1. Introduction
In the 2015 Paris Agreement (PA), the parties to the UN Framework Convention on Climate Change (UNFCCC) agreed to ‘[h]olding the increase in the global average temperature to well below 2 °C above pre-industrial levels and pursuing efforts to limit the temperature increase to 1.5 °C above pre-industrial levels’ (UNFCCC, 2015, Art. 2). In order to evaluate whether this can be achieved, the Intergovernmental Panel on Climate Change (IPCC) published a Special Report ‘Global Warming of 1.5 °C’ (IPCC 1.5 °C report) in 2018, presenting emission pathways consistent with remaining below this limit. In the report, all pathways which would allow us to achieve this aim of limiting ‘global warming to 1.5 °C with limited or no overshoot project the use of carbon dioxide removal (CDR) on the order to 100–1000 Gt CO2 over the 21st century’ (IPCC, 2018, p. 19). Similarly, the vast majority of scenarios in line with remaining below 2 °C in the IPCC Fifth Assessment Report of 2014 (AR5) contain the large-scale application of negative emissions technologies (NETs) (Fuss et al., Reference Fuss, Jones, Kraxner, Peters, Smith, Tavoni, van Vuuren, Canadell, Jackson, Milne, Moreira, Nakicenovic, Sharifi and Yamagata2016, p. 2; IPCC 2014, p. 57), specifically of bio-energy with carbon capture and storage (BECCS) and afforestation (Rayner, Reference Rayner2016, p. 1). In line with this, various observers argue that the PA's objective can only be achieved by including NETs into a portfolio of climate measures (Fuss et al., Reference Fuss, Canadell, Peters, Tavoni, Andrew, Ciais, Jackson, Jones, Kraxner, Nakicenovic, Le Quéré, Raupach, Sharifi, Smith and Yamagata2014; Gasser et al., Reference Gasser, Guivarch, Tachiiri, Jones and Ciais2015, p. 5; Goldberg & Lackner, Reference Goldberg and Lackner2015, p. 238; Greene et al., Reference Greene, Huntley, Archibald, Gerber, Sills, Granados, Beal and Walsh2017, p. 279; Horton et al., Reference Horton, Keith and Honegger2016, p. 3; Jones et al., Reference Jones, Ciais, Davis, Friedlingstein, Gasser, Peters, Rogelj, van Vuuren, Canadell, Cowie, Jackson, Jonas, Kriegler, Littleton, Lowe, Milne, Shrestha, Smith, Torvanger and Wiltshire2016, p. 2; Minx et al., Reference Minx, Lamb, Callaghan, Bornmann and Fuss2017, p. 2; Smith et al., Reference Smith, Haszeldine and Smith2016, p. 1400; Williamson, Reference Williamson2016, p. 155). Pointedly, Rockström et al. claim: ‘Both 2 °C and 1.5 °C targets will almost surely require implementing negative emissions technologies […] for carbon dioxide’ (Rockström et al., Reference Rockström, Schellnhuber, Hoskins, Ramanathan, Schlosser, Brasseur, Gaffney, Nobre, Meinshausen, Rogelj and Lucht2016, p. 468).
Furthermore, we agree with the claim that there is increasing potential for a ‘paradigm shift’ away from an exclusive focus on mitigation and adaption, toward possible future NET employment (Geden et al., Reference Geden, Scott and Palmer2018, p. 1), particularly as NETs have prominently been discussed in various high-level political communications. For example, in 2018, EU Commissioner Miguel Arias Cañete argued that ‘[a]s well as reducing emissions, we must also look at how to absorb emissions in order to get to zero net greenhouse gas emissions’ (EU Commission, 2018) and the German chancellor Angela Merkel stressed at the Petersburg Climate Summit in May 2019 that NETs will be needed (Kersting, Reference Kersting2019). Such a shift might further be catalyzed through phenomena such as the hot and dry summer 2018 in most of Western Europe, the ‘warmest winter season ever recorded in Europe’ in 2019/2020 (C3S, 2020) and the interest in the so-called ‘“Hothouse Earth” pathway’ (Steffen et al., Reference Steffen, Rockström, Richardson, Lenton, Folke, Liverman, Summerhayes, Barnosky, Cornell, Crucifix, Donges, Fetzer, Lade, Scheffer, Winkelmann and Schellnhuber2018, p. 1). The publication of the IPCC Special Report on 1.5 °C might serve, in the long run, as a ‘window[…] of opportunity […] which could see CDR receive greater attention’ (Geden et al., Reference Geden, Scott and Palmer2018, p. 6). Finally, a number of states are exploring or already have put into law net-zero targets by 2050, but without differentiating targets for negative emissions from targets for emissions reduction (McLaren et al., Reference McLaren, Tyfield, Willis, Szerszynski and Markusson2019).
Hence, the point of departure of this paper is that the ability to produce ‘negative emissions’ is deeply entrenched in the current thinking on how to solve the climate crisis (Carton et al., Reference Carton, Asiyanbi, Beck, Buck and Lund2020; Geden & Schäfer, Reference Geden and Schäfer2016, p. 1; Rockström et al., Reference Rockström, Schellnhuber, Hoskins, Ramanathan, Schlosser, Brasseur, Gaffney, Nobre, Meinshausen, Rogelj and Lucht2016, p. 468). However, existing technologies have been criticized to be ‘insufficiently mature to rely on them for climate stabilization’ (Fuss et al., Reference Fuss, Jones, Kraxner, Peters, Smith, Tavoni, van Vuuren, Canadell, Jackson, Milne, Moreira, Nakicenovic, Sharifi and Yamagata2016, p. 1) and several questions concerning NETs – in particular, with regard to economic, political, and social prerequisites and implications – remain unanswered to date (see Cox and Edwards, Reference Cox and Edwards2019; Geden, Reference Geden, Peters and Scott2019; Honegger and Reiner, Reference Honegger and Reiner2018; Kriegler et al., Reference Kriegler, Luderer, Bauer, Baumstark, Fujimori, Popp, Rogelj, Strefler and Van Vuuren2018; Larkin et al., Reference Larkin, Kuriakose, Sharmina and Anderson2018; Michaelowa et al., Reference Michaelowa, Allen and Sha2018; Reynolds, Reference Reynolds2018; Van Vuuren et al., Reference Van Vuuren, Stehfest, Gernaat, Van den Berg, Bijl, de Boer, Daioglou, Doelman, Edelenbosch Oreane, Harmsen Mathijs, Hof and van Sluisfeld2018). The objective of this paper is to systematize answers to some of these questions by asking what the potential geopolitical challenges, conflicts, and consequences of large-scale use of NETs are. Similar to Carton et al. (Reference Carton, Asiyanbi, Beck, Buck and Lund2020, p. 3), we thus go beyond an instrumental perspective of analyzing NETs. The relevance of this pursuit is two-fold: first, NETs – in particular land-based NETs – require physical territory – indicated already in the need to deploy them on a ‘large scale’. Without large-scale territorial deployment, several of these technologies would not have any climate-relevant effect (McGlashan et al., Reference McGlashan, Shah, Caldecott and Workman2012, p. 501). We argue that this will, in turn, lead to specific patterns of contestation. Second, building on the literature of ‘critical geopolitics’, geography can be understood as being used discursively for political identity construction. We claim that the discursive construction of space and identity which would take place in the wake of large-scale use might lead to new conflict configurations in its own right. We build on analyses of Boyd, who has developed a first set of geopolitical ranking criteria to evaluate various NETs as well as solar radiation management (SRM) measures (Boyd, Reference Boyd2016), and perspectives by Yusoff, who assesses the impact of discursive construction of ‘an engineerable earth’ on decision-making processes concerning geoengineering (Yusoff, Reference Yusoff2013, p. 2799). Furthermore, we take up the call for critically engaging with the ‘long history of carbon removal’ (Carton et al., Reference Carton, Asiyanbi, Beck, Buck and Lund2020). We combine these arguments and put them to use with regard to three negative emission technologies (NETs): afforestation, BECCS, and direct air capture and carbon storage (DACCS).
