In newborns with congenital heart disease, approximately twenty percent have anomalies affecting the right ventricular outflow tract, such as tetralogy of Fallot with or without pulmonary atresia, requiring surgical repair with bioprosthetic valves, valved conduits or transannular patch. Reference Verheugt, Uiterwaal and van der Velde1 Severe pulmonary valve regurgitation is observed in almost half of this population immediately after the initial operation and in 85% after 2 years. Reference Ong, Boone and Gao2,Reference Boethig, Thies, Hecker and Breymann3
The haemodynamic burden of chronic pulmonary regurgitation after surgical palliation has been associated with late morbidity including exercise intolerance, right heart failure, and arrhythmias. Reference Tatewaki and Shiose4 These long-terms outcomes in these congenital heart disease patients are responsible for a significant decrease in survival in the second decade after repair. Reference Nollert, Fischlein, Bouterwek, Bohmer, Klinner and Reichart5 There is a consensus that pulmonary valve replacement is indicated in symptomatic patients with significant pulmonary regurgitation, signs of right ventricular failure, and arrhythmias. Reference Verheugt, Uiterwaal and van der Velde1 Several MRI-based publications have focused on establishing imaging criteria for timing pulmonary valve replacement in those with minimal or no cardiac-related symptoms. Reference Meijboom, Roos-Hesselink and McGhie6-Reference Ammash, Dearani, Burkhart and Connolly9 However, only a few advanced imaging reports have centred attention on how to determine best measurements to assess candidacy for available transcatheter pulmonary valve when indicated. Reference Ferrari, Shehu and Mkrtchyan10-Reference Secinaro, Muscogiuri and Chinali12
At our centre, right ventricular outflow tract intervention has evolved to encompass transcatheter, surgical, or hybrid palliative methodologies. Reference Ligon, Latson and Ruzmetov13 The latter includes off-bypass surgical plication, then subsequent transcatheter pulmonary valve replacement. Transcatheter replacement could be accomplished in most patients necessitating intervention, with surgical and hybrid methodologies being implemented in those with landing zones too large for current uniformly available balloon-expandable transcatheter technologies. Surgical pulmonary valve replacement was reserved for patients with positive coronary compression testing, stent/valve system migration during deployment, or patient preference. A standardised algorithm for pre-procedure workup to pulmonary valve replacement included MRI with identification of a proper intended valve site and measuring the right ventricular outflow tract perimeter (circumference) in end-systole, in addition to traditional cross sectional diameter dimensions in orthogonal planes. The perimeter measurement is divided by π to determine the diameter of the region when forced into a circular shape (circularised diameter) by a valve prosthesis.
Several methodologies have been outlined in the literature attempting to predict candidacy for transcatheter replacement by MRI Reference Ferrari, Shehu and Mkrtchyan10,Reference Leonardi, Secinaro, Perrone, Curione, Napolitano and Gagliardi14 or CT angiography. Reference Curran, Agrawal and Kallianos15,Reference Rinaldi, Sadeghi and Rajpal16 These efforts have focused on diameter measurements from advanced imaging during different phases of the cardiac cycle or extrapolating risk of coronary compression based on proximity of the coronary arteries. However, no study has directly outlined MRI-derived landing zone perimeter/circularised diameter and their correlation to transcatheter candidacy. Our primary aim was to compare patients by MRI-derived perimeter/circularised diameter values to their palliative interventions performed (transcatheter versus surgical/hybrid methodologies). Additional aims included correlation of landing zone perimeter/circularised diameter to transcatheter balloon sizing results prior to intervention.
