasian serotypes A and O coexist with African serotypes SAT1, 2 and 3 (SAT for South African Territory). The pivotal role played by the African buffalo as a sylvatic maintenance host of the SAT group of FMD viruses was identified in the late 1960s, and initial findings in various buffalo populations were published by Condy (Reference Condy1971), Hedger (Reference Hedger1972) and Falconer and Child (Reference Falconer and Child1975). This was followed by further publications on the characterization of the viruses in several buffalo subpopulations in eastern and southern Africa (Anderson et al., Reference Anderson, Doughty, Anderson and Paling1979; Vosloo et al., Reference Vosloo, Bastos and Kirkbride1996; Thomson and Bastos, Reference Thomson, Bastos, Coetzer and Tustin2004). Most buffalo populations in southern Africa, with the exception of those relict populations that survived the rinderpest in the Eastern Cape and Kwazulu/Natal Provinces of South Africa, have been shown to be infected. Random serological sampling of buffalo herds in KNP in South Africa, the Okavango delta in Botswana and the Zambezi valley in Zimbabwe demonstrate that over 95 per cent of those free-ranging buffalo have antibodies to at least one, but frequently two or even all three SAT virus serotypes, by the age of 12 months. Surveys in West, Central and East Africa showed a similar picture (Bronsvoort et al., Reference Bronsvoort, Parida and McFarland2008). In addition, FMD viruses of all three serotypes can regularly be isolated from oro-pharyngeal probang samples collected from these buffalo, and this carrier state may last for years (Jolle et al., Reference Jolle, Gorsich and Gubbins2021). The virus apparently survives almost indefinitely in certain dendritic cells of the tonsils, even in the presence of humoral antibodies. Most free-ranging buffalo show no clinical signs of infection.
Thus, until recently, there could be no doubt that infected buffalo herds were the ultimate source of all FMD infections in naive livestock, and most SAT-type FMD outbreaks in livestock could also be traced back to interface buffalo contact (Guerrini et al., Reference Guerrini, Pfukenyi and Etter2019). However, more recently, it would appear that certain SAT virus topotypes have adapted to cattle, and several outbreaks have recently occurred in South Africa without any identifiable buffalo contact (Thomson, personal communication). This has been confirmed in Kenya (Wekesa et al., Reference Wekesa, Sangula and Belsham2015). They found that FMDV serotypes O, A, SAT1 and SA2 were circulating among cattle in Kenya and cause disease, but only SAT1 and SAT2 viruses were successfully isolated from clinically normal buffalo. The buffalo isolates were also genetically distinct from isolates obtained from cattle. In another study in Kenya, Omondi et al. (Reference Omondi, Gakuya and Arzt2020) investigated the epidemiology of FMDV in buffalo, including the role of buffalo in the circulation of FMDV in livestock populations. By sequencing the virus’ VP1 coding region from blood and oropharyngeal fluids collected from wild buffalo and sympatric cattle in central Kenya, they were able to show that FMDV has a high seroprevalence in buffalo and targeted cattle populations. In addition, serotype SAT1 and SAT2 sequences from buffalo and serotype O and A sequences from sympatric cattle were recovered. These results further confirmed the findings of Wekesa et al. (Reference Wekesa, Sangula and Belsham2015), because amongt sympatric buffalo and cattle, no SAT1 or 2 sequences found in buffalo were found in cattle, which suggests that transmission of FMDV from buffalo to sympatric cattle in this region is rare. Similarly, there was no evidence that serotype O and A sequences found in cattle were transmitted to buffalo. Serotypes A and O have also been introduced with infected cattle into Ethiopia and other countries in the region. In Ethiopia, serology confirmed the presence of antibodies to serotypes A, SAT1 and SAT2 in clinically normal buffalo in Mago/Omo NP, whereas in neighbouring cattle, which have a seropositivity prevalence of between 5.6 per cent and 42.7 per cent with endemic distributions, serotypes O, A, SAT1 and SAT2 were documented (Sahle, Reference Sahle2004; Abdela, Reference Abdela2017). More recently (2021), Eurasian serotype O has been reported in cattle in Zimbabwe, Namibia and Mozambique.
FMD is rarely a fatal disease in cattle except for occasional calf mortalities, but it certainly does impact animal production, as dairy cows dry up and beef cattle lose condition. It is therefore not generally considered to be an important disease among pastoralists and subsistence farmers. However, due to FMD international control regulations (through the WOAH (World Organization for Animal Health, founded as the OIE (Office International of Epizootics)) directed by northern hemisphere countries, subsistence farmers living close to buffalo populations in Africa have been negatively impacted. Fortunately, in recent years there has been an increasing realization among world leaders that globalization is perpetuating the dominance of the developed world, and there is a genuine desire to address poverty issues in Africa (Ferguson et al., Reference Ferguson, Cleaveland and Haydon2013; Thomson et al., Reference Thomson, Penrith and Atkinson2013a, Reference Thomson, Penrith and Atkinson2013b; Chapter 12).
