7 results
26 - Bohr’s Problem for Vector-Valued Dirichlet Series
- from Part 4 - Vector-Valued Aspects
-
- Book:
- Dirichlet Series and Holomorphic Functions in High Dimensions
- Published online:
- 19 July 2019
- Print publication:
- 08 August 2019, pp 645-663
-
- Chapter
- Export citation
4 - Solution to the Problem
- from Part 1 - Bohr’s Problem and Complex Analysis on Polydiscs
-
- Book:
- Dirichlet Series and Holomorphic Functions in High Dimensions
- Published online:
- 19 July 2019
- Print publication:
- 08 August 2019, pp 93-110
-
- Chapter
- Export citation
9 - Strips under the Microscope
- from Part 1 - Bohr’s Problem and Complex Analysis on Polydiscs
-
- Book:
- Dirichlet Series and Holomorphic Functions in High Dimensions
- Published online:
- 19 July 2019
- Print publication:
- 08 August 2019, pp 205-229
-
- Chapter
- Export citation
12 - Bohr’s Problem in Hardy Spaces
- from Part 1 - Bohr’s Problem and Complex Analysis on Polydiscs
-
- Book:
- Dirichlet Series and Holomorphic Functions in High Dimensions
- Published online:
- 19 July 2019
- Print publication:
- 08 August 2019, pp 289-314
-
- Chapter
- Export citation
1 - The Absolute Convergence Problem
- from Part 1 - Bohr’s Problem and Complex Analysis on Polydiscs
-
- Book:
- Dirichlet Series and Holomorphic Functions in High Dimensions
- Published online:
- 19 July 2019
- Print publication:
- 08 August 2019, pp 3-36
-
- Chapter
- Export citation
7 - Probabilistic Tools I
- from Part 1 - Bohr’s Problem and Complex Analysis on Polydiscs
-
- Book:
- Dirichlet Series and Holomorphic Functions in High Dimensions
- Published online:
- 19 July 2019
- Print publication:
- 08 August 2019, pp 153-180
-
- Chapter
- Export citation
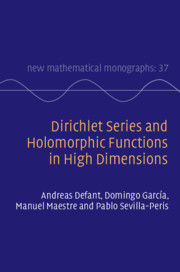
Dirichlet Series and Holomorphic Functions in High Dimensions
-
- Published online:
- 19 July 2019
- Print publication:
- 08 August 2019