INTRODUCTION
The respiratory encapsulated bacterium Streptococcus pneumoniae (pneumococcus) colonizes the nasopharyngeal tract, but can cause disease such as otitis media, pneumonia and invasive pneumococcal disease (IPD). At least 94 different pneumococcal serotypes are known. A polysaccharide pneumococcal vaccine (PPV) protecting against IPD caused by a selection of serotypes has been available since the late 1970s. A 23-valent PPV (PPV23; Pneumovax 23®, Merck, USA) is recommended in Norway, as in many Western societies, to prevent disease in risk groups. PPV is not immunogenic in children aged <2 years. A conjugated vaccine (PCV) has been available since 2000. In Norway, a 7-valent PCV (PCV7; Prevnar, Wyeth, USA) was introduced in the childhood immunization programme in 2006 and replaced by a 13-valent vaccine (PCV13; Prevnar 13®, Wyeth) in 2011. The introduction of PCV has decreased the overall incidence of IPD extensively in children through direct protection, and in non-immunized age groups through indirect protection [Reference Loo1–Reference Rozenbaum4], despite a small increase in the incidence of non-vaccine serotype IPD (serotype replacement [Reference Miller2, Reference Vestrheim5–Reference Weinberger, Malley and Lipsitch8]). Still, persons with certain medical conditions or at advanced age remain at increased risk for severe pneumococcal disease.
Since summer 2013, PCV13, as the first PCV, is licensed for use in all age groups [9]. Because of different immunogenic properties resulting from conjugation to a carrier protein and the added adjuvant (see Table 1), PCV13 may induce better protection against vaccine-type pneumococcal infections than PPV23. With PCV13 being available for all age groups and with the changing IPD epidemiology following PCV implementation in childhood immunization programmes, revision of vaccine recommendations for risk groups outside the childhood immunization programme is needed.
Table 1. Differences between the pneumococcal polysaccharide and conjugate vaccines
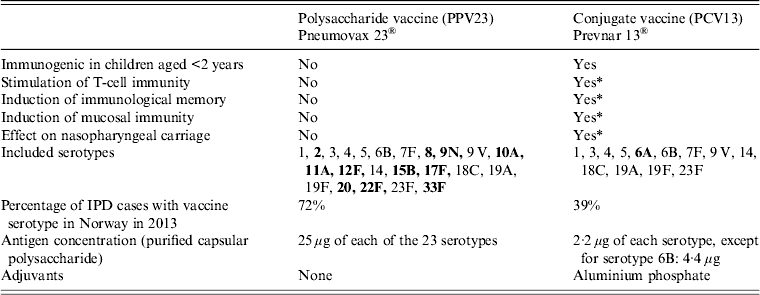
IPD, Invasive pneumococcal disease.
Serotypes in bold indicate serotypes that are only included in the respective vaccine.
* Shown in children. Not yet known if this also accounts for adults.
In this paper we discuss the available evidence to inform pneumococcal vaccine recommendations for risk groups aged ⩾2 years. We show its implications for the Norwegian recommendations, which are now step-wise and consider (1) conditions leading to higher risk for severe pneumococcal disease compared to healthy adults, and per risk group the (2) need for vaccination, (3) choice of pneumococcal vaccines, and (4) re-vaccination interval. If combined with local surveillance data on vaccine-type IPD as measure of the preventive potential of PCV13 and PPV23, our results are also applicable for other (Western) settings that consider changing their vaccine recommendations.
METHOD
An expert group at the Norwegian Institute of Public Health reviewed relevant literature which was identified through search in PubMed, Medline and Google Scholar using qualifiers for: (i) conditions that make people more at risk for severe pneumococcal disease [case-fatality rates (CFR) and risk ratios (RR) or odds ratios (OR) for IPD, pneumonia, or exacerbations/deterioration of medical conditions as a result of pneumococcal infections; based on cohort, case-control and cross-sectional studies], (ii) vaccine effectiveness (VE) of PPV23 and PCV13 in risk groups (percentage of cases prevented; from clinical trials and observational studies [10]) and (iii) safety of the vaccines in risk groups (adverse events reported in clinical trials and observational post licensure studies). Additional information was obtained on risk groups to which vaccination was already recommended by the public health institutes of Norway, Sweden [11], Finland [12], UK [13, 14], Germany [15, 16], The Netherlands [17], and USA [18]. These countries were chosen because of linguistic reasons and their Western societies. Group members complemented the information with expert knowledge. The literature review does not fulfil the criteria for a systematic review as it includes grey literature such as informally published reports, and as results are extrapolated to the current setting using expert consultations, as to fill the gaps for topics for which no literature is currently available.