Buffalo-Associated Theileriosis
Theileria parva is a tick vector-borne protozoan infection of buffalo. It is transmitted by Rhipicephalus appendiculatus and R. zambesiensis, which are three-host ticks. It is naturally a silent infection in buffalo, but is highly pathogenic and causes an acute fatal disease in cattle, especially the European cattle breeds (Neitz et al., Reference Neitz, Canham and Kluge1955). It is generally a dead-end disease in these cattle, because they die before the lymphoid tissue-associated schizonts can produce erythrocyte-associated merozoites (small piroplasms), and are therefore unable to infect the vector ticks to maintain circulation of the infection. Small clustered outbreaks of buffalo-associated theileriosis still occur today in southern and East Africa where there is a buffalo/cattle interface. However, cattle-adapted strains have now also evolved, which cause the diseases known as East Coast fever (ECF) and Zimbabwe theileriosis (January disease). These diseases can circulate independently in cattle without any buffalo presence because these Theileria parva variants can complete their life cycle in cattle, produce small piroplasms, and are therefore able to infect the tick vectors (Irvin and Cunningham, Reference Irvin, Cunningham, Ristic and McIntyre1981). ECF occurs widely through the range of the same main vectors (R. appendiculatus and R. zambesiensis) in East and southern Africa north of the Zambezi River. In these regions, ECF, together with heartwater and trypanosomiasis, remain the greatest disease obstacle to agricultural development and prosperity. However, T. parva is absent in West Africa and most of Central Africa, including the whole Congo basin, because R. appendiculatus is absent. In Central Africa, the disease is only present in the far eastern side, above 500 m in the region of the Great Lakes: Burundi, Rwanda, Kivu in eastern DRC. This is a disease of great concern to most pastoralists in the affected countries because it has the potential to threaten food security. ECF was also introduced with cattle movement to regions south of the Zambezi River in 1901/02, but after resulting in the death of 1.25 million of the 4 million cattle present in the affected territories, was subsequently eradicated at great expense to governments and both farmers and pastoralists. From an agriculturalist/pastoralist perspective, this was a disaster, but for conservation and environmental objectives, these diseases have ensured that large tracts of land in Africa remain ecologically intact and viable, a vital element in the desired long-term recovery from anthropogenic impacts that are of such contemporary global concern. These diseases were eventually eradicated from southern Mozambique in 1917, from Rhodesia in 1954 and from South Africa in 1955 (Lawrence, Reference Lawrence, Norval, Perry and Young1992), but continue to circulate in cattle north of the Zambezi River.
There are other theilerias frequently found in buffalo blood samples, such as T. mutans (transmitted by Amblyomma spp.) and T. taurotragi (transmitted by R. appendiculatus). Both of these parasites generally cause benign infection in cattle. Buffalo-associated T. taurotragi also generally results in a mild infection in cattle, but occasionally causes a disease called turning sickness in young cattle, characterized by nervous signs, such as circling, head pressing, ataxia, paralysis and uni- or bilateral blindness (Lawrence and Williamson, Reference Lawrence, Williamson, Coetzer and Tustin2004).
Trypanosomiasis
Trypanosomiasis is a very important disease of cattle (known as nagana), camels (known as surra), horses, pigs and dogs in parts of sub-Saharan Africa, and certain Trypanosoma species may also affect humans (causing sleeping sickness disease). The various Trypanosoma spp. have different transmission modes, including biological transmission by tsetse flies, an insect genus endemic to Africa that had time to co-evolve with buffalo (T. brucei and T. congolense, T. vivax), and mechanical transmission by other biting flies of three main families, Tabanidae (genera Chryspos, Haematopota and Tabanus), Stomoxynae (genera Stomoxys and Haematobia) and Hippoboscidae (genera Melophagus and Hippobosca) (all previous plus T. evansi) (Itard, Reference Itard1981). With non-tsetse biting flies, trypanosomiasis expands well beyond the tsetse fly-infested areas.