Materials and methods
Retrospective review of patients undergoing pulmonary valve replacement with a primary indication of pulmonary regurgitation from January 2017 to January 2021. All MRI studies were performed on a 1.5-T clinical scanner and encompassed multiplane reconstruction from three-dimensional steady-state free-precession electrocardiographic-gated and respiratory navigated sequences (the preferred methodology). If this sequencing was not available, then contrast magnetic resonance angiography (Twist) sequence was utilised. Patients who underwent CT due to non-compatible materials or underwent surgical palliation due to coronary compression were excluded for the purposes of this comparative study. Percutaneous transcatheter pulmonary valve replacement was performed in a cardiac catheterisation laboratory under general anaesthesia. Biplane angiography of the intended landing was performed to assess native diameters and length of the right ventricular outflow tract in two planes. Balloon sizing, which forces the landing zone into a circular cross section, was performed in the right ventricular outflow tract or “landing zone” (whether native, surgical conduit, or bioprosthetic valve), making special note of the diameter of the waist in the balloon (all performed in the straight lateral – 90 degrees left anterior oblique projection) as well as simultaneous coronary compression testing via an aortogram. This study was approved by our institutional review board.
Cardiac magnetic resonance
MRI was performed on a 1.5 T Siemens Aera (Siemens Healthcare Sector, Erlangen, Germany). The MRI protocol included functional imaging using balanced steady-state free precession cine imaging, phase-contrast imaging for flow analysis and quantification, non-contrast and contrast (Twist) for multiplanar reconstruction for measurement purposes, followed by late Gadolinium enhancement sequence for fibrosis/scar assessment. Late gadolinium-enhancement images were obtained using a breath-hold, phase-sensitive inversion recovery sequence in the four-chamber and short-axis planes beginning 12–15 minutes after contrast was administered.
Cardiac magnetic resonance post-processing
All MRI post-processing was performed blinded to clinical data by an image analyst with all analyses verified by a cardiologist (LEH) using CVi42 v. 5.13.5 (Circle Cardiovascular®, Calgary, AB, Canada). Ventricular volume and function were measured in the end systolic and end diastolic phase on SSFP cine images acquired in a short axis plane as a stack of 12 contiguous slices covering full length of both ventricles. The landing zone end-systolic (given that this represents the largest vascular dimension) perimeter and two orthogonal diameters were measured retrospectively in all study patients using multiplanar reconstruction by an imaging and interventional staff member (first author RAL and senior author LEH), agreeing on the intended valve site within the right ventricular outflow tract (Fig 1). The multiplanar reconstruction measurement was performed using non-contrast MRI sequence (whole heart) or a Twist sequence. The perimeter measurements were all calculated into a “circularised” diameter – derived via measurement division by π. Additional MRI parameters and cardiac catheterisation results (including right ventricular outflow tract balloon sizing and/or transcatheter pulmonary valve replacement procedures) were collected retrospectively for data analysis.

Figure 1. Right ventricular outflow tract measurement by cardiac MRI.
Statistics
MRI parameters and cardiac catheterisation results (including right ventricular outflow tract balloon sizing and/or transcatheter pulmonary valve replacement procedures) were collected retrospectively for data analysis. Outcomes of interventions and patient imaging results were outlined via descriptive analyses. Patient interventions were divided into cohorts of transcatheter and surgical/hybrid pulmonary valve replacement. A t-test was utilised to determine any statistically significant differences in the baseline characteristics of the cohorts. Bivariate analysis using nonparametric measures (Spearman’s rank correlation coefficient) was implemented to evaluate for any correlation between the circularised diameters to the median diameter of the narrowest region during actual transcatheter landing zone balloon sizing. Statistical analyses were performed using SPSS version 23 (IBM, NY NY).
Results
A total of 37 patients underwent pulmonary valve replacement that met study criteria – 21 transcatheter, 7 surgical, and 9 hybrid (Table 1). The study population was divided into cohorts that underwent transcatheter intervention (n = 21) versus surgical/hybrid intervention (n = 16); the latter being those patients with landing zones too large then available transcatheter technologies (determined by right ventricular outflow tract balloon testing).
Table 1. Study cohort characteristics and advanced imaging findings.

Abbreviations: MRI = magnetic resonance imaging, RVOT = right ventricular outflow tract.