Through group discussions on the study quality and relevance for a Western setting, the degree of risk for severe pneumococcal disease per risk group was evaluated. Several factors make it difficult to quantify that current risk based on studies from different populations (including non-western societies) or from the pre-vaccination era, such as differences in quality of and access to health care, socioeconomic differences, improved treatment for various diseases and the changed epidemiology since introduction of childhood immunization. Furthermore, several studies have been done in partly vaccinated risk populations, which structurally underestimate the risk of unvaccinated populations. Additionally, the risk is often heterogeneous within risk groups. Based on these factors we decided against pooling results in a statistical meta-analysis, but to categorize the risk conditions in very high, high and moderate risk for severe pneumococcal disease, without quantifying the risk.
Data on effectiveness and safety of the vaccines was obtained from scientific publications and product descriptions. For some risk groups, no VE results were available, and potential beneficial effects had to be extrapolated from data on other risk groups, thereby specifically taking into account the expected level of immunosuppression.
Based on the abovementioned information, supplemented with epidemiological data of the Norwegian Surveillance System for Communicable Diseases [19] on the percentage of IPD cases infected by vaccine serotypes, we defined step-wise recommendations for risk group. This article is based on a Norwegian report [20].
RESULTS
We included 187 peer-reviewed publications and non-peer-reviewed reports in the evaluation. Studies from van Hoek [Reference van Hoek21], Kyaw [Reference Kyaw22] and Vinogradova [Reference Vinogradova, Hippisley-Cox and Coupland23] played a key role for the categorization of risk groups.
Risk groups for severe pneumococcal disease
Groups with very high risk
Immunity against pneumococci is mainly based on antibodies and complement-mediated opsonization, with clearance of bacteria in the liver and spleen. Anatomical or functional asplenia (including sickle cell anaemia [Reference Singer, Rosenberg and Bolander24]), patients with B-cell deficiency (particularly a- and hypogammaglobulinaemia [Reference Quinti25]) and patients with severe T-cell-deficiency are therefore at very high risk for IPD [Reference Picard26]. In asplenic patients, pneumococci causes more than half of the infections leading to sepsis [Reference Holdsworth, Irving and Cuschieri27], often with rapid development of sepsis, multi-organ failure and high mortality rates [Reference Aavitsland28]. Patients with a- and hypogammaglobulinaemia cannot produce antibodies, which increases the risk for severe pneumococcal disease and other infections substantially [Reference Quinti25].
Due to the role of CD4+ T-cells in antibody and cytokine production after antigen recognition, (CD4+) T-cell deficiency leads to impaired immunity. With HIV/AIDS, the risk for IPD was 40–100× higher compared to persons without HIV [Reference van Hoek21, Reference Kyaw22, Reference Barry29, Reference Cohen30]. Interestingly, the risk seems independent of CD4+ counts, and while highly active antiretroviral treatment (HAART) lowers the risk, it remains high [Reference Jordano31], partly because comorbidities are often present [Reference Barry29].
Immune cells develop out of haematopoietic stem cells, which are located in bone marrow. With certain medical conditions (e.g. leukaemia, lymphoma, bone marrow cancer), treatment consists of stem-cell transplantation. Stem-cell transplantation can be autologous (from oneself) or allogeneic (from a donor) and includes treatment with high doses of cytostatic drugs. Additionally, patients with allogeneic stem cells need lifelong immune-modifying treatment to prevent graft vs. host disease. The cytostatic and immune-modifying drugs highly increase the risk for IPD [Reference Kumar32], with allogeneic transplanted patients being at highest risk (1220 vs. 460/100 000 for autogenic transplanted patients [Reference Engelhard33]). In patients with haematological cancer (e.g. Hodgkin's lymphoma, other lymphomas, leukaemia, and multiple myeloma), both the immunosuppressive effect of cytostatic drugs and of cancer itself increase the risk for IPD. The risk increases with increased immunosuppression (4–63× higher than in healthy adults), with myeloma patients at highest risk [Reference Kyaw22, Reference Wong34, Reference Vethe35]. Moreover, organ transplantation patients use lifelong immune-modifying drugs to prevent graft vs. host disease, leading to 13× (95% CI 8–20) higher risk for IPD compared to the general population [Reference Kumar36].