Historically, the distribution of tsetse flies (known as tsetse belts) profoundly shaped and limited the distribution of livestock, and consequently severely hampered the development of the livestock industry. However, the situation has changed dramatically in the last decades under pressure from two main driving forces: (i) the drastic reduction of the tsetse distribution range, due notably to the destruction of their habitat such as forest galleries for agriculture (Gouteux et al., Reference Gouteux, Blanc and Pounekrozou1994; Cuisance, Reference Cuisance1996; Reid et al., Reference Reid, Russell and Kruska2000), tsetse eradication campaigns, the global climate change (Courtin et al., Reference Courtin, Rayaissé and Tamboura2010) and for savanna tsetse species such as Glossina morsitans, the disappearance of large game (Itard, Reference Itard1981); and (ii) the development and now widespread use of trypanocide drugs, both preventive and curative, which allow large transhumant cattle herds to graze in tsetse-infested areas during the dry season, including in Protected Areas, and leads to the degradation of the pastoral rangeland though excessive bushfire, overgrazing, tree felling, etc.
Preferred wildlife hosts of tsetse flies include African buffalo, wild porcines, spiral horned antelopes (Tragelaphs spp.), elephants, black rhinoceros and hippopotami, and these species are all capable of surviving well within the tsetse belts. They frequently develop significant infection rates with detectable parasitaemias, but without developing disease, and may therefore serve as natural maintenance hosts for the various Trypanosome spp. Interestingly, the respective historical distribution ranges of African buffalo and tsetse flies largely match (Olubayo, Reference Olubayo1991). Buffalo and tsetse flies have co-evolved in the same ecological system for millenaries to the mutual advantage of the three stakeholders, the parasite, its vector and its host, with the consequence of having made the buffalo both a maintenance host and one resistant to the parasite. The mechanisms of trypano-resistance of the African buffalo have been partially investigated (e.g. Olubayo, Reference Olubayo1991; Redruth et al., Reference Redruth, Grootenhuis and Olubayo1994; Wang et al., Reference Wang, Hamilton and Black2000; Guirnalda et al., Reference Guirnalda, Murphy, Nolan and Black2007).
Trypano-tolerant cattle breeds, all of them humpless Bos taurus (e.g. N’dama and Baoulé cattle), as well as trypano-tolerant goat and sheep breeds, have evolved in pastoral societies, mainly in West Africa, but also in Central and East Africa (Murray et al., Reference Murray, Morrison and Murray1979). They are, however, not trypano-resistant and may potentially serve as maintenance hosts.
An important conservation corollary is that many of the remaining and relatively pristine wildlife conservation areas in Africa owe their very existence to the presence of this disease, which made these areas unsuitable for agricultural expansion or human habitation (Chapter 12). However, as seen above, the situation is changing drastically with the wide use of trypanocide drugs. Pastoralists no longer hesitate to enter deep inside tsetse-infested Protected Areas with large cattle herds. To quote only one striking example from Cameroon, before trypanocid drugs no livestock entered Bouba Ndjidda NP and the seven surrounding Hunting Areas due to heavy tsetse infestation, while in 2015 the wildlife aerial census of these Protected Areas counted 526,233 (over half a million) head of cattle (Wildlife Conservation Society, Ministry of Forests and Wildlife and Ecole de Faune de Garoua, 2015), which was 21 times more than in 2008 (Omondi et al., Reference Omondi, Bitok and Tchamba2008), despite the continued presence of tsetse flies there today.
With the contemporary shrinkage of the distribution range of tsetse flies, their main remaining strongholds are now largely Protected Area complexes, which also are the residual strongholds of the African buffalo. In these restricted situations, the coevolution of the tryptic ‘buffalo/tsetse/trypanosome’ is maintained, including the resistance of buffalo to the disease, and buffalo remain there as a maintenance host and maybe even a bridge host. The question is raised whether these Protected Areas can now be regarded as maintenance sites, including for Human African Trypanosomiasis (HAT). HAT originating from wildlife has been explored to some extent, but only in a very limited number of wild species like bushbuck (Heisch et al., Reference Heisch, McMahon and Manson-Bahr1958). Much remains to be investigated with regards to the possible role of wildlife, including buffalo, in the maintenance of Trypanosoma brucei gambiense, which is responsible for HAT in West and Central Africa.
Peste des petits ruminants
The peste des petits ruminants (PPR) virus is closely related to the rinderpest virus (serologically indistinguishable with screening enzyme-linked immunosorbent assays, or ELISAs), from which it likely evolved (rinderpest of small ruminants) over recent centuries in West Africa. It spread to much of the rest of Africa and Asia, and this pandemic has gathered pace over the last two decades, entering East Africa and spreading south and across Asia, reaching the China seaboard in 2013. Serological surveys of wildlife, including mostly buffalo, during the rinderpest eradication campaign showed cross neutralization between rinderpest and PPR antibodies, and ELISA tests during epidemio-surveillance produced confusing results. Once differentiated, this confirmed widespread infection of wild artiodactyls, buffalo included, in Africa with the PPR virus where livestock were infected, and evidence suggests the small ruminants spilled the virus into adjacent wildlife populations (Kock, Reference Kock, Barret, Pastoret and Taylor2006; Mahapatra et al., Reference Mahapatra, Sayalel and Muniraju2015; Fernandez Aguilar et al., Reference Fernandes Aguilar, Mahapatra and Begovoeva2020). Buffalo were no exception and have shown the highest true prevalence for PPR virus infection among sampled species (Jones et al., Reference Jones, Mahapatra and Keyyu2021). No evidence of disease in African buffalo is reported, while only rare and, epidemiologically unconfirmed, reports of PPR disease have been made in sub-Saharan Africa in free-ranging antelope, notably gazelle in Sudan (Asil et al., Reference Asil, Ludlow and Ballal2019).