The paper proceeds as follows: first, the notion of NETs is discussed. Next, we introduce the two theoretical perspectives of ‘traditional’ and of ‘critical’ geopolitics from which we derive categories to present potential geopolitical implications of NETs in a systematic way. Third, we apply these lenses to the case of NETs in order to understand which geopolitical challenges and potential consequences a large-scale deployment of NETs might produce. As NETs have not been used on a large scale yet, we, however, first apply our scheme to existing but similar measures – namely, reducing emissions from deforestation and forest degradation (REDD+) and renewable energies (RE) – to draw inferences about the implications of NETs (afforestation, BECCS, and DACCS). We show how geopolitics in both its traditional and critical understanding may play a role in these NETs.
2. What are negative emissions technologies (NETs)?
NETs are here defined, following Fuss et al. (Reference Fuss, Jones, Kraxner, Peters, Smith, Tavoni, van Vuuren, Canadell, Jackson, Milne, Moreira, Nakicenovic, Sharifi and Yamagata2016, p. 1), as ‘any anthropogenic activities that deliberately extract CO2 from the atmosphere’. The term encompasses a number of very different approaches concerning their scope, potential, side effects, risks, and readiness (Fuss et al., Reference Fuss, Lamb, Callaghan, Hilaire, Creutzig, Amann, Beringer, Garcia, Hartmann, Khanna, Luderer, Nemet, Rogelj, Smith, Vicente, Wilcox, Dominguez and Minx2018; Honegger & Reiner, Reference Honegger and Reiner2018; Minx et al., Reference Minx, Lamb, Callaghan, Fuss, Hilaire, Creutzig, Amann, Beringer, Garcia, Hartmann, Khanna, Lenzi, Luderer, Nemet, Rogelj, Smith, Vincente, Wilcox and del Mar Zamora Dominguez2018; Nemet et al., Reference Nemet, Callaghan, Creutzig, Fuss, Hartmann, Hilaire, Lamb, Minx, Rogers and Smith2018): among them are BECCS – ‘the generation of energy from burning biomass coupled to the capture and storage of carbon dioxide […] in geological or other reservoirs’ (Fuss et al., Reference Fuss, Jones, Kraxner, Peters, Smith, Tavoni, van Vuuren, Canadell, Jackson, Milne, Moreira, Nakicenovic, Sharifi and Yamagata2016, p. 3); afforestation – an approach which is commonly considered ‘green’ but which can, applied on large scales, have grave detrimental side effects (Kreidenweis et al., Reference Kreidenweis, Humpenöder, Stevanovic, Bodirsky, Kriegler, Lotze-Campen and Popp2016); DACCS – which entails the absorption of carbon dioxide (CO2) from ambient air and subsequent storage; and other approaches such as enhanced weathering (Fuss et al., Reference Fuss, Jones, Kraxner, Peters, Smith, Tavoni, van Vuuren, Canadell, Jackson, Milne, Moreira, Nakicenovic, Sharifi and Yamagata2016, p. 3) and ocean fertilization (OF) (for a comparative assessment, see McLaren, Reference McLaren2012). In this study, in order to allow for a more fine-grained analysis, we will focus on the approaches of afforestation, BECCS, and DACCS.
For afforestation, a number of model studies have garnered results on various impacts of large-scale application. It is representative of several technologies that try to increase the absorption capacity of land-based natural sinks. DACCS, which follows a completely different logic, has been applied in small-scale field facilities already (Marshall, Reference Marshall2017) and is representative of a large-scale technical application. In addition, certain concerns voiced, for example, with regard to wind energy generation could also apply to the establishment of DACCS facilities. Although the technology is, compared to other NETs such as afforestation or BECCS, considered to be very immature (Nemet et al., Reference Nemet, Callaghan, Creutzig, Fuss, Hartmann, Hilaire, Lamb, Minx, Rogers and Smith2018) and ‘a plethora of more cost-effective and more conventional options exist’ (Fuss et al., Reference Fuss, Lamb, Callaghan, Hilaire, Creutzig, Amann, Beringer, Garcia, Hartmann, Khanna, Luderer, Nemet, Rogelj, Smith, Vicente, Wilcox, Dominguez and Minx2018, p. 19), Fuss et al. find that its potential is assumed to be very large in several NET assessments (Fuss et al., Reference Fuss, Lamb, Callaghan, Hilaire, Creutzig, Amann, Beringer, Garcia, Hartmann, Khanna, Luderer, Nemet, Rogelj, Smith, Vicente, Wilcox, Dominguez and Minx2018, p. 17).Footnote 1 Our selection of these three approaches allows us to assess very different NETs that can be considered representative of larger groups. Each is also land-based unlike, for instance, OF, which allows us to draw on lessons learned from land-based mitigation approaches (see also Carton et al., Reference Carton, Asiyanbi, Beck, Buck and Lund2020, p. 3). By assessing afforestation, BECCS, and DACCS, we hope to contribute to the discussion of each technology but also to the debate on NETs overall.
When we talk of ‘negative emissions technologies’, by ‘technology’, we mean more than just a single device or artifact, that is, more than an individual direct air capture facility – as already have been put to work, for example, in Switzerland (Speicher, Reference Speicher2017). Instead, we define technology in the sense of the socio-technical system as a ‘configuration[…] of actors, technologies [in the narrower sense] and institutions for the fulfilment of societal functions’ (Schot & Kanger, Reference Schot and Kanger2018, p. 1045). For NETs to be effective at fulfilling their societal function, namely countering CO2 emissions to the degree arguably implied by the PA, a complete set of socio-technical systems of NETs (Schot & Kanger, Reference Schot and Kanger2018, p. 1045) would be necessary (Rayner, Reference Rayner2016). This focus on scale has material consequences. However, aside from the material devices and infrastructures, ‘political cultures and coalitions, social values, world-views, and paradigms’ make up such a system (Smit, Reference Smit, Goodin and Tilly2006, p. 734), which does, so far, not exist for any of the technologies discussed. A single DACCS plant cannot be expected to make an impact on the global climate but an entire system would need to be in place, including plants, infrastructure, regulatory regimes, and actors with specific interests and knowledge. In contrast to the case of NETs, measures such as RE production or REDD+ are already based on such more mature socio-technical systems.
3. Geopolitical perspectives on NETs
The socio-technical systems of NETs are not only framed within a specific political setting (Waller et al., Reference Waller, Rayner, Chilvers, Gough, Lorenzoni, Jordan and Vaughan2020) or build on existing carbon removal techniques (Carton et al., Reference Carton, Asiyanbi, Beck, Buck and Lund2020), but might also change geopolitics. Hence, scholars such as Horton and Reynolds have asked International Relations (IR) scholars to engage in the discussion on the international (geo-)politics of climate engineering (Horton & Reynolds, Reference Horton and Reynolds2016) and, similarly, Bellamy and Palmer argue to bring ‘to bear […] geographical ideas about space, scale, power and geopolitics’ into discussions about geoengineering (Bellamy & Palmer, Reference Bellamy and Palmer2018, p. 1). But, what do we mean when we employ the term ‘geopolitics’?
Ever since Thukydides' contemplation of territorial aspects of the Peloponnesian War in the 4th century BC, all geopolitical analyses share ‘a focus on the relationship between geography and politics, in that all geography is politics and politics is always spatial’ (Guzzini, Reference Guzzini and Guzzini2012, p. 13). ‘Geopolitics’ as a term is of rather recent origin and was introduced by Rudolf Kjellén in 1899 (Ritz, Reference Ritz2013, p. 72). Geopolitical thinking at the beginning of the 20th century had a strong social Darwinist connotation, building on Malthus' theory of scarce resources resulting, in particular, in Friedrich Ratzel's notion of ‘Lebensraum’ (living space) (Reuber, Reference Reuber2012, p. 159). Furthermore, this first wave of geopolitical thinking focused on military conflicts only and derived any conclusions mono-causally from geographic circumstances (for current proponents of such deterministic geographical thinking, see Kaplan, Reference Kaplan2013; Marshall, Reference Marshall2015). Today, it is claimed that ‘geopolitics is back’ (Wetzel, Reference Wetzel2014) and there is a new focus on geopolitical themes taking account of territorial and material aspects but without falling into geographic determinism (e.g. Dodds, Reference Dodds2019; Flint, Reference Flint2017; O'Lear, Reference O'Lear2020).
