Introduction
Although the geomorphic activity of snow avalanches is extensively described (for example Reference RappRapp, 1959; Reference LuckmanLuckman, 1977), little attention is given to what are here termed avalanche tarns. Good examples of these small lakes, which are located at the base of avalanche tracks, are found in the Fiordland region of New Zealand (Fig. 1). The purpose of this paper is to describe these features in more detail as they have been examined in only a few cases, with those from Norway probably being the best known (Reference CornerCorner, 1980). They also represent an extreme form of avalanche impact. Little is known about the dynamics of such events, although Reference Perla, Perla, Beck and BannerPerla and others (1978) have measured the impact forces of snow falling vertically from a height of 10 m in small controlled experiments, and Reference SchaererSchaerer (1962) presents equations for the impact forces of avalanches that jump a natural bench to land on a snow shed.

Fig. 1. The Fiordland region of New Zealand and location of avalanche tarns.
Previous Descriptions
Repeated avalanche impact may produce small depressions, sometimes filled by water, which have been called avalanche scour pits (Reference DavisDavis, 1962), or avalanche pits (Serbenko in Reference PeevPeev 1966). They describe these forms as ranging up to 300 m2 in area and 9 m deep. Reference LiestølLiestøl ([1974]) notes circular crater-shaped ridges of debris thrown out of small lakes by the explosive effects of avalanche impact. In the same way, Reference DavisDavis (1962), when describing an avalanche scour pit on the floor of Grouse Meadow in the Kings River basin of California, presumed a snow avalanche had tumbled down the mountain and shoved soil outward and forward to form a rim; then snow melted and left behind a depression filled with water. Serbenko noted that the pits are ellipsoid in shape and are slightly inclined on their outer side towards the valley and that this was to be explained by the upheaval of the soil during avalanche impact.
Reference CornerCorner (1980) found that most of the avalanche impact landforms of northern Norway are located below long avalanche chutes or gullies. He noted that three morphological varieties occur: (a) avalanche-impact tongues, which are debris accumulations on the distal banks of streams; (b) avalanche-impact pits, which are water-filled depressions; and (c) avalanche-impact pools, which are submerged depressions occurring in large water bodies such as fiords or lakes. The avalanche tarns described here are similar to the avalanche pits of Corner, and were first noted in the Fiordland region by Reference SmithSmith (1947).
Fiordland Avalanche Terrain
The Fiordland region has been deeply glaciated, so that avalanches originating in the high cirques and shelf areas plunge down steep, convex slopes to the valley floors below (Fig. 2). Precipitation is high, averaging 6236 mm/year at Milford Sound, and 6747 mm/year at Wilmot Pass (New Zealand Meteorological Service, 1973). These two factors combine to produce avalanches that occur along the valleys with a density of 160/km year (Reference SmithSmith, 1947).
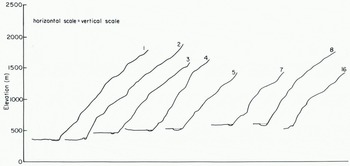
Fig. 2. Profiles of some avalanche paths with tarns.
The avalanche-starting zones of Fiordland have characteristics that are similar to those elsewhere. Of 41 avalanche paths that cross the Milford Road, the average area is 0.1 km2 with a range from 0. 008 to 0.861 km2, and the average slope is approximately 40°, ranging from 29° to 59°. Almost half originate on areas of permanent snow. Most starting zones are above 1500 m a.s.l, an elevation which often marks the lip of the glacial trough, and extend up to 2500 m a.s.l. (Reference Fitzharris and OwensFitzharris and Owens, 1980).
In contrast, the tracks appear to differ significantly from those reported from many other avalanche areas, undoubtedly as a result of the strongly over-steepened glacial valley slopes. For example, the mean slope of the 41 tracks is over 50°, much steeper than that for the San Juan Mountains, U.S.A. (Reference Miller, Miller, Armstrong and ArmstrongMiller and others, 1976), and most tracks are usually steeper than starting zones.
The run-out zones generally consist of steep fans or bedrock areas, but some are flat river beds especially in the larger, more extensively glaciated valleys. Vegetation patterns often show the effects of extensive wind blast and of direct snow impact. Because of the moderating influence exerted on the climate by the nearby Tasman Sea, it is unusual for the run-out zones to be snow covered, even in winter, when the snow-line usually fluctuates about an elevation of 1000 m a.s.l.