While the very high risk for IPD in the abovementioned conditions results from impaired immunity, certain medical conditions increase the risk due to increased accessibility for bacteria caused by breakdown of barriers such as the blood–brain barrier. Intracranial pathology, e.g. after trauma, surgery or medical procedures, can lead to chronic leakage of cerebrospinal fluid (CSF). CSF leakage highly increases the risk for retrograde infections through the nose, sinuses and ears and therefore leads to a very high risk for IPD [Reference Adriani37].
Groups with high risk
The role of the complement system in labelling bacteria to enhance phagocytosis by macrophages (opsonization) is important for immunity against encapsulated bacteria, such as pneumococci. Complement-defects, specifically C3 defects [Reference Picard26], therefore increase the vulnerability for pneumococcal infections [Reference Figueroa and Densen38]. Other primary immunosuppressive disorders such as T-cell deficiency and neutropenia (lack of neutrophilic granulocytes, which play a role in phagocytosis) also increase the risk for IPD [Reference Picard26].
The level of immunosuppression caused by solid cancer and the immunosuppressive character of cytostatic drugs used for these types of cancer are generally lower compared to haematological cancer, but may be substantially increased leading to high risk for IPD (RR 23, 95% CI 12–44) [Reference Kyaw22]. The risk for community-acquired pneumonia, which is often caused by pneumococci, is increased specifically in lung cancer patients (OR 5, 95% CI 4–6) [Reference Vinogradova, Hippisley-Cox and Coupland23].
The liver has an important function as ‘sieve’ for bacteria, which are subsequently phagocytized and degraded by specialized macrophages (Kupffer cells). Chronic liver disease decreases the capacity to clear bacteria, thereby increasing the risk for IPD. The risk is specifically high in case of cirrhosis (OR 33, 95% CI 31–36) [Reference van Hoek21, Reference Kang39], in which neutrophil-mediated phagocytosis may also be diminished [Reference Propst-Graham40]. Patients with active hepatitis and primary biliary cirrhosis are less vulnerable to IPD (RR 3, 95% CI 3–4) [Reference Wotton and Goldacre41].
While patients with chronic kidney disease are at higher risk for pneumonia (OR 1·7, 95% CI 1–2) [Reference Vinogradova, Hippisley-Cox and Coupland23] and IPD (OR 7, 95% CI 6–7) [Reference van Hoek21] with higher mortality rates, it is not completely clear which mechanism increases the risk. Uraemia-related immunosuppression, in addition to effects of dialyse-related interventions, may play a role.
The weakened immune system, deterioration of natural barriers, delayed diagnosis, and/or diminished response to therapy [Reference Gavazzi and Krause42] make elderly (individuals aged ⩾65 years) more susceptible for IPD [Reference van Hoek21]. The risk is even increased in elderly without other medical conditions (RR 2–7 compared to healthy adults aged 50–64 years [Reference Kyaw22]). In 2013, 50% of all notified IPD cases in Norway were aged ⩾65 years.
Homeless individuals and drug addicts are generally more susceptible for infections because of their living conditions, lack of hygiene, malnutrition, and because comorbidities are common. The IPD incidence was up to 30× as high as in the general adult population [Reference Plevneshi43]. Similar risks can be expected for individuals with badly managed mental illnesses [Reference Hwang44]. In alcohol-addicted individuals, the risk was increased only in those consuming more than 25 alcoholic units per day (OR 7, 95% CI 2–30) [Reference Nuorti45].
In individuals who are deaf or have severely diminished hearing, an electronic hearing aid may be implanted inside the cochlea (cochlea implants). During surgery, the inner ear is opened, which poses a risk for infections. The risk for IPD, specifically pneumococcal meningitis, is therefore increased up to 30×, but decreases with time after surgery [Reference Reefhuis46, Reference Rubin and Papsin47].