Studies on the geopolitics of fossil fuels, in particular, are very common (e.g. Klare, Reference Klare2012; Yergin, Reference Yergin2020), and lately even RE technologies have been assessed through this lens (see, e.g. Austvik & Rzayeva, Reference Austvik and Rzayeva2017; Eisen, Reference Eisen2010; Fischhendler et al., Reference Fischhendler, Herman and Anderman2016; Lederer Reference Lederer2020; Scholten & Bosman, Reference Scholten and Bosman2016). Contrary to existing analyses, we are not interested in the energy security of individual states but rather apply the concept of geopolitics to NETs and their potential implications, inter alia for the security of states and other actors. The potential (geo-)political implications of these territorial requirements have been subject only of a very limited number of studies (see Boyd, Reference Boyd2016).
In our argument, we acknowledge that territory and materiality are influencing the unfolding politics of NETs but also include the socially constructive power of discourse and identity construction. We build on an interesting twist to geopolitical thinking that followed the ‘spatial turn’ in various Anglo-Saxon theories and that has been labeled, by its own thinkers, ‘critical geopolitics’. This perspective is interested in a discursive understanding of how territory, space, power, and identity are linter-linked. Geographical determinism is thus overcome and critical geopolitics becomes a form of critique of ideology in which specific ideas, perceptions, or identities are being analyzed (Dalby, Reference Dalby2013, Reference Dalby2014, Reference Dalby2020; Ó Tuathail & Agnew, Reference Ó Tuathail and Agnew1992). The spatial assumptions of classical geopolitical perspectives described above are thus criticized as a specific political representation of the world serving a specific function (Flint, Reference Flint2017; Ó Tuathail & Dalby, Reference Ó Tuathail and Dalby1998). Classical examples of such approaches would be critical reflections of the ‘West’ against ‘the rest’ or the similarly famous ‘North/ South’ divide. Of course, these critical readings build on some of the insights of more classical approaches and also problematize territory and material resource distributions. However, for heuristic purposes, we treat them as two distinct perspectives.
Thus, to identify and systematize potential geopolitical implications of afforestation, BECCS, and DACCS, we develop an analytical framework incorporating both traditional and critical perspectives in order to gain a better understanding of the various conflicts that are unfolding. The concepts of ‘space/territorial aspects’, ‘material input’, and ‘local conflict potential’ as well as ‘regional/global conflict potential’ reflect the notion of the classic geopolitical view that nature determines politics, leading to conflicts around territorial control. As nature can no longer be considered a given in the Anthropocene (Bellamy & Osaka, Reference Bellamy and Osaka2019; Dalby, Reference Dalby2020), being shaped and in some cases even constructed through human action, we also employ the categories ‘discursive strands’, ‘actor identity construction’, and ‘Anthropocene geopolitics’, in order to capture, at least as far as currently possible, the social construction of the geographical space of climate policies to identify conflicts – between states, but also between other actor types – around ideational distinctions (Yusoff, Reference Yusoff2013).Footnote 2 Drawing an analogy from the cases of REDD+ and RE allows us to pinpoint potential geopolitical implications in a situation of limited empirical data on afforestation, BECCS, and DACCS. Thus, methodically we contribute to further diversifying knowledge production approaches aiming at understanding as much as possible about the possible futures of NETs (Low & Schäfer, Reference Low and Schäfer2019), offering insights not from model runs, engagement exercises, or scenario workshops, but from past, but similar cases (see also Carton et al., Reference Carton, Asiyanbi, Beck, Buck and Lund2020 for a similar approach).
The various geopolitically relevant aspects of NETs discussed in this paper – both potential and current – were identified in this study in a three-step approach: first, the analytical framework (Figure 1) was developed on the basis of the two perspectives discussed above: classic geopolitics on one hand, and critical geopolitics on the other. Second, the framework was applied to the cases of REDD+ and RE, in order to be able to generate a blueprint of how the geopolitical profile of a developed socio-technical system of such a measure might look. Third, we assessed academic literature from various disciplines on the three NETs of afforestation, BECCS, and DACCS for insights into current and indicators for future space and material requirements, conflict potentials, discursive strands, and identity construction.

Figure 1. Analytical framework to assess geopolitical implications of REDD+, RE, and NETs.
Critics might argue that there is no need or potential for such (geo-)political consideration of NETs as long as most of these approaches – in the sense of complete socio-technical systems – do not materially exist and as long as the underlying ideas still need to be examined concerning their technical feasibilities and boundaries. In contrast, we claim that the natural science and engineering research into emerging technologies such as NETs needs to be accompanied by considerations of governance and social and (geo-)political context in order to avoid the so-called Collingridge dilemma as pointed out by technology scholar David Collingridge:
‘[A]ttempting to control a technology is difficult, and not rarely impossible, because during its early stages, when it can be controlled, not enough can be known about its harmful social consequences to warrant controlling its development; but by the time these consequences are apparent, control has become costly and slow’. (Collingridge, Reference Collingridge1980, p. 19)
In order to circumvent this dilemma, the consideration of political implications of comparatively young technologies such as NETs is vital in order to prevent their development in a way that causes grave harmful social consequences (Bellamy, Reference Bellamy2016; Foley et al., Reference Foley, Guston and Sarewitz2015; Markusson & Wong, Reference Markusson and Wong2015). This is based on the assumption that no technology is solely determined by the laws of physics which draws the boundaries of what is technologically possible, but also by social processes which shape each technology (Bijker, Reference Bijker, Goodin and Tilly2006, p. 684), for example, through political decision-making.
4. Geopolitical analogies to NETs: REDD+ and RE
For two reasons we focus on the specific cases of REDD+ and RE as potential blueprints for our geopolitical discussion of NETs: first, both have, over the last 10 years, been perceived as potentially highly effective policy-measures to contribute to a stable climate although, initially, they were often criticized as utopian pipedreams. However, both instruments have undergone a rather rapid transformation accompanied by concrete policies as well as conflicts. NETs could undergo a similar development and might be employed in an exponential fashion over the next few years. Second, both REDD+ and RE were initially discussed as if there were no trade-offs or negative externalities and when implementation started several policy-makers and academics started to realize that particularly for REDD+ but also regarding RE, there is no ‘free lunch’ (see Corbera et al., Reference Corbera, Estrada and Brown2010 or Asiyanbi & Lund, Reference Asiyanbi and Lund2020 for social implications and conflicts in the context of REDD+; Scheidel & Sorman, Reference Scheidel and Sorman2012 or Lederer, Reference Lederer2020 for trade-offs and conflicts in land-use when employing RE).
Today, it seems fair to claim that RE is overall a success story, whereas the verdict for REDD+ is much more negative as we discuss below. The early discussion of NETs resembles these developments and might allow us to understand what is to come. This is relevant as social and political negative externalities have moved further toward the center stage (see, e.g. the rise of social science articles on the issues of NETs and SRM in recent years Linnér & Wibeck, Reference Linnér and Wibeck2015, p. 258). Although the discussion of afforestation and BECCS in the context of REDD+ is, we claim, fairly straightforward, the development of geopolitical implications of DACCS from those of RE warrants further elaboration but they are also strongly interlinked as large amounts of RE will be needed to make DACCS possible. However, although DACCS is a very specific technology to draw CO2 from ambient air, RE technologies present a portfolio of different approaches to achieve a common aim, namely the generation of energy from non-finite sources. As some implications of DACCS are similar to those of solar installations, others more to those of wind energy production, we chose to stick with the comparison of the individual technology to the portfolio.