Malignant Catarrhal Fever
In Africa, the most important cause of malignant catarrhal fever (MCF) in cattle in the context of this chapter is wildebeest-associated MCF caused by Alcelaphine Herpesvirus 1. There is also a sheep-associated MCF affecting cattle caused by Ovine Herpesvirus 2. The important role played by wildebeest (Connachaetes spp.) in the maintenance and seasonal shedding of Alcelaphine Herpesvirus 1 has been elucidated (Plowright et al., Reference Plowright, Ferris and Scott1960; Plowright, Reference Plowright1967; Mushi et al. Reference Mushi, Karstad and Jesset1980). Free-ranging African buffalo in multi-species systems may be infected with this virus and sero-convert, but do not develop overt clinical signs of disease, or detected viral persistence, and thus appear totally unimportant in the maintenance or transmission of this important viral disease of cattle. West and Central Africa are outside the wildebeest distribution range and MCF infection has not been reported in buffalo there.
Rabies
Rabies is an ancient disease, and recognizable descriptions of it can be traced back to early Chinese, Egyptian, Greek and Roman records (Wilkinson, Reference Wilkinson, Campbell and Charlton1988). In sub-Saharan Africa, sylvatic rabies has been diagnosed in 33 carnivorous species and 23 herbivorous species, including African buffalo (Mitchell et al., Reference Mitchell, Steyl and Woodburn2021), with regional variation in dominant epidemiological role players (Swanepoel, Reference Swanepoel, Coetzer and Tustin2004). In spite of this, by far the largest number of rabies cases reported in the developing world occur in domestic dogs. In Africa, endemic rabies (caused by both viverid and canid biotypes) has been identified in certain communal burrow-dwelling wildlife species such as the yellow mongoose, and in bat-eared fox as well as various jackal species. African buffalo appear to be incidentally infected and do not appear to play any role in the maintenance of this infection.
Buffalo Involvement in Other Important Livestock Diseases
Tick-borne Diseases
Heartwater (Cowdriasis, Ehrlichia/Rickettsia ruminantium Infection)
Heartwater is a tick-transmitted rickettsial infection, and is one of the most important diseases of domesticated ruminants in sub-Saharan Africa. This disease causes high morbidity and significant mortality in cattle (especially Bos taurus types), as well as in sheep and goats, throughout the range of its biological vectors, which are present in most of sub-Saharan Africa with the exception of the extremely arid zones. The important biological vectors are Ixodid ticks of the genus Amblyomma, which are three-host ticks. Interestingly, in West and Central Africa, the historical distribution area of buffalo largely matches with the range of A. variegatum (annual rainfall over 500 mm). Some Amblyomma are specific to buffalo and suspected to be vectors, namely A. splendidum in forest buffalo (Syncerus caffer nanus), A. astrion in Central African savanna buffalo (S. c. aequinoctialis), A. cohaerens in East African buffalo and A. hebraeum in Southern African buffalo (Morel, Reference Morel1981).
Free-ranging African buffalo, together with giraffe, black wildebeest, blesbok and eland that occur within the distribution range of the Amblyomma ticks, frequently harbour this rickettsial organism without developing the disease. The infection in these species is generally subclinical due to the evolution of disease resistance over millennia and the development of an endemically stable host/pathogen relationship. Helmeted guineafowl, leopard tortoises and scrub hares, which are the preferred hosts of the larval and nymph stages of this vector, may also harbour this pathogen (Oberem and Bezuidenhout, Reference Oberem and Bezuidenhout1987). All of these silent carriers may potentially serve as sources of infection for the vector ticks, which theoretically could infect livestock in an open interface situation where livestock and wildlife share range and resources. However, it appears from recorded spatial and temporal patterns of disease outbreaks that the major source of infection for the vectors causing most livestock outbreaks are in fact the domestic livestock themselves, and this perpetuates the cycle of infection and disease.