Groups with moderate risk
The use of immune-modifying drugs can lead to iatrogenic immunosuppression, which increases the risk for severe pneumococcal disease [Reference Bongartz48–Reference Gluck and Muller-Ladner50]. Examples of such drugs are cytostatic drugs and disease modifying anti-rheumatic drugs (DMARDs). The majority of these drugs are inhibitors of the inflammatory cytokine tumour necrosis factor-α (TNF-α). Immunosuppression occurs at systemic doses of ⩾2 mg/kg or ⩾20 mg/day for ⩾2 weeks, or with inhaled steroids at 800 mg/day. Because of differences in kind of medication, doses and duration of treatment, and differences in severity of the medical condition, the immunosuppressive effect increasing the risks for IPD is heterogeneous within and between risk groups with iatrogenic immunosuppression [Reference Kapetanovic51].
Coeliac disease is an immune-modulating condition with reduced absorption and increased permeability of the intestine. Extraintestinal complications like lymphoma, hyposplenia and other immunosuppressive conditions may be present, which increase the risk for IPD (RR 2, 95% CI 1–3) [Reference Thomas52, Reference Ludvigsson53] and mortality of sepsis [Reference Peters54].
In persons with diabetes, the risk for IPD is increased up to 5× compared to individuals without diabetes [Reference van Hoek21, Reference Kyaw22, Reference Wotton and Goldacre41], as high blood glucose concentrations reduce the ability to clear bacteria [Reference Kumar, Clark, Kumar and Clark55]. At the same time (pneumococcal) infections can lead to deregulation and more difficult monitoring of diabetes. The cardiovascular and renal complications that can occur in patients with long-lasting diabetes, likely further increase the risk [Reference Knapp56]. That cardiovascular problems increase the risk for pneumonia and IPD has also been shown in chronic heart patients (RR 6, 95% CI 4–11 [Reference Kyaw22]; OR 2, 95% CI 1·8–2·4 [Reference Vinogradova, Hippisley-Cox and Coupland23]).
As pneumococci are transmitted via the respiratory system, individuals with chronic respiratory disease [e.g. chronic obstructive pulmonary disease (COPD), emphysema and asthma] are more susceptible to pneumococcal infections, though, the degree of risk is heterogeneous (RR 1–6) [Reference van Hoek21, Reference Kyaw22, Reference Lee, Weaver and Weiss57]. Nasopharyngeal colonization by pneumococci may increase the risk for exacerbations of COPD [Reference Bogaert58]. Other conditions that may impair ventilation capacity are e.g. neurological conditions like stroke, tetraplegia, multiple sclerosis and myasthenia gravis, or severe osteoporosis with kyphosis and Bechterew's disease. If clearance of the airways by coughing is reduced, the risk for pneumonia and the fatality rate during pneumonia increases [Reference Vinogradova, Hippisley-Cox and Coupland23, Reference Wotton and Goldacre41, Reference Hartkopp59, Reference Katzan60].
Certain individuals have recurrent pneumococcal infections. While the reason for recurrent infections is unknown, unrecognized medical conditions may be present, as was shown in a Dutch nation-wide study where 77% of those with recurrent meningitis had a risk condition [Reference Adriani37].
Some occupations expose employees to higher risk for (severe) pneumococcal infections, either because of increased exposure (individuals working in laboratories with pneumococci), or because of increased susceptibility (welders [Reference Coggon61, Reference Koh62]). The increased susceptibility among welders results from exposure to metal fumes, inorganic dust and chemicals which may cause airway inflammation [Reference Koh62, Reference Toren63]. The increased risk is twice the risk among construction workers who are not exposed to metal fumes, inorganic dust and chemicals (95% CI 1–4) [Reference Toren63]. The risk is reversible. The risk is highest among welders who smoke, with infections that may have a fulminant course [Reference Wong64]. Smoking also increases the risk for IPD in the general population (OR 4, 95% CI 2–7) [Reference Nuorti45]. The risk decreases if time since stopping of smoking increases.