The requirement of territory of REDD+ is theoretically large as REDD+ might be employed on large parts of the territory of, for example, Brazil, the Democratic Republic of Congo, or Indonesia for forest preservation, and thus exclude various other uses of these territories. However, REDD+ is primarily focused on preserving existing forests and not on the creation of new forested areas.Footnote 3 Furthermore, no material input – except for financial means – is needed for REDD+ and diverted from other uses. However, the local conflict potential presented by REDD+ is high, as alternative uses of the forested lands are possible and various conflicts have evolved – for example, between actors from the agricultural sector, industrial logging, sustainable forest management, agroforestry, etc. (Di Gregorio et al., Reference Di Gregorio, Brockhaus, Cronin, Muharrom, Angelsen, Brockhaus, Sunderlin and Verchot2012).
Many have, thus, claimed that REDD+ should be considered a ‘hype’ (Massarella et al., Reference Massarella, Sallu, Ensor and Marchant2018) and that it failed ‘to arrest global forest loss’ as well as bring about co-benefits of any kind (Asiyanbi & Lund, Reference Asiyanbi and Lund2020, p. 380). Rather, it increased rights abuses and marginalization (ibid.; Carton et al., Reference Carton, Asiyanbi, Beck, Buck and Lund2020, p. 11f; Milne et al., Reference Milne, Mahanty, To, Dressler, Kanowski and Thavat2019). Others have been more positive arguing that, under specific circumstances, the resources employed did lead to change and that some success can be seen (e.g. Korhonen-Kurki et al., Reference Korhonen-Kurki, Brockhaus, Sehring, Di Gregorio, Assembe-Mvondo, Babon, Bekele, Benn, Gebara, Kambire, Kengoum, Maharani, Menton, Moeliono, Ochieng, Paudel, Pham, Dkamela and Sitoe2018), particularly if one focuses on the effects REDD+ had on institutions and policies (Lederer & Höhne, Reference Lederer and Höhne2019). But, also those more positive accounts stress that these changes entail conflicts and trade-offs that have to do with the territorial requirements and material aspects of REDD+ (ibid.). There is also global conflict potential concerning the question of who gets to decide what is to happen about forest-rich and highly climate-relevant territory such as tropical rainforests: Is it state territory for nation states to deal with as they please? Is it a ‘global carbon sink’ which might, arguably, be used in order to allow industrial states to maintain an unsustainable lifestyle (McAfee, Reference McAfee2016)? This became also apparent in the negotiations on forest issues at the UNFCCC Conferences of the Parties (COP) where countries of the global South were not acting in unity (Allan & Dauvergne, Reference Allan and Dauvergne2013) but some – such as for example, India – could strongly influence the outcome (Negi & Giessen, Reference Negi and Giessen2018).
To move to the perspective of critical geopolitics, the hegemonic discourse on REDD+ can be described as a nature-based solution that is carbon-centered and where forests are perceived as nothing but sinks (Bäckstrand & Lövbrand, Reference Bäckstrand and Lövbrand2006; Carton et al., Reference Carton, Asiyanbi, Beck, Buck and Lund2020). Parallel discourses have evolved and now put a stronger focus on potential co-benefits of forest preservation as well as on landscape approaches (Turnhout et al., Reference Turnhout, Gupta, Weatherley-Singh, Vijge, de Koning, Visseren-Hamakers and Lederer2016) but these can be criticized for disguising the impossibility of REDD+ to lead to transformative practices and for disguising an ecomodernist approach that will not lead to any serious changes (Asiyanbi & Lund, Reference Asiyanbi and Lund2020). Furthermore, various identities are being constructed in the context of REDD+ policies: for example, new agents on the political stage have gained global legitimacy as indigenous peoples are perceived as guardians of the common heritage of mankind and thus as agents of global politics (Schroeder, Reference Schroeder2010; Wallbott, Reference Wallbott2014) although locally they often lost out (Milne et al., Reference Milne, Mahanty, To, Dressler, Kanowski and Thavat2019). At the same time, the carbon-centered hegemonic discourse is prevalent in identity construction as well, as countries of the Global South – those rich in rainforests – are identified as a global carbon sink. This shows how classical and critical perspectives on the geopolitics of REDD+ are also closely interlinked. Thus, REDD+ might turn out to be a precursor to various NETs (Carton et al., Reference Carton, Asiyanbi, Beck, Buck and Lund2020) and be part of a turn to Anthropocene geopolitics in which humankind shapes its own environment, in particular if the focus of REDD+ should move from forest preservation to reforestation and afforestation.
Moving to the other case, RE have become a geopolitical issue (for recent overviews, see Lederer, Reference Lederer2020; Scheffran, Reference Scheffran2020). First, regarding local conflict potential, RE technologies have potentially large territorial requirements, in particular hydropower, off- and onshore wind installations, and large-scale biomass production (Global Commission on the Geopolitics of Energy Transformation, 2019; Scheidel & Sorman, Reference Scheidel and Sorman2012). Solar power production facilities have smaller requirements as long as degraded lands or used lands (rooftops) are exclusively being considered but even in this case conflicts over territory could easily evolve as various projects in California experienced (Mulvaney, Reference Mulvaney2019, chapter 5 speaks of a ‘green civil war’). Also, RE approaches use up large quantities of material, for example concrete for dams and wind power installations. Beyond that, the construction of batteries and turbines require specific input of certain materials such as rare earths (Dutta et al., Reference Dutta, Kim, Uchimiya, Kwon, Jeon, Deep and Yun2016, p. 183). The local conflict potential of RE is, therefore, very dependent on the specific technology applied. The potential is, however, generally high whenever alternative land use is possible in the case of dams, biomass, and solar or wind power installations. Local protests indicate conflicts around issues of trust and control over decision-making concerning installations and infrastructure (Friedl & Reichl, Reference Friedl and Reichl2016; Galvin, Reference Galvin2018).
Regional and global conflict potential is expected to be somewhat lower than that of fossil fuel-based energy provision as RE is more open to decentral production (Paltsev, Reference Paltsev2016, p. 390). This potential might, however, increase with growing reliance on RE (Johansson, Reference Johansson2013, p. 202) and particularly, if ‘power to gas’ applications become mainstream. In the latter case, the export of hydrogen through pipelines from Russia to the EU or via ship from Australia to Japan, China, or Europe are considered as possibilities that could bring about geopolitical tensions (Westphal et al., Reference Westphal, Dröge and Geden2020). We might, thus, see new alliances that form adding another layer to the geopolitics to the energy overall (e.g. Scheffran, Reference Scheffran2020). Finally and very materially, geopolitical challenges have increased in the context of rare earths which are produced only in very specific locations across the globe (Kiggins, Reference Kiggins2015; Sovacool et al., Reference Sovacool, Ali, Bazilian, Radley, Nemery, Okatz and Mulvaney2020; Wilson, Reference Wilson2017). Beyond this, the possibility of a new resource curse in RE or critical material-producing countries warrants attention (O'Sullivan et al., Reference O'Sullivan, Overland, Sandalow, Begg, Behrens, Bhatiya, Clark, Cremer, Elkind, Fessler, Lemphers, Nakagawa, Seol, Soylu and Vakulchuk2017, pp. v–vi).