Hybrid palliation (n = 9) included off-bypass surgical plication, then subsequent transcatheter pulmonary valve replacement. Surgical pulmonary valve replacement (n = 7) was reserved for patients with positive coronary compression testing (n = 4), stent/valve system migration during deployment (n = 1), or patient preference (n = 2). The most common anatomic diagnosis for the transcatheter (n = 13) and surgical/hybrid (n = 11) cohorts was tetralogy of Fallot. The median age at intervention for the transcatheter cohort was 28 years (range 8–70) and 22 years (10–50) for the surgical/hybrid; the median weight was 66.6 kg (13.9–96.5) and 51.3 kg (24.2–111), respectively. For these baseline characteristics, there was no statistically significant difference between the age (p = 0.21) nor weight (p = 0.11) in the two cohorts.
There were three patients (all within the transcatheter cohort) that did not have the non-contrast steady-state free-precession magnetic resonance angiography available, and the Twist sequence was utilised. The mean landing zone perimeter of the transcatheter cohort was 88.9 ± 8.7 mm and in the surgical/hybrid cohort measured 106.6 ± 7.5 mm (Fig 2). The two-dimensional diameter measurements from the same identified intended valve site within the transcatheter cohort were median 24.7 mm (range 19.9–27.2) in the smaller dimension and 31.3 mm (27–38.1) in the larger. For the surgical/hybrid cohort, this was 26.3 mm (22.5–33.1) and 39.7 mm (36.2–48.6), respectively. For the transcatheter cohort, the median “circularised” diameter derived from the MRI perimeter measurement (divided by π) was 27.7 mm (range 24.3–32.4). Notably, this correlated (r = 0.93, p < 0.01) with the median diameter of the narrowest region during actual transcatheter landing zone balloon sizing (lateral imaging) of 27.1 mm (range 23.2–30.1) (Fig 3). After transcatheter valve implantation, the mean outer diameter of the implanted prosthesis was 26.7 ± 2.2 mm. In comparison, the median circularised diameter based on the MRI-perimeter in the surgical/hybrid cohort was 33.5 mm (range 30.3–38).

Figure 2. Mean end-systolic right ventricular outflow tract perimeter (derived from cardiac MRI) of the surgical/hybrid palliation cohort (blue) versus the transcatheter cohort.
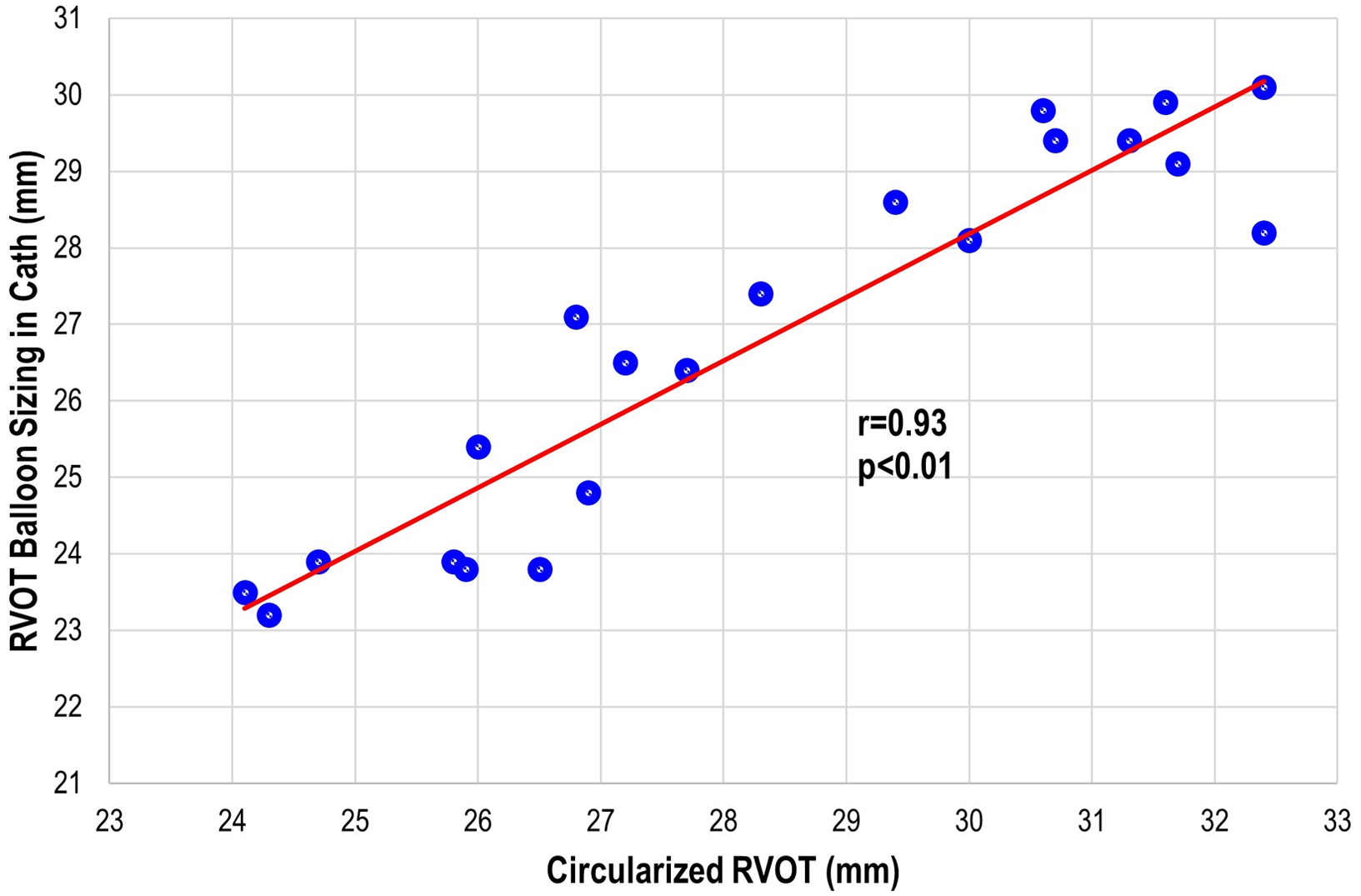
Figure 3. Transcatheter pulmonary valve replacement cohort: correlation plot of the actual right ventricular outflow tract (RVOT) sizing during cardiac catheterisation to the circularised RVOT tract derived from the perimeter measurement on advanced imaging.
Discussion