Vaccines protecting against S. pneumoniae
Two different kinds of vaccines are available to prevent pneumococcal infections caused by vaccine-serotypes: PPV and PCV. The two vaccines stimulate the immune system differently (Table 1). PPV is T-cell independent, so it only stimulates B cells to produce antibodies. Immunological memory is absent. Furthermore, it does not induce mucosal immunity, and therefore does not affect nasopharyngeal colonization. PCV is conjugated to a carrier protein (CRM197 in PCV13) to enhance the immune-response through T-cell activation and is adsorbed to aluminium-phosphate as adjuvant. It was shown in children that the T-cell-dependent response provides immunological memory, and prevents colonization of vaccine-serotypes through mucosal immunity [Reference Spijkerman65]. The currently available PCVs (10- and 13-valent) cover a smaller percentage of serotypes causing IPD than PPV23. PCV13 and PPV23 have 12 serotypes in common. In 2013, 72% of all notified IPD cases in Norway were caused by serotypes included in PPV23, while 39% were caused by PCV13-serotypes. For the population aged ⩾65 years, this was 69% and 29%, respectively. The percentage coverage for PCV13 may decrease further as a result of indirect protection of childhood vaccinations.
The effect of vaccination
The studies evaluating the effect of PPV23 are generally based on its direct protective effect against disease (VE), while the evaluation of PCV in risk groups is generally based on its immunogenicity. Immunogenicity is defined by IgG antibody concentrations measured by enzyme-linked immunosorbent assays (ELISA) or antibodies' functional activity measured by opsonophagocytic assays (OPA). To interpret immunogenicity results, clinical cut-offs for ELISA or OPA results resembling protection against infection are needed. Such cut-off is defined for children (⩾0·35 μg/serotype per ml measured with ELISA [66]), but is not clearly defined for adults. Levels of ⩾1·0 μg/ml may be needed for long-term protection. The lack of such clear cut-off makes interpretation of immunogenicity studies less straightforward than VE studies.
Generally, one dose of PPV23 protects 50–70% of adults against IPD [Reference Shapiro67, Reference Moberley68], although there is controversy about the quality and representativeness of different VE studies and therefore about the actual VE [Reference Huss69]. Protection against pneumonia is generally poor [Reference Moberley68, Reference Melegaro and Edmunds70, Reference Jackson71]. Effectiveness studies of PCV13 in adults are limited, but one dose PCV13 probably induces an adequate immune-response [Reference Scott72]. A meta-analysis of randomized clinical trials in children before implementation in childhood immunization programmes showed 29% efficacy against X-ray-confirmed pneumonia and 90% efficacy against vaccine-type IPD [Reference Pavia73]. Results of a large study measuring PCV13 VE against pneumonia and against IPD in older adults were still unavailable at the time of this evaluation [Reference Hak74].
Effectiveness in risk groups
Several (host) factors may decrease VE, specifically the presence of (iatrogenic) immunosuppression, thereby leading to heterogeneity of VE in those risk groups. While immunosuppressed patients generally respond to vaccination (PPV23 and a similar effect is expected for PCV13), their response may be diminished compared to healthy individuals. The best immune-response is obtained when vaccination is administered as early as possible in the course of disease, ideally prior to the start of immune-modifying therapy [Reference Agarwal75]. A diminished vaccine response was observed in patients after bone-marrow or organ transplantation, patients with haematological cancer, chronic liver disease, inflammatory bowel disease, patients using different immune-modifying therapies concurrently, and potentially in patients with chronic kidney or respiratory disease [Reference Agarwal75–Reference Mahmoodi81]. For individuals with bone marrow transplantation, the response may even be diminished up to 4–10 years after transplantation [Reference Nordøy78]. Patients with severe B-cell deficiency have an impaired antibody response, making them unresponsive to vaccination. In asplenic patients, solid cancer patients, patients being treated with TNF-α inhibitors alone, rheumatic patients, diabetics, as well as the elderly for whom advanced age is the only risk factor, the vaccine response was not diminished ([Reference Butler82–Reference Lin85]; for review see [Reference Agarwal75]). In HIV patients, PPV23 provides limited protection [Reference French86], while PCV protected 74% (95% CI 30–90) of IPD cases [Reference French87].