Discursively, RE is often viewed as a silver bullet of ecological modernization to avoid dangerous climate change. ‘Ecological modernization’ denotes a discourse in which environmental challenges are accepted as real and threatening, but can be addressed through existing structures of politics and economic action: it ‘suggests that the recognition of the ecological crisis actually constitutes a challenge for business [as it] open[s] up new markets and create[s] new demands […]’ (Hajer, Reference Hajer1997, pp. 31–32). Further discursive strands present RE as an instrument to achieve energy autonomy (Chaiyapa et al., Reference Chaiyapa, Esteban and Kameyama2018), to strengthen national industries (Schmidt et al., Reference Schmidt, Schmid and Sewerin2019), and/or to create more decentralized, potentially even more democratically controlled energy provision (Sarrica et al., Reference Sarrica, Biddau, Brondi, Cottone and Mazzara2018; Scholten & Bosman, Reference Scholten and Bosman2016). In some cases, RE production is part of national climate leadership and has become a trademark for the country's energy policy and this again proves that ideational and material aspects are closely intertwined. For example, Germany has often been presented and presents itself as a frontrunner in RE employment (Jänicke, Reference Jänicke, Wurzel and Connelly2011) and this led the government to push hard, for example, RE subsidies and feed-in tariffs in various countries while at the same time was part of Germany soft-power stance on climate change issues (Steinbacher & Pahle, Reference Steinbacher and Pahle2016). Regionally, RE has given new impetus toward the European Energy Union and its geopolitical ambitions, for example, in the context of the Crimea crisis (Lederer, Reference Lederer2020). Globally, green markets are presented as potential candidates to eventually fulfill the promise of sustainable development and represent a new hegemony. Thus, RE can be understood as the core of Anthropocene geopolitics in the realm of energy transformation as they have the potential to substantially change the relationship of humans with naturally given territory (Dalby, Reference Dalby2016). This implies loosening the tight connection between energy supply and geographical location characterizing fossil fuel energy production – only where the geography provides fossil fuel resources, these can be unearthed – and allowing for a wider space of human decision-making concerning energy supply (Mecklin, Reference Mecklin2016, p. 377; Paltsev, Reference Paltsev2016, p. 390).
Our discussion shows that a geopolitical reading of current measures like the large-scale introduction of RE and of REDD+ is not only possible, but also quite revealing (see Table 1). On the one hand, we can see that some geopolitical concerns will most likely not materialize (e.g. large-scale global conflicts due to REDD+ projects or RE deployment). On the other hand, it is evident that certain material aspects (e.g. critical materials in the case of solar and wind power) might cause geopolitical problems within specific sectors or localities. Furthermore, we would claim that also ideational aspects become apparent, in both the cases of RE (e.g. the strong focus on Germany as a leader in the roll-out of RE or the general hype about RE as being the most important part of an ecological modernization approach) as well as with REDD+ where a new discourse on sinks and nature-based solutions has taken off. On the basis of this first rough sketch, we summarize our discussion of the geopolitics of RE and REDD+ and start considering potential geopolitical implications of NETs.
Table 1. Overview of geopolitical implications of REDD+ and RE
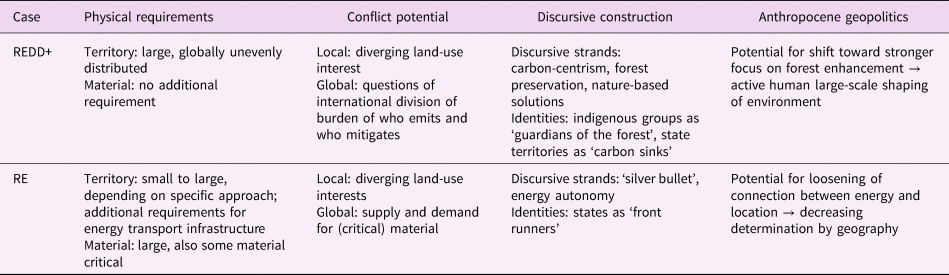
5. The geopolitics of NETs
Modeling studies have found that the two land-based CDR approaches afforestation and BECCS would require large amounts of territory in order to have any kind of relevant impact on global carbon budgets. In this, they are very similar to REDD+ schemes. Williamson (Reference Williamson2016) claims that achieving a limitation of global average temperature rise to 2 °C with BECCS only would require arable land of ‘about half the land area of the United States’ (Williamson, Reference Williamson2016; see also Burns & Nicholson, Reference Burns and Nicholson2017). Again similar to REDD+, this amount of land would primarily be located in the global South (Shaw & Marien, Reference Shaw and Marien2021).
Afforestation measures in line with remaining below 2 °C warming above pre-industrial levels would, even under a scenario of ‘considerable (Representative Concentration Pathway 4.5 […]) emissions reductions’ (Boysen et al., Reference Boysen, Lucht, Gerten, Heck, Lenton and Schellnhuber2017, p. 1), require the conversion of either ‘>1.1 Gha of the most productive agricultural land […] or ~ 1.5 Gha of natural land’ into biomass plantations (Boysen et al., Reference Boysen, Lucht, Gerten, Heck, Lenton and Schellnhuber2017, p. 6). Large-scale afforestation would imply the elimination of substantial parts of either food production or biodiversity (IPCC, 2018, p. 19). The impact on food production, in particular – not only from land-use changes, but also from irrigation and fertilizer needs of NET plantations (Boysen et al., Reference Boysen, Lucht, Gerten, Heck, Lenton and Schellnhuber2017, p. 1) – can, in turn, be expected to spark both local and regional conflicts (Carmenza et al., Reference Carmenza, Hans-Jörg, Göran, Simon, Esteve, Felix and Pete2017; Kreidenweis et al., Reference Kreidenweis, Humpenöder, Stevanovic, Bodirsky, Kriegler, Lotze-Campen and Popp2016; Shaw & Marien, Reference Shaw and Marien2021). This might, in particular, be the case where alternative use of land or resources is possible, as can be witnessed already in conflicts about bioenergy plantation projects (see, e.g. Arevalo et al., Reference Arevalo, Ochieng, Mola-Yudego and Gritten2014; Gerber, Reference Gerber2011). Further regional or even global conflict potential is inherent in the possible impact of large-scale application of afforestation on global food prices (Azar, Reference Azar2011; Boysen et al., Reference Boysen, Lucht, Gerten, Heck, Lenton and Schellnhuber2017; Kreidenweis et al., Reference Kreidenweis, Humpenöder, Stevanovic, Bodirsky, Kriegler, Lotze-Campen and Popp2016, pp. 311–312). In future projections, when not only the state of current global land use, but also projections of global population growth and subsequent increases in land demands – for example, for agriculture – as well as rapid global urbanization are taken into account (Canadell & Schulze, Reference Canadell and Schulze2014; Creutzig, Reference Creutzig2017; Creutzig et al., Reference Creutzig, Bren D'Armour, Weddige, Fuss, Beringer, Gläser, Kalkuhl, Steckel, Radebach and Edenhofer2019; Smith et al., Reference Smith, Gregory, Van Vuuren, Obersteiner, Havlík, Rounsevell, Woods, Stehfest and Bellarby2010), the conflict potential can be expected to increase even further.
Beyond these land-use challenges facing both afforestation and BECCS, large-scale BECCS is characterized by at least two further geopolitically relevant prerequisites which it shares in common with DACCS (see below): a functional infrastructure (which requires space and security) and suitable carbon storage space. Concerning the latter, although assessments indicate that enough suitable storage space is in existence globally (Dooley, Reference Dooley2013), these spaces are by far not distributed evenly across regions or nation-states (see, e.g. Kelemen et al., Reference Kelemen, Benson, Pilorgé, Psarras and Wilcox2019). Concerning the former, in order for BECCS to function, infrastructure is needed that allows for the capturing and storing of carbon produced through the burning of biomass. Also, the political geography of the territory plays a role as political instability can affect the ongoing functioning of the infrastructure, thus threatening the successful capturing of carbon (Backhaus et al., Reference Backhaus, Gausling and Hildebrand2015).
At this point, it is not yet evident in which locations large-scale BECCS installations might be set up, but it is doubtful that this will happen exclusively in areas of consolidated statehood with a high degree of good governance. And even if this requirement is met, it is at least questionable whether large-scale carbon sequestration schemes will not meet with civil opposition in areas of the world which, historically, did not contribute substantially to greenhouse gas emission and that are now predominantly farmed by smallholders. For example, even in scenarios that limit the bioenergy potential of regions according to agricultural and nature conservation requirements, Beringer et al. (Reference Beringer, Lucht and Schaphoff2011, p. 307) find that South America would be ‘responsible for about a quarter of the total bioenergy potential’, followed by Eastern and Southern Asia as well as Africa. This is already being framed as a new and potentially highly conflictive trade-off or ‘the coming scramble’ that developing as well as developed countries will face internally as well as at a global level (Toensmeier & Garrity, Reference Toensmeier and Garrity2020). Finally, even if one only focuses on developed countries, BECCS would only be feasible to a certain extent due to limited availability of suitable storage sites and high transaction costs that arise from transportation of biomass and/or CO2 (Baik et al., Reference Baik, Sanchez, Turner, Mach, Field and Benson2018). Experiences with pilots where CCS has been tried show that local acceptance is a major issue (Aminu et al., Reference Aminu, Nabavi, Rochelle and Manovic2017; Arning et al., Reference Arning, Offermann-van Heek, Linzenich, Kaetelhoen, Sternberg, Bardow and Ziefle2019) and it is thus of not much surprise that only 19 BECCS demonstration projects existed at the end of 2019 (Shaw & Marien, Reference Shaw and Marien2021) and civil society groups are clearly voicing their opposition to NETs in general and BECCS in particular (Shaw & Marien, Reference Shaw and Marien2021; Thanki, Reference Thanki2019).