Avalanche Tarns in Fiordland
Eleven avalanche tarns were recognized from field inspection, either from a helicopter or on the ground, with a further five identified from topographic maps (Fig. 1. Table I). In total about 10000 km2 of south-west New Zealand was searched. The tarns tend to be centred around the most heavily glaciated part of Fiordland, with many easily observed from the Milford highway or from the Milford Track. Despite the continuation of glaciated terrain into southern Fiordland, they are less common there because the mountains become lower, and receive less snow. Likewise, although high and glaciated terrain continues to the north and east, there are no avalanche tarns because the bedrock geology changes from resistant granites, gabbros, and gneisses, which favour steeper slopes, to softer alpine schists and lower-grade metamorphic rocks. These erode more readily and form valleys with less spectacular walls.
TABLE I. Fiordland Avalanche Tarns
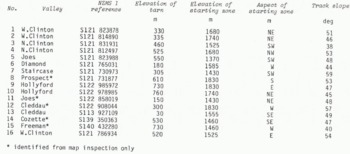
The tarns have a variety of shapes. Some are circular, while others are elongated parallel or at right angles to the valley. They vary from minor lakes of dimension 300 m × 150 m (No. 7), to small ponds 20 m × 10 m (No. 16). The largest avalanche tarn covers 50000 m2 and the smallest 200 m2. Since the mean area of the tarns listed in Table I is 11000 m2 (standard deviation 16000), they are much larger than the five avalanches pits described by Reference CornerCorner (1980), who notes that the biggest and morphologically most striking pit in north Norway is Rundvatnet, an almost circular avalanche tarn about 8000 m2 in area. However, his largest avalanche pools are bigger, being c.200–300 m across, or up to 70000 m2 in area.
The Fiordland avalanche tarns occur on the valley floors, so occupy a range of elevations. All are located close to valley sides at the base of avalanche paths with slopes ranging from 38–59°. Those shown in Figs 2–6 are good examples.
Vertical profiles of some of the avalanche paths were compiled from those areas mapped at a scale of 1 : 14000 (Fig. 2), and a contour map of the avalanche path and tarn at Hidden Lake, a popular lunch place for walkers on the Milford Track, is shown in Figure 3. While the slopes of the tracks are steep by most standards, and steeper than the 15–40° reported for avalanche impact pits by Reference CornerCorner (1980), they are not unusual for Fiordland. Likewise, the elevations of the starting zones are similar to other avalanche paths of the region, ranging from 1370 m to 1740 m a.s.l, and having a wide variety of aspects. Thus the reason why a few avalanche paths produce avalanche tarns, but most do not, is not immediately obvious from their morphological characteristics.
Formation of Avalanche Tarns
There is good evidence to support the notion that the tarns described in Table I are a product of avalanche impact. They all occur near the base of steep tracks, where there is an abrupt change of slope with the valley floor. Patterns of damage and vegetation succession radiate from the base of the avalanche tracks. In 1982, tarn No. 2 of Table I, known locally as “the swimming pool”, was struck by a large avalanche which destroyed vegetation over an area of about 10000 m2, and by its impact pushed up gravel debris as concentric ridges on the valley side of the tarn (Fig. 5). These observations are consistent with those of impact pits reported from elsewhere by Reference DavisDavis (1962), Reference LiestølLiestøl ([1974]), and Reference CornerCorner (1980). At the nearby Hidden Lake (Figs 3 and 4), similar, but older features are found on a larger scale, together with a radiating series of smaller tarns. When combined with the concentric patterns of vegetation, the evidence is consistent with that caused by ejecta flung outwards by the impact of large avalanches (Fig. 4). An avalanche fell into Hidden Lake in September 1983 and caused such a rapid evacuation of the water that the bridge over its outlet stream on the Milford Track was washed away.
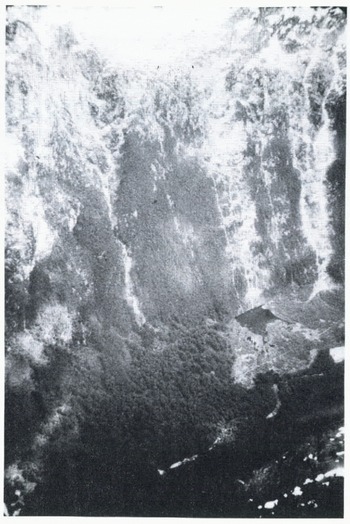
Fig. 4. Avalanche tarn called Hidden lake which is No. 1 of Table I. Note the debris free bedrock slope which continues to the level of the tarn and the radiating patterns of lesser tarns and vegetation. The Milford Track is the transverse line below Hidden Lake.
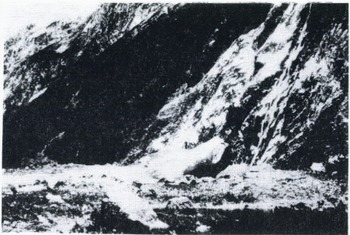
Fig. 5. Photograph showing avalanche snow and tarn at the “Swimming pool” (No. 2 of Table I). Together with damage caused by an avalanche the previous winter.