The utilisation of MRI for determining pulmonary valve replacement indication(s) has been widely reported in the literature. Reference Meijboom, Roos-Hesselink and McGhie6-Reference Ammash, Dearani, Burkhart and Connolly9,Reference Geva17 However, the use of this advanced cardiac imaging to determine which palliative methodology is employed in individual patients remains very limited. Reference Ferrari, Shehu and Mkrtchyan10-Reference Secinaro, Muscogiuri and Chinali12
Given the vast array of right ventricular outflow tract morphologies necessitating pulmonary valve replacement, Reference Schievano, Capelli and Young18-Reference Schievano, Coats and Migliavacca21 calculating perimeter may prove more clinically beneficial than traditional measurements such as two-dimensional diameters. As outlined above, no transcatheter pulmonary valve replacement patient demonstrated a landing zone perimeter larger than 101.8 mm (circularised diameter 32 mm), and no surgical/hybrid patient had one smaller than 95.1 mm.
Various advanced imaging modalities have been explored in regards to guiding transcatheter valve replacement, including intravascular ultrasound. Reference Rudzinski, Kalinczuk, Mintz and Demkow22,Reference Kalinczuk, Mintz, Chmielak, Rudzinski and Witkowski23 Ebel et al compared invasive balloon sizing results with advanced imaging (cardiac magnetic resonance angiography) analyses and demonstrated the highest correlation coefficients for the maximum and effective diameter measured in systole. Reference Ebel, Gottschling and Buzan24 Curran et al calculated retrospective CT measurements of the right ventricular outflow tract in patients with landing zone dysfunction that underwent attempted transcatheter pulmonary valve replacement. Reference Curran, Agrawal and Kallianos15 This entailed landing zone circumference-derived and area-derived diameter parameters which correlated well with implanted valve size, especially in native right ventricular outflow tract or pulmonary bioprosthesis. A predicted valve category was generated for each patient using four diameters (maximum, minimum, area, and circumference), which was then compared to the category of the implanted transcatheter valve. Our study highlights consideration to a parameter able to be extracted from the standard workup for pulmonary valve replacement in most institutions (MRI) in addition to the standard diameter measurements in the orthogonal planes. The landing zone perimeter and the extrapolated circularised diameter was then compared to patient outcomes and candidacy for transcatheter pulmonary valve replacement that demonstrated correlation. This speaks to its potential clinical utility as well as reduction in measurement variability across users.