Concerning a critical geopolitical perspective on BECCS and afforestation, one discursive strand relevant to both these approaches can be expected to be centered around carbon, because these approaches are defined as activities aimed at extracting CO2 from the atmosphere. The carbon-centered discourse is strengthened by the inclusion of these approaches in climate scenarios that can allow for remaining below a warming of 2 °C (Bastin et al., Reference Bastin, Finegold, Garcia, Mollicone, Rezende, Routh and Crowther2019; IPCC, 2014, pp. 12–13). However, some approaches support non-carbon co-benefits such as the production of energy or the increase of soil productivity which might take precedence in the discourse in, for instance, agricultural or forest policy communities. The consideration of effects of carbon extraction approaches on planetary boundaries other than climate change (Rockström et al., Reference Rockström, Steffen, Noone, Persson, Chapin, Lenton, Scheffer, Folke, Schellnhuber, Nykvist, de Wit, Hughes, van der Leuuw, Rodhe, Sörlin, Snyder, Costanza, Svedin, Falkenmark and Foley2009, p. 472; Steffen et al., Reference Steffen, Rockström, Richardson, Lenton, Folke, Liverman, Summerhayes, Barnosky, Cornell, Crucifix, Donges, Fetzer, Lade, Scheffer, Winkelmann and Schellnhuber2018) (e.g. on biodiversity) would be another possible discourse (Heck et al., Reference Heck, Donges and Lucht2016, p. 793). Furthermore, the issue of CCS has already raised tensions at the international level and politicized the negotiations (Carton et al., Reference Carton, Asiyanbi, Beck, Buck and Lund2020, p. 7; Krüger, Reference Krüger2017) – a trend that BECCS will most likely undergo as well. One way in which the discourses of afforestation and BECCS differ, however, is the relationships to the notion of nature: afforestation is often considered ‘green’, whereas ‘BECCS has been associated with large-scale industrial agriculture and modern technology’ (Bellamy & Osaka, Reference Bellamy and Osaka2019).
It is important to highlight that there might be various dominant discursive strands in parallel depending on the respective communities. Dedicated analyses of various relevant communities will garner very interesting insights into this early stage of the discourse when the discussion slowly moves beyond academia. For instance, an article by Bastin et al. (Reference Bastin, Finegold, Garcia, Mollicone, Rezende, Routh and Crowther2019) on afforestation was much discussed in popular newspapers such as the Guardian and the German news site Spiegel online. Furthermore, the IPCC ‘performed an important legitimating function for the speculative technology of BECCS, pulling it into the political world […]’ (Beck & Mahony, Reference Beck and Mahony2018, p. 4). The future will show whether this has resulted in ‘making previously unthinkable notions – such as overshoot and negative emissions – more mainstream and acceptable’ (Beck & Mahony, Reference Beck and Mahony2018, p. 4). Inside of the academic sphere, currently various discursive streams exist alongside each other. For instance, ‘competing judgements of BECCS's feasibility’ exist ‘between the IAM [integrated assessment modelling] community and its critics’ (Low & Schäfer, Reference Low and Schäfer2020; see also Carton et al., Reference Carton, Asiyanbi, Beck, Buck and Lund2020 for the politics of carbon removal in the context of the IPCC). Furthermore, similar to REDD+, the NET discourse might evolve from being carbon-centric to include co-benefits due to political pressure that can be expected to come from civil society groups, particularly as BECCS is rather decried as ‘wishful thinking’ by some influential civil society organizations (Ernsting & Munnion, Reference Ernsting and Munnion2016). However, afforestation and BECCS, again just like REDD+, offer the potential for identity construction of individuals and groups tending for forests as ‘guardians of the climate’ to some degree. It is thus no surprise that several of the ideas of the Green New Deal in the USA also make use of CCS projects (Cohen, Reference Cohen2019). This could very well turn out to evolve as a new push for BECCS.
BECCS also provides an insightful example of Anthropocene geopolitics. By relying on a resource that can, at least in theory, be available across large portions of the globe, it has the potential to turn the geopolitical logic of fossil fuel generation on its head. In contrast to fossil fuel sources, which exist in some nation-states and not in others, the production of biomass is much less concentrated locally although it is evident that most BECCS projects will be located in the global South. Thus, through BECCS, humans can, to a larger degree than with fossil fuels, manipulate where to produce the resources necessary for energy generation. This, in turn, influences the geopolitics of BECCS as the geopolitical interactions concerning BECCS can be expected to be much less about specific, very small territories (such as crude oil reservoirs in Africa or the Middle East; OPEC, 2016, p. 24) and much more about the availability of resources necessary for biomass production such as water and fertilizer (Shaw & Marien, Reference Shaw and Marien2021).
DACCS makes use of chemicals instead of biological processes to sequester carbon directly from the atmosphere (NAS, 2015, p. 67). In contrast to approaches capturing carbon directly from the source of emission (carbon capture and storage, CCS), DACCS filters carbon from ambient air, where it resides in much lower concentrations (Lackner & Brennan, Reference Lackner and Brennan2009, p. 360). Consequently, DACCS can counteract emissions not only from large, stationary point sources such as factories, but also from smaller, mobile sources such as automobiles or airplanes. However, much larger amounts of space are required in order to capture the same amount of carbon as CCS (NAS, 2015, p. 68): ‘Building a DACCS plant that captures 1 Mt CO2 yr−1, requires a significant surface area for the contactor alone – on the order of 38,000 m2 for 75% capture’ (Fuss et al., Reference Fuss, Lamb, Callaghan, Hilaire, Creutzig, Amann, Beringer, Garcia, Hartmann, Khanna, Luderer, Nemet, Rogelj, Smith, Vicente, Wilcox, Dominguez and Minx2018, pp. 19–20) although overall costs and efficiency concerns are likely to be more relevant for companies who are engaged in this nascent business.
Furthermore, for the captured carbon to be stored, suitable geological storage formations need to be established, which also takes up space (Minx et al., Reference Minx, Lamb, Callaghan, Fuss, Hilaire, Creutzig, Amann, Beringer, Garcia, Hartmann, Khanna, Lenzi, Luderer, Nemet, Rogelj, Smith, Vincente, Wilcox and del Mar Zamora Dominguez2018). The estimation of global storage capacity is complicated due to ‘incomplete and inconsistent’ regional assessments (Kearns et al., Reference Kearns, Teletzke, Palmer, Thomann, Keshgi, Chen, Paltsev and Herzog2017, p. 4697). However, even if globally enough storage space was deemed to exist in potentia to store CO2 sequestered through CCS (Dooley, Reference Dooley2013), these potentials are, to current knowledge, distributed unevenly regionally (see, e.g. Kelemen, et al., Reference Kelemen, Benson, Pilorgé, Psarras and Wilcox2019). This might cause ‘storage bottlenecks’ which could limit the potential of capturing CO2 for specific regions (Fuss et al., Reference Fuss, Lamb, Callaghan, Hilaire, Creutzig, Amann, Beringer, Garcia, Hartmann, Khanna, Luderer, Nemet, Rogelj, Smith, Vicente, Wilcox, Dominguez and Minx2018, p. 11). In a scenario of large-scale use of DACCS, it might also present a cause for local, but also regional or global conflict, for example if CO2 storage burdens – and associated safety and space challenges – are distributed unevenly. This conflict potential is well-known at the local level in studies on RE projects (see, e.g. Yenneti & Day, Reference Yenneti and Day2016). The challenge of finding appropriate storage space and, geopolitically, to decide which potential storage spaces are actually to be used for carbon storage, presents itself to both large-scale DACCS applications and BECCS (see above). Direct material input is assumed to be low for DACCS approaches, as the sorbent used to absorb CO2 from the air can be regenerated and then put to use several thousand times, as small-scale laboratory experiments indicate (McGlashan et al., Reference McGlashan, Shah, Caldecott and Workman2012, p. 502).