Where a cone of avalanche snow frequently occurs, as near to tarn No. 3 (Fig. 5), a small rampart of debris can develop, as detritus rolls down and collects at its edge. When the snow melts, a small depression enclosed by the rampart and the valley wall may be created, which could fill with water. However the evidence from this site suggests this is not the mechanism for the formation of the tarns, because the location of the snow cone and the tarn are not the same.
There are two possible hypotheses as to why tarns form beneath only a small fraction of avalanche paths in the Fiordland region: (a) the profiles of paths with tarns favour the production of airborne avalanches which plunge directly to the valley floor with sufficient impact and frequency to excavate a depression; (b) while airborne avalanches are common in Fiordland (Reference SmithSmith, 1947), they do not usually produce impact depressions or pits because their force is dissipated by oblique impact on fan deposits lying against the base of the valley wall. A necessary condition for their formation is an abrupt break of slope at the valley floor. Those examined in the field were characterized by continuation of a debris-free bedrock slope to the level of the tarn, as illustrated in Figs 4–6.

Fig. 6. Avalanche path in north branch of Clinton Valley illustrating steep nature of the track and tarn at its base (No. 4.)
Thus avalanche tarns only occur where there is little fan debris, either because it has been removed by the main river, or because the avalanche path produces little detritus for fan building. The latter possibility could be due to different rock types within the avalanche path, except that the tarns occur within at least four distinct rock lithologies ranging from igneous to metamorphic. Alternatively, debris produced in the avalanche-starting zones could be trapped in its snow-fields and not reach the valley floor. However an examination of starting zones in summer does not support this idea.
Snow avalanches can possess great destructive power with studies on impact pressures indicating probable values of 10 to 600 kN/m2 (Reference Mellor and VoightMellor, 1978; Reference Lang and BrownLang and Brown, 1980). Reference Shimizu and ShimizuShimizu and others (1980) report larger impact pressures of up to 1400 kN/m2 in the Kurobe Canyon of Japan, for high-speed avalanches (velocities of at least 60 m/s). From measurements with load cells, Reference Schaerer and SalwaySchaerer and Salway (1980) consider that the initial peak pressure P on a unit surface perpendicular to the direction of avalanche flow may be expressed as:

where c is a coefficient dependent on the deformation of the snow (in turn a function of temperatures, free-water content, initial density, and the type and bonding of the snow grains), ρ the density of the avalanche snow, and v the avalanche speed.
If v = 60 m/s, ρ = 150 kg/m3, and c = 1.5 for large Fiordland avalanches, then P = 1080 kN/m2. Plunging avalanches strike the valley floors at angles of at least 38° (Table I, Fig. 2) so that the impact pressures would be P sin 38° = 665 kN/m2 or larger. Reference SmithSmith (1947) describes a large avalanche near to tarn No. 10 of Table I, in which 280000 tonnes of snow fell 440 m, apparently in free fall, into the Hollyford Valley below. At a point 130 m from impact, it destroyed a reinforced concrete structure built to protect the portal of a tunnel. The force at the structure is calculated by Smith to exceed 167 kN/m2. Impact pressures at the base of some Fiordland avalanche paths where there is an abrupt change of slope and rapid deceleration, may well exceed these values, and thus appear to be sufficient to cause the impact pits occupied by avalanche tarns.
Because the avalanche tracks in Fiordland are very steep, and the avalanches sometimes fall free, the validity of Equation (1) is uncertain. There appear to be few observations and little theory of the dynamics of plunging avalanches. As Reference SchaererSchaerer (1962) points out, a jumping avalanche would also cause a vertical moving load and a friction load. In addition, the influence of high water pressures as the avalanche violently expels the contents of the tarn could be considered.
Conclusions
The avalanche tarns in the Fiordland region of New Zealand are large and are distributed about the most heavily glaciated part of the region. They occur at the base of slopes steeper than 38° where there is an abrupt change of angle with the valley floor. Avalanche tarns are only found where deep glacial erosion in resistant rocks has created convex avalanche-path profiles which allow snow to plunge directly onto the valley floor. The evidence of concentric mounds of debris, together with radial bands of minor tarns, indicates that they are the product of violent avalanche impact. A necessary condition for their formation is that there is little or no fan debris to deflect the impact of the avalanche. Impact pressures have yet to be measured for large plunging avalanches of the type that appear to have produced the tarns described here, but could indicate upper limits to the destructive power of falling snow. The dynamics of such events also needs further study.