Combination of PCV13 and PPV23
Because PPV23 and PCV13 stimulate the immune system differently, it might be advantageous to combine the two vaccines. In combined regimens, the order and time interval are important. To prevent a decreased immune response, PCV13 should be administered first, with PPV23 administered at least 8 weeks after PCV13 [9, 88, 89]. While there is no clear recommendation on when PCV13 should be administered in individuals who have previously been vaccinated with PPV23, we recommend a time interval of at least 3 years. This interval is based on the fact that: (1) there is little vaccine failure of PPV23 within the first 3 years, (2) the response to PCV is decreased if the interval between PPV23 and subsequent PCV13 is only 1 year [88], (3) the frequency of adverse events in individuals who obtained PCV within 3 years after PPV23 is increased [90], and (4) because PPV23 covers the same (and more) serotypes as PCV13, except for serotype 6A.
Small clinical trials in asplenic patients suggest that antibody responses may last longer if PCV is used in addition to PPV23 [Reference Smets83]. Moreover, in case of Hodgkin's lymphoma, a combination of PCV and PPV may improve the immune-response. Note that this was suggested by a study using PCV7 that was conjugated to a different carrier protein than the one used in currently available PCV [Reference Chan91]. For organ-transplanted patients, a second PCV or PPV vaccination did not improve the vaccine response [Reference Lin85, Reference Gattringer92]. For other risk groups, including the elderly, there is no clear documentation of an additional clinical benefit of PCV when used in combination with PPV23 [Reference Jackson93, Reference de Roux94].
Re-vaccination
Because of waning antibody concentrations after PPV23, revaccination is required, generally after 10 years, but in asplenic patients and patients who are severely immunosuppressed, revaccination may be required after shorter time intervals. Re-vaccination does not lead to a booster response (i.e. stronger and prolonged effect with immunological memory) but induces a response similar to a primary response. If vaccination with PPV23 is repeated within a short time interval (e.g. 1–2 years), a low response to vaccination can be observed (hyporesponsiveness [Reference O'Brien, Hochman and Goldblatt95]), and the probability of adverse reactions increases considerably. With a time interval of at least 5 years, hyporesponsiveness has not been observed [Reference O'Brien, Hochman and Goldblatt95]. Based on existing knowledge, there is no reason to set a maximum to the number of re-vaccinations with PPV23.
For PCV, the duration of protection and potential need for re-vaccination is still unknown. Furthermore, it is unknown whether PPV23 provides a booster response in individuals previously vaccinated with PCV.
Conclusion of vaccine effect
There is long-standing clinical experience with PPV23 providing adequate protection against IPD in most risk groups, but limited protection against pneumonia. PCV13 likely provides T-cell-mediated immunity with immunological memory and may therefore provide better protection against pneumococcal infections caused by the 12 shared vaccine serotypes, but the overall coverage of serotypes that cause IPD is lower for PCV13 than for PPV23. Overall, the percentage of cases that may be prevented by PCV13 is evaluated as being too low in Norway (39% in 2013) to only recommend PCV13, and the available data on an additional effect of PCV13 when used in combination with PPV23 is currently too limited for entire risk groups.
Vaccine safety
Both, PPV and PCV, are non-live vaccines and can therefore be administered to immunosuppressed individuals. Very few severe adverse events after PPV administration have been described, but local adverse reactions such as injection-site pain, swelling, erythema, headache, fatigue, and myalgia are common (>10%), but generally resolve within 5 days [90]. The frequency of adverse events increases with the number of administered PPV doses. The frequency of local adverse reactions after PCV is similar to that after PPV. Additionally, in adults, reduced appetite, chills and diarrhoea were observed. The frequency does not increase after more PCV doses [9, 89]. There is limited data on rare adverse events after PCV administration in adults.
There has been discussion on whether inducing an immune response in transplanted patients may lead to rejection of the transplanted organ. No such rejection has been observed after PPV or PCV administration, and pneumococcal vaccination appears safe in transplanted patients [Reference Avery and Michaels96].