Beyond direct material and space needs, secondary space needs are presented by the energy requirements of DACCS, which are considered a ‘key issue’ (Fuss et al., Reference Fuss, Lamb, Callaghan, Hilaire, Creutzig, Amann, Beringer, Garcia, Hartmann, Khanna, Luderer, Nemet, Rogelj, Smith, Vicente, Wilcox, Dominguez and Minx2018, p. 16) in the development and implementation of this approach (see also McLaren, Reference McLaren2012; Realmonte et al., Reference Realmonte, Drouet, Gambhir, Glynn, Hawkes, Köberle and Tavoni2020). Energy requirements include ‘energy for releasing CO2 from the sorbent, regenerating the sorbent, for fans and pumping, as well as for pressurizing CO2 for transportation’ (Fuss et al., Reference Fuss, Lamb, Callaghan, Hilaire, Creutzig, Amann, Beringer, Garcia, Hartmann, Khanna, Luderer, Nemet, Rogelj, Smith, Vicente, Wilcox, Dominguez and Minx2018, p. 16). The amounts of energy required for generation and regeneration of sorbents vary for different types of sorbents that could be used for DACCS (Chatterjee & Huang, Reference Chatterjee and Huang2020; Realmonte et al., Reference Realmonte, Drouet, Gambhir, Glynn, Hawkes, Köberle and Tavoni2020). These energy requirements are the more relevant if DACCS is intended to be applied on large scales. Chatterjee and Huang (Reference Chatterjee and Huang2020) find that ‘to reach the capacity of capturing 30 Gt-CO2/yr […]’, material production and regeneration energy requirements would amount to between almost half of total global energy supply to far more than 100% of this number, depending on the solvents applied as well as other factors.
The energy production required to keep DACCS facilities running would take up substantial amounts of space if RE sources such as wind or solar are considered – which makes sense from a climate change perspective. Powering DACCS facilities through excess electricity generated by the existing RE infrastructures (Wohland et al., Reference Wohland, Witthaut and Schleussner2018) might offer a way forward, restricted by, for example, the amount of excess electricity thus collected as well as issues of cost per ton of CO2 avoided (Daggash et al., Reference Daggash, Patzschke, Heuberger, Zhu, Hellgardt, Fennell, Bhave, Bardow and MacDowell2018). For this, however, the RE infrastructure in question has to be reliable and resilient.
Local conflict potential of DACCS facilities can be expected to be similar to that of RE installations. This could be reduced if, as Goldberg and Lackner (Reference Goldberg and Lackner2015, p. 239) propose, facilities are located, together with wind turbines or solar panels to generate energy, in remote locations at large igneous provinces which can be used to store the captured CO2 (Goldberg & Lackner, Reference Goldberg and Lackner2015, p. 238). However, large-scale human manipulation of locations far away from human property and population might interfere with the protection of biodiversity (Creutzig et al., Reference Creutzig, Bren D'Armour, Weddige, Fuss, Beringer, Gläser, Kalkuhl, Steckel, Radebach and Edenhofer2019). Also, the material and labor requirements to build a DACCS facility would make ‘the siting of DACCS plants of significant scale in remote locations challenging’ (Fuss et al., Reference Fuss, Lamb, Callaghan, Hilaire, Creutzig, Amann, Beringer, Garcia, Hartmann, Khanna, Luderer, Nemet, Rogelj, Smith, Vicente, Wilcox, Dominguez and Minx2018, p. 20). The regional conflict potential of DACCS can be assumed to be low as the approach does not use up large amounts of space or materials on a regional scale. Globally, however, conflict over the distribution of burdens to be borne in order to counteract anthropogenic climate change is conceivable if, as in the case of REDD+, some countries perceive themselves to be used as carbon sinks for the benefit of other states' economic development.
The discourse on DACCS, similarly to other specific NETs, is very limited to date and takes place in rather specified communities. A distinct hegemonic discourse or social construction of DACCS is hard to pinpoint. Discourse and similar analyses have, so far, often assessed the discussion on geoengineering approaches as a whole (i.e. both NETs and SRM approaches) (see, e.g. Anshelm & Hansson, Reference Anshelm and Hansson2014; Boettcher, Reference Boettcher2019; Kreuter, Reference Kreuter2021; Linnér & Wibeck, Reference Linnér and Wibeck2015; Luokkanen et al., Reference Luokkanen, Huttunen and Hildén2014; Porter & Hulme, Reference Porter and Hulme2013), discussed NETs as a group (see, e.g. Colvin et al., Reference Colvin, Kemp, Talberg, De Castella, Downie, Friel, Grand, Howden, Jotzo, Markham and Platow2019; Markusson et al., Reference Markusson, McLaren and Tyfield2018) or specific NET groups (Cox et al., Reference Cox, Spence and Pidgeon2020, Reference Cox, Boettcher, Spense and Bellamy2021), or focused on SRM approaches (see, e.g. Macnaghten & Szerszynski, Reference Macnaghten and Szerszynski2013). Discussing both SRM and NETs as ‘geoengineering’ is criticized by Lomax et al., who argue that, instead, NETs ‘can in fact form a valuable complement to emissions control within on-going mitigation efforts’ (Lomax et al., Reference Lomax, Workman, Lenton and Shah2015, p. 125). Markusson et al. (Reference Markusson, McLaren and Tyfield2018) warn against overestimating the potential of NETs to complement mitigation when they argue that ‘the scope of NETs to substitute for mitigation may be easily exaggerated, and thus that the risk of harm from mitigation deterrence should be taken seriously’ (Markusson et al., Reference Markusson, McLaren and Tyfield2018, p. 1). If a coherent, dominant discourse on NETs such as DACCS or the enhancement of carbon sinks (see Carton et al., Reference Carton, Asiyanbi, Beck, Buck and Lund2020) fosters mitigation deterrence, this can also be geopolitically significant as geopolitical tensions arising from conflict over fossil fuel resources could, in this way, be continued over longer term.
The identification of a hegemonic discourse on DACCS is difficult. However, various concerns and ideas are noted in the literature which, if not discussed as a discourse, nevertheless warrant attention and possibly play a role in constructing meaning. In assessments of DACCS, cost is one of the foremost considerations, as the approach is rather expensive when compared to other NETs – at least when contemporary technology is applied (Fuss et al., Reference Fuss, Lamb, Callaghan, Hilaire, Creutzig, Amann, Beringer, Garcia, Hartmann, Khanna, Luderer, Nemet, Rogelj, Smith, Vicente, Wilcox, Dominguez and Minx2018; Keith, Reference Keith2009). However, the cost might be reduced through efficiency increases. Other issues contrast to the register of cost: DACCS is considered potentially ‘more benign than CCS, as fossil fuels are not involved’ (Nemet et al., Reference Nemet, Callaghan, Creutzig, Fuss, Hartmann, Hilaire, Lamb, Minx, Rogers and Smith2018, p. 14). However, although not directly coupled to fossil fuels, according to an assessment modeling study, an unintended effect of DACCS might be to extend the use of fossil fuels as the pressure for mitigation measures might decrease (Chen & Tavoni, Reference Chen and Tavoni2013). Lackner et al. discuss further concerns that might become relevant about environmental impacts of storage of CO2 sequestered as well as storage safety – as CO2 gas concentrations above 5–10% of air volume are lethal in humans (Lackner & Brennan, Reference Lackner and Brennan2009, p. 368) – and liability in situations of failure, that is, leakage. These conflicting notions of a generally positive image of the abstract approach on one hand and apprehensions against the specific deployment of the approach in the vicinity on the other are shared by several RE approaches, specifically wind power generation (Christidis et al., Reference Christidis, Lewis and Bigelow2017; Galvin, Reference Galvin2018; Schwenkenbrecher, Reference Schwenkenbrecher2017) as well as by analyses of CCS (see above). A survey study found that the perception of DACCS might be much more positive if it is embedded in a ‘green town’ concept where the DACCS facility is fully integrated into the community (Cheng et al., Reference Cheng, Fürth, Johnson, Tay, Shenoi and Wilson2013). This might change the perception present in academic publications so far, that DACCS is discussed as being ‘“deployed”, rather than “adopted”’ (Nemet et al., Reference Nemet, Callaghan, Creutzig, Fuss, Hartmann, Hilaire, Lamb, Minx, Rogers and Smith2018, p. 18). Also, the framing of the approach seems significant: a framing highlighting similarity or closeness to natural processes seems to support a more favorable view on the approach than a framing highlighting relevant industrial processes (Corner & Pidgeon, Reference Corner and Pidgeon2015). It remains to be seen whether one discursive understanding of DACCS – be it the idea of a silver bullet to the climate problem, or that of a cheap life-support system for the fossil fuel industry (Mitchell, Reference Mitchell2009), or that of artificial trees (Corner & Pidgeon, Reference Corner and Pidgeon2015), or that of an integral part of the modern city – will become dominant in the discourse and whether this dominant view, in turn, will influence how societies choose to act.