Anaplasmosis
Both Anaplasma marginale subsp. centrale and A. marginale are regularly found in buffalo blood samples. Eygelaar et al. (Reference Eygelaar, Jori and Mokopaseto2015) report prevalence rates of 30 per cent for A. marginale subsp. centrale and 20 per cent for A. marginale in free-ranging buffalo in northern Botswana. These pathogens can be transmitted by several one-host and multi-host ticks of the genus Rhipicephalus; they can also be mechanically transmitted by biting insects, mainly by biting flies of the families Tabanidae and Stomoxynae, less so by mosquitoes (Morel, Reference Morel1981). A. marginale is pathogenic in cattle, causing severe anaemia and icterus, mainly in older dairy and beef cattle of the Bos taurus type. This disease is commonly known as ‘dry gall sickness’. In endemic areas, most calves are generally immune. A. marginale subsp. centrale infection is usually apathogenic in cattle, and is in fact used in a blood-based vaccine for cattle. It appears that buffalo have become incidentally infected by ticks that have fed on infected cattle and play a minor role, if any, in the maintenance of this disease. Buffalo do not develop any clinical signs of infection.
Babesiosis
The babesioses are tick-borne infections caused by intra-erythrocytic protozoal parasites of the genus Babesia. Four species are known to infect cattle in southern Africa, namely B. bovis, B. bigemina, B. occultans and an as yet unnamed species. B. bovis and B. bigemina are highly pathogenic and both cause redwater disease in cattle, but they have never been documented in African buffalo. Other pathogenic Babesia occur in sheep, goat, pigs and equids. Therefore, buffalo do not appear to play any role in the epidemiology of these two important cattle diseases. However, B. occultans, which causes benign infections in cattle, is also frequently found in buffalo, and a prevalence of 23 per cent has been reported in 120 buffalo blood samples collected in Northern Botswana (Eygelaar et al., Reference Eygelaar, Jori and Mokopaseto2015). The vector of B. occultans is Hyalomma marginatum rufipes, a two-host tick with a wide distribution range in southern Africa.
African Insect-Borne (Arbo-) Virus Diseases
Serological studies have shown that a whole host of wild artiodactyls, including African buffalo, are able to be infected with several of these insect-borne viruses, but the infections are naturally subclinical, indicating innate resistance or low pathogenicity in these wildlife species. These diseases include lumpy skin disease (LSD), caused by a capripox virus, bluetongue and epizootic haemorrhagic disease caused by orbiviruses, bovine ephemeral fever caused by a rhabdovirus, Rift Valley fever (RVF) caused by a phlebovirus and congenital arthrogryphosis/hydrancephaly caused by Akabane virus. In addition, some recent investigations have looked into the involvement of buffalo in certain Flavivirus and Bunyavirus infections.
Lumpy Skin Disease
Davies (Reference Davies1991) reported the detection of antibodies to LSD in buffalo in an endemic LSD area, but no clinical disease. In South Africa, sero-surveys of 440 free-ranging buffalo from KNP were all negative for serum-virus neutralizing antibody. In addition, experimental infection of sero-negative buffalo gave negative results (Howell and Coetzer, unpublished results). These animals did not even sero-convert. From these observations, it can be concluded that African buffalo are not susceptible to this virus and play no role in the epidemiology of this important disease of cattle.
Bluetongue
Serum-virus neutralizing antibodies have been detected in African buffalo, as well as many other wild sympatric ruminants (Davies and Walker, Reference Davies and Walker1974). However, clinical disease has not been reported, and the role of these wild ruminants in the epidemiology of this livestock disease remains speculative.
Ephemeral Fever
Also known as three-day stiff sickness, ephemeral fever occurs in most sub-Saharan countries in the form of epizootics in cattle. In between these epizootics, limited foci of disease may be encountered and there is evidence of sero-conversion in sentinel herds. The examination of a range of wild ruminant sera showed evidence of neutralizing antibody in 54 per cent of buffalo, 62 per cent of waterbuck (Kobus ellipsiprymnus), 9 per cent of wildebeest and 2.8 per cent of hartebeest (Alcelaphus buselaphus) (Davies et al., Reference Davies, Shaw and Ochieng1975). An interesting observation is that there were sero-conversions in waterbuck and buffalo in samples collected before the previous cattle epizootic of the disease. This would appear to indicate that the virus was circulating in wild ruminant populations during a period when no clinical disease was observed in cattle. Thus wild ruminants, including buffalo, may play a maintenance role during inter-epizootic periods.