Candidacy for transcatheter pulmonary valve replacement is determined by a litany of considerations including landing zone morphology, distensibility/compliance of the landing zone, and risk of coronary artery compression. Unfortunately, no one advanced imaging technique will definitively answer all the above factors when taken in isolation. Therefore at our centre, we have generated an institutional systematic approach to pulmonary valve replacement. Reference Ligon, Latson and Ruzmetov13 This entails MRI (CT if not a candidate), which includes calculation of landing zone perimeter/circularised diameter and close examination of coronary artery proximity to the landing zone in order to best counsel the patient and/or family. The patient will then come to the cardiac catheterisation laboratory for landing zone balloon sizing and coronary artery compression testing. The landing zone perimeter has proven to be a valuable advanced imaging parameter to assist in stratifying patients into categories of potential candidates for balloon expandable transcatheter pulmonary valve replacement versus need for other methodologies. That is, patients with a large landing zone by advanced imaging (landing zone perimeter approximately greater than or equal to 98 mm or circularised perimeter of about 31 mm) are not considered candidates for traditional balloon-expandable transcatheter valve replacement. As demonstrated in Fig 2, there is certainly overlap of these populations that guides our interventional team significantly in regard to patient counseling and our heart centre in regard to multidisciplinary consensus recommendations. If transcatheter pulmonary valve replacement is not technically feasible, then consideration to hybrid off-pump plication (without cardiopulmonary bypass) or standard on-pump surgical pulmonary valve replacement is undertaken in a multidisciplinary fashion – with the preference being on the former.
Our study is limited by its retrospective nature from a single institution, recognising that the surgical and transcatheter practices may vary widely from centre to centre. There are inherent disadvantages to statistical analyses of these patient cohorts given their limited sizes and heterogeneity of the subgroups. As mentioned above, non-contrast steady-state free-precession magnetic resonance angiography was not available in all patients, thus a Twist sequence was utilised in a select few (n = 3). It was not felt by the authors that identification of landing zone or measurements were compromised secondary to the acquired images, but this belief may be prone to subjective bias that may not be applicable to other operators. Additionally, self-expanding system technologies such as the Medtronic Harmony™ valve (Medtronic, Minneapolis, MN) Reference Gillespie, Benson and Bergersen25-Reference Schoonbeek, Takebayashi and Aoki27 and the Edwards Alterra Adaptive Prestent™ (Edwards Lifesciences, Irvine, CA) Reference Zahn, Chang, Armer and Garg28,Reference Balzer29 require different types of measurements than the balloon dilatable stent valves that were the only style available until recently. During patient counseling, this new approach is also reviewed and any additional imaging (CT) or landing zone analysis is performed to evaluate candidacy for these new technologies as well – none of these patients (that received self-expanding stent technologies) were included for the purpose of this study. Measurement of landing zone perimeter/circularised diameter allows us to identify patients who are likely candidates for standard balloon dilatable transcatheter valves. Those with borderline measurements can then be sent for additional imaging as needed for newer self-expanding valves or surgery. This approach allows for improved patient counseling and helps enable a standardised approach from our institution surrounding right ventricular outflow tract intervention.
MRI-derived landing zone perimeter/circularised diameter is useful in planning pulmonary valve replacement in terms of candidacy of transcatheter methodologies versus the need for a surgical/hybrid approach. These measurements should be added to reports on patients being considered for pulmonary valve replacement.
Acknowledgements
None.
Financial support
This research received no specific grant from any funding agency, commercial, or not-for-profit sectors.
Conflicts of interest
None.