Identity construction is also difficult to assess concerning DACCS so far. Some notions are studied which present researchers, public, and private actors in diverging lights, for example, on a spectrum between neutral or selfless actors supporting the development of benign technology on the one side and actors following their own vested, personal interests on the other side of the spectrum (Jacobsen, Reference Jacobsen2018). If the framing proposed by Lomax et al. (Reference Lomax, Workman, Lenton and Shah2015) and the similarities between DACCS and RE move further center-stage, identity constructions and the discursive strands on DACCS might shift and, in turn, become more strongly similar to that of RE, presenting DACCS-deploying states as frontrunners in the attempt to counter negative effects of climate change. Finally, DACCS might have an impact on ‘remote’ areas, that is, locations of, so far, little human interference or it might influence the conceptualization of the city if the concept of the ‘green town’ where air is being constantly ‘cleaned’ by DACCS technologies is applied large scale.
Overall, we claim that taking up geopolitical perspectives on NET technologies has been a fruitful undertaking as, even though these technologies have not been deployed on a large scale, the potential social and political consequences become clearer (see Table 2). Whether these consequences should be considered as too severe in comparison with the consequences that other mitigation scenarios or the use of for example, SRM might bring, is ultimately a political question that politicians and citizens will have to confront rather sooner than later. We would claim that the abovementioned geopolitical aspects should not be neglected but rather be at the center stage of any such decision-making processes.
Table 2. Overview of geopolitical implications of afforestation, BECCS, and DACCS

6. Discussion and conclusion
We find that, first, afforestation and BECCS would have a classic geopolitical impact due to their requirements of territory for biomass growth which is known, already, from REDD+. This would lead to national and international conflict potential over who should provide the territory required to counter past CO2 emissions – which, in turn, were created while benefitting the industry and economy of some states and not others. In a similar vein, both DACCS and BECCS – like wind energy – present conflict potential ranging from the local to the global scale over who should bear the burden of setting up facilities. In the case of DACCS and BECCS, additionally, the focus can be expected to be more strongly on safety issues due to the need to store CO2 sustainably. Second, the material requirements of afforestation and BECCS – most prominently in fertilizers and water supply – might present local or regional conflict potential over the use of these resources when it comes to conflicts of aims between CO2 drawdown and, for example, food production. Third, the discursive construction of space and identity which would take place in the wake of large-scale deployment might lead to very interesting new conflict patterns in its own right. A first indication in this direction is the potential for conflicting discursive representations of abstract concepts of DACCS (and possibly BECCS) on the one hand and of concrete facilities in the vicinity on the other, a conflict which has also been apparent in the discussion on wind energy installations. Fourth, BECCS and RE share the potential to turn geopolitics on its head – at least to some degree. Both could loosen the connection between energy generation and geographic location – without, of course, severing it completely.
To sum up, afforestation and BECCS pose challenges due to their high land use and material requirements and subsequent conflicts over what to use these resources for and who will benefit from its use. It is, however, unclear, at this point, which actors would be motivated to pursue large-scale afforestation or BECCS. This might change if sequestered carbon gains a relevant price tag and thus market actors gain an interest in setting up the instrument – a story well known from REDD+ that also in the end will only have a large-scale impact as a ‘results-based payment’ scheme (Turnhout et al., Reference Turnhout, Gupta, Weatherley-Singh, Vijge, de Koning, Visseren-Hamakers and Lederer2016). The latter, however, would further undermine the attempt to gain any co-benefits leading to a trade-off that has neither been solved in the international negotiations nor on the ground. This would most likely also occur in the case of NETs. Furthermore, DACCS requires large amounts of energy and might thus be placed in conjunction with RE. This could foster existing land-use conflicts posed by RE installations, possibly providing them with a global dimension when it comes to selecting suitable carbon storage spaces which are distributed unevenly around the globe. Other energy generation approaches are conceivable, such as nuclear, but this, in turn, poses its own set of requirements of suitable locations for production and disposal, an issue that is certainly also highly conflictual. Specifically, DACCS shares the burden of RE approaches such as wind energy generation that the general and abstract notion of its use is considered benign, but the concrete deployment in the vicinity meets with local resistance. Furthermore, increasing carbon pricing, emerging national or international negative emissions policies, and/or policy entrepreneurship from interested parties – such as private companies whose business model is based on carbon sequestration – could lead to the development of socio-technical systems of BECCS or DACCS. This, in turn, might affect the geopolitical relevance of specific territories but also lead to specific identity constructions. For instance, remote locations with carbon storage and RE generation potential (for DACCS) or fertile lands with carbon storage potential (for BECCS) would increase in importance materially but also as spaces for green deals etc. could eventually materialize. This would cause a shift in the balance of the current geopolitical constellation the consequences of which cannot, so far, be known.
In a nutshell, land use, resource conflicts on local and global scales, and the discourses surrounding them are common staple in international relations and they concern territory as well as space. Several of the conflict potentials opened by NETs are known from RE or REDD+ and it can be expected that the social construction of the space for NETs, which, so far, takes place only in very limited, mainly academic circles (Buck, Reference Buck, Methmann, Rothe and Stephan2013; Matzner & Barben, Reference Matzner, Barben, Janich and Rhein2018), will follow similar lines as the discourses on RE, REDD+, and other environmental and even other global issues and be straddled with several of the same challenges as current mitigation strategies. The geopolitics of NETs might have just begun.
Data
All relevant information is available within the manuscript.
Acknowledgments
We thank the participants of the workshop ‘The Politics and Governance of Negative Emissions Technologies. Between the Paris Agreement and the Anthropocene’ on June 15–16, 2017, at Utrecht University, The Netherlands, as well as the participants of the panel ‘The Politics of Negative Emissions’ at the EASST 2018 Conference on July 25–28, 2018, at Lancaster University, UK, for their insightful feedback on earlier versions of this article. In particular, we thank Jesse Reynolds and Emily Cox for their invaluable feedback.
Author contributions
Judith Kreuter and Markus Lederer conceived and designed the study, conducted research, and wrote the article.
Financial support
We acknowledge support by the Deutsche Forschungsgemeinschaft (DFG – German Research Foundation) and the Open Access Publishing Fund of Technical University of Darmstadt. The presentation of this work at the workshop ‘The Politics and Governance of Negative Emissions Technologies: Between the Paris Agreement and the Anthropocene’ in June 2017 in Utrecht was support by a travel grant provided by COST action INOGOV (468 euros). This research received no other specific grant from any funding agency, commercial, or not-for-profit sectors.
Conflict of interest
Judith Kreuter and Markus Lederer declare none.