Rift Valley Fever
Rift Valley fever is a zoonotic mosquito-borne virus disease of livestock and wild ruminants that has been identified as a risk for international spread. Typically, the disease occurs in geographically limited outbreaks associated with high rainfall events, and can cause massive losses of livestock. It is unclear how the RVF virus persists during interepidemic periods, but cryptic low-level cycling of the virus in livestock and/or wildlife populations may play a role. What is known is that the RVF virus can be efficiently maintained by certain floodwater breeding Aedine mosquitoes. In these mosquitoes, male/female sexual transmission as well as transovarial transmission of virus occurs. The eggs are laid on grasses, sedges and mud on the edge of rainwater pans, and these eggs are dormant and require a drying-out period followed by re-wetting to hatch. The time course for this drying out and re-wetting follows climatic cycles and may be weeks, months, years or even decades, and this is probably the main maintenance mechanism during the interepidemic periods (Linthicum et al., Reference Linthicum, Davies, Bailey and Kairo1984, Reference Linthicum, Davies, Kairo and Bailey1985).
In 1999, an abortion storm caused by the RVF virus occurred at a disease-free buffalo breeding project in KNP, followed by a second outbreak at another buffalo breeding project just south of the park. Serological surveys in free-ranging buffalo in KNP revealed generally low levels of sero-positivity that spiked during these outbreaks (Beechler et al., Reference Beechler, Bengis and Swanepoel2015). In addition, several other aborted buffalo foetuses were also positive for RVF (Mitchell et al., Reference Mitchell, Steyl and Woodburn2021). Sero-positivity also has been detected in a range of other wild ungulates in South Africa (Swanepoel, Reference Swanepoel1976) and Zimbabwe (Caron et al., Reference Caron, Miguel and Gomo2013). In Kenya, a sero-prevalence of around 15 per cent of RVF virus neutralizing antibodies has been detected in buffalo (Evans et al., Reference Evans, Gakuya and Paweska2008; Britch et al., Reference Britch, Binepal and Ruder2013). Many buffalo live in endemic RVF areas, and this could explain the seroconversion to this mosquito-borne disease. It is notable that other wildlife such as gazelles living outside these endemic regions can suffer clinical disease during periods of epizootic expansion, although this has not been observed in sympatric buffalo. It also has been observed that significant mortality from RVF occurred when naive scimitar-horned oryx (Oryx damma) were translocated into the RVF endemic area of Chad from the UAE, whereas the long-term resident oryx appeared resistant (Chardonnet, personal communication).
In 2010, during a major outbreak of RVF in the Orange Free State and Northern Cape Provinces in South Africa, apart from heavy livestock losses, RVF-associated abortions and mortality were recorded in ranched buffalo, eland (Taurotragus oryx), sable antelope, waterbuck, springbok (Antidorcas marsupialis), blesbok (Damaliscus dorcas phillipsi) and bontebok (D. d. dorcas), as well as exotic fallow deer, llamas and alpacas. Whether buffalo or any of these other species play a role in low-level maintenance cycling of the RVF virus during the interepizootic period remains speculative.
Mycoplasma
Contagious bovine pleuropneumonia (CBPP), caused by Mycoplasma mycoides subsp. mycoides, is one the three great historic cattle plagues of the world (OIE, 2022a), and Contagious caprine pleuropneumonia (CCPP), caused by M. capricolum subsp. capripneumoniae, is one of the most severe diseases of goats (OIE, 2022b). Moreover, these two diseases have gained renewed attention since the eradication of rinderpest and the PPR eradication programme. The African buffalo is not susceptible to CBPP and does not play any role in its transmission. In contrast with CBPP, which affects cattle only, CCPP also affects a number of wild ungulates, including some African antelopes; however, it is not known to affect the African buffalo. It is difficult to evaluate the prevalence of these mycoplasma diseases in wildlife due to sampling and transportation constraints. However, the use of specific serological tests and PCR may improve information in wildlife.
Emerging Infectious Diseases
So-called emerging animal infectious diseases include recently detected diseases (often in new geographies), variants of known diseases, diseases that have recently crossed the ‘species barrier’ and finally, truly novel diseases. With regard to African buffalo, the following emerging infectious diseases have been reported.
Bovine Papillomatosis
Infection with bovine papilloma virus types 1 or 2, causing cutaneous lesions in giraffe, Cape mountain zebra (Equus zebra zebra), sable antelope and African buffalo, have been described and confirmed by histopathology and immunohistochemistry. These cutaneous lesions varied from single or multiple wart-like growths to massive sarcoids (Williams et al., Reference Williams, van Dyk and Nel2011; Figure 9.4). This is a good example of a cattle disease that has crossed the species barrier.

Figure 9.4 Papillomatosis on an African buffalo.