Implications of the current evidence for vaccine recommendations
For vaccine recommendations, the evidence on risk groups should be combined with evidence on the effectiveness and safety of the available vaccines in preventing severe disease within the risk groups as well as the size of the potential preventive effect that a vaccine can have with the current and local epidemiology. Time intervals between different vaccines or revaccination should be defined, when different vaccines are combined or immunity wanes over time. Because of the safety profiles of PPV23 and PCV13, safety does not need to be addressed further when deciding on pneumococcal vaccine recommendations for risk groups. Vaccine recommendations should be re-evaluated when new evidence becomes available.
As summarized in Table 2, the severity of the patient's condition, and the corresponding vulnerability for severe pneumococcal disease or exacerbations of medical conditions, are too heterogenic in several risk groups to recommend vaccination to each individual in such risk groups. This particularly applies to persons with one risk condition and whose condition is mild and well-regulated, such as asthma in mild form, smoking or alcohol abuse. Figure 1 shows in a step-wise manner the need to vaccinate, as defined in Norway. Pneumococcal vaccination is only recommended to all individuals aged ⩾2 years with a condition that poses a very high risk for IPD, as well as to groups where a high risk is assumed to be evenly distributed: individuals with cochlear implants and individuals aged ⩾65 years. For other, more heterogeneous risk groups, the need for vaccination should be assessed on individual base, depending on the patient's condition. Key factors for the decision to vaccinate are the number and severity of the risk conditions and thereby the expected risk for IPD.

Fig. 1. Flow diagram for the decision on vaccination of risk groups aged ⩾2 years in Norway from May 2013 [20].
Table 2. Categorization of risk groups and the corresponding vaccine recommendations for risk groups aged ⩾2 years in Norway from May 2013 [20]

IPD, Invasive pneumococcal disease.
* In Norway, hospitals have their own guidelines for patients who have had bone marrow transplantation.
If there is a need to vaccinate, we recommend vaccination with PPV23 because of the higher coverage of serotypes compared to PCV13 and its long-standing experience. We evaluated the additional value of PCV13 as low for most risk groups. Still, the most fragile patients should have access to the potential added effect of PCV13 in combination with PPV23, despite the lack of demonstrated effect. We therefore recommend PCV13 in addition to PPV23 to patients with asplenia and HIV/AIDS that never have had PCV13 before, because of the rapid and fulminant course (asplenia) or the clear additional benefit (HIV/AIDS). For all other risk groups, the use of PCV13 in addition to PPV23 should be assessed individually based on the expected risk for IPD and aspects in relation to potential transplantation or start of immunosuppressive therapy. In Norway we do not recommend PCV13 for individuals for whom advanced age (⩾65 years) is the only risk factor. Because of different societal, economical and epidemiological situations, several countries have concluded on other risk groups and vaccine recommendations. While in Finland, Denmark and the USA, PCV13+PPV23 is recommended to most risk groups [10, 12, 18], the UK recommends only PPV23 [13], with the exception of severely immunocompromised individuals for whom PCV13 should be used in addition to PPV23 [14]. In Germany, PCV13 in addition to PPV23 is recommended only for individuals with immunosuppression or chronic kidney disease [15, 16].
In case PCV13 will be used, PCV13 should be administered at least eight weeks before PPV23, though, if PPV23 has been administered before, PCV13 should be administered at least 3 years after the last PPV23. Revaccination is only recommended for PPV23, generally after 10 years but may be decided earlier based on the severity of the medical condition. For asplenic patients, the revaccination-interval should be determined upon measurement of antibody concentration after 5 years (the interval should be a minimum of 3 years).
CONCLUSIONS
While for some risk groups the increased risk for severe pneumococcal disease is assumed to be evenly distributed, for most risk groups the vulnerability is heterogenic. Norway therefore decided on stepwise recommendations with individual assessment of the need to vaccinate. Although PCV13 may be more effective then PPV23 against pneumococcal disease caused by the 12 shared serotypes, it is the local epidemiology that directs its potential to prevent disease. PPV23 is therefore still the main choice in Norway, although, PCV13 may be used additionally in the most fragile patients. More evidence is needed on a potential additional benefit of PCV13 when used in combination with PPV23. If combined, PCV13 should be administered at least 8 weeks before PPV23.
ACKNOWLEDGEMENTS
This research received no specific grant from any funding agency, commercial or not-for-profit sectors.
DECLARATION OF INTEREST
None.