Diseases Caused by Akabane and Related Simbu-Group Viruses
In KNP, several buffalo calves with arthrogryposis and hydranencephaly were born at a buffalo breeding facility. These congenital defects are frequently a result of in utero infection of the foetus with Akabane virus during certain critical stages of development. There is no reason to suspect that these congenital deformities do not also occur in free-ranging buffalo, but are rarely seen because most new-born animals with congenital defects are taken out by predators. In a sero-survey for Akabane virus infection in African wildlife, neutralizing antibody was detected in 222/979 buffalo (22.7 per cent; Al-Busaidy et al., Reference Al-Busaidy, Hamblin and Taylor1987).
In the past decade, mortalities associated with neurological clinical signs have been reported in several free-ranging wildlife species, including a variety of antelope, warthogs, white rhinoceros and African buffalo. Histopathology demonstrated a viral encephalitis characterized by glial apoptosis, neuronal degeneration and/or cerebral oedema (Mitchell et al., Reference Mitchell, Steyl and Woodburn2021). A Shuni virus of the Simbu group has been implicated.
Diseases Caused by Flaviviruses
Bovine viral diarrhoea virus (BVDV) and antibody have been detected in a whole range of free-ranging ruminants (Hamblin and Hedger, Reference Hamblin and Hedger1979), and in Africa, some buffalo and wildebeest populations suffer high infection rates (Hyera et al., Reference Hyera, Liess, Anderson and Hirji1992). In a recent report (Mitchell et al., Reference Mitchell, Steyl and Woodburn2021), two aborted buffalo foetuses and one neonatal mortality were confirmed to be caused by BVDV, using immunohistochemistry.
Macroparasitic Infections in African Buffalo
When reading this paragraph, what needs to be appreciated is that all free-ranging wildlife are ‘biological packages’ that are infected subclinically by a variety of endo- and ectoparasites. African buffalo are no exception, and host numerous different nematodes, cestode and trematode worms and several external parasites (Boomker et al., Reference Boomker, Horak, Penzhorn, Keet and Penzhorn1996). What is important is that most of these parasites do not appear to deleteriously affect the health of free-ranging wildlife in any way. In free-ranging multi-species wildlife systems, certain mammalian species may be susceptible to certain parasitic infections, and the uptake of larvae or ova by non-patent species functions as a natural balancing mechanism that reduces infection burden and helps to reduce parasite loads resulting in sustainable host/parasite relationships. In addition, in the co-infection context in which most wild species live, multiple minor parasitic infections may result in parasitic competition or in immune stimulation, both of which attenuate parasitic pathogenesis and minimize the effect on the host health (Chapter 11).
It is beyond the scope of this chapter to attempt to tabulate and describe all of the macro-parasites that have been detected in African buffalo, and we will limit our discussion to include mainly those parasitic infections of buffalo that are overtly visible in the live animal or in the dead animal carcass.
Ixodid Ticks
Most free-ranging wildlife are parasitized to a greater or lesser extent by one or more of the life-cycle stages of ixodid ticks. Certain species, such as African buffalo, giraffe and black and white rhinos, are preferred hosts for the adult stage of several of these ixodid ticks, which is the reproductive stage of these ticks. Adult ticks of the important genera Amblyomma, Rhipicephalus and Hyalomma are frequently found on these host species. In a study conducted in Ethiopia’s Mago/Omo National Park (Shiferaw and Kock, Reference Shiferaw and Kock2002), seven species of ticks, namely Amblyomma cohaerence, A. lepidum, A. variegatum, A. nuttali, A. gemma, Rhipicephalus pravus, R. pulchaellus and R. evertsi, were collected from buffalo. In southern Africa, A. hebraeum, R. appendiculatus and R. zambeziensis are the most common buffalo ticks. In Central African Republic, A. variegatum, A. astrion, R. longus, R. muhsamae, R. cliffordi, R. lunatus, Hyalomma nitidus and H. rufipes have been described on 100 per cent of 85 examined buffalo (Thal, Reference Thal1971).
Parafilaria bassoni Infection
Parafilaria bassoni is a spirurid nematode that infects the skin and subcutaneous tissues of buffalo, causing bleeding skin nodules (Figure 9.5). These bleeding nodules are associated with gravid female worms ovipositing embryonated eggs. These lesions occur mainly on the dorsum and lateral sides of the buffalo. Complications of these lesions develop in a low percentage of buffalo due to secondary bacterial infections forming subcutaneous abscesses, or as a consequence of a Type 1 hypersensitivity reaction that may result in vascular occlusion, and skin infarction with the development of cutaneous ulcers, which often become enlarged by oxpecker worry. A sero-survey using a Parafilaria ELISA in the Greater KNP Complex demonstrated that this parasite occurs in buffalo populations throughout this complex at a seroprevalence rate of approximately 34 per cent (Keet et al., Reference Keet, Boomker and Kriek1997).
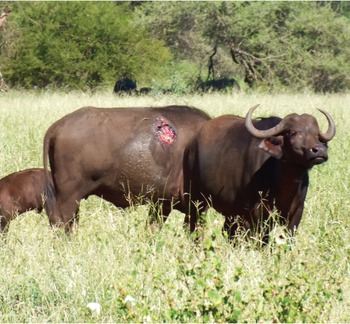
Figure 9.5 Parafilaria skin ulceration on an African buffalo.
Psoroptic Mange
Psoroptic mange is caused by a large sarcoptiform mite, Psoroptes pienaari, commonly found on buffalo. This is a large mite that can be seen with the naked eye. This mite is most commonly found on the head, neck, shoulders and rump of buffalo, and causes a pruritic scaley alopecia (hair loss), frequently giving the skin a thickened, hairless, greyish appearance.
Demodectic Mange
Demodectic mange is caused by the parasitic mite Demodex caffer, which inhabits the hair follicles in the skin of buffalo, and when present in large numbers may cause nodular parasitic and sebaceous cysts (Figure 9.6). These cutaneous skin nodules are most commonly seen in the younger age classes, and are generally limited to the head, neck and shoulder regions, but in severe cases, may be present over the entire animal (Dräger and Paine, Reference Dräger and Paine1980). If one of these nodules is incised and lateral pressure applied, a thick, creamy white material exudes, which consists of sebaceous cells and masses of Demodex mites. In a study in KNP, approximately 50 per cent of 203 buffalo examined had clinical signs varying from few to multiple skin nodules (Wolhuter et al., Reference Wolhuter, Bengis, Reilly and Cross2009).

Figure 9.6 Nodular demodex on a captured African buffalo.
Parasites Commonly Seen in Buffalo Carcasses
Buffalo serve as intermediate hosts for a number of tapeworms and enteric protozoa of sympatric predators, which are the definitive hosts. Cysticercosis usually seen in the muscles of the forelimbs, diaphragm and heart muscle of buffalo are the intermediate stages of the tapeworms of lions, leopards and hyaenas, such as Taenia regis, T. gonyamai and T. crocutae. In some regions where there is a close interface between buffalo and humans, cysticerci of the human tapeworm, T. saginata, have also been found in buffalo.
Two trematodes of common pathological importance for livestock are frequent in buffalo with no clinical signs, namely the giant fluke (Fasciola gigantea) and the small fluke (Dicrocoelium hospes), which are found in buffalo livers, often together and sometimes in a massive infestation. In Central African Republic, 12 of 33 inspected buffalo (36.3 per cent) were affected by both flukes with no apparent clinical signs (Thal, Reference Thal1971). Other trematodes of the genus Schistosoma also are frequently found in the mesenteric blood vessels of buffalo, again causing no clinical signs.
Hydatidosis and Coenurus Cerebralis
Hydatid and coenural cysts are parasitic cysts occasionally observed in buffalo carcasses. These cysts are the intermediate stage of the wild predator and domestic dog tapeworms, Echinococcus granulosis and T. multiceps. Hydatid cysts are most commonly found in the liver, lung and occasionally brain of buffalo, and may be large and multiple. Coenural cysts are much less common, and may be found in the brain or subcutaneous tissues.
Sarcosporidiosis
Sarcosporidia are protozoal parasites that have a two-host life cycle. The sexual reproductive cycle takes place in the small intestine of predators, and sporocysts are passed in their faeces and contaminate the environment. These sporocysts are then ingested by grazing herbivores and undergo asexual replication to form merozoites, which in turn form the sarcocysts in the muscle. Sarcocysts are cysts filled with thousands of bradyzoites, and it is these that will infect a predator when the muscle tissue is eaten, and thus the life cycle is completed. In the African buffalo, large macrocysts caused by Sarcocystis fusiformis (preliminary identification) are most commonly seen in the tongues and pharyngeal muscles of older buffalo, but can occur elsewhere (Quandt et al., Reference Quandt, Bengis and Stolte1997). Most buffalo appear to be infected, and these macrocysts are a common finding at meat inspection and may result in partial condemnation of the carcass.
Pentastome Larvae
The larval stages of a pentastome parasite of the paranasal sinuses of large carnivores, Linguatula serrata, are frequently found in the mesenteric lymph nodes, liver and cardiac chambers of African buffalo at meat inspection.
Conclusions and Summary
We hoped to provide a comprehensive view of infections and macro-parasites of African buffalo, although significant gaps remain in our knowledge. All in all, the African buffalo is a resilient and hardy species that is well adapted to harsh African conditions and pathogen challenges. Much of its reputation as a disease villain is not warranted, and its qualities as a resource for nature, ecosystems and humanity speak for themselves.