Introduction
Shiga toxin-producing Escherichia coli (STEC) serogroup O157 is a zoonotic bacterial pathogen that colonises the gastrointestinal tracts of large ruminants such as cattle and sheep. Transmission to humans occurs through the faecal–oral route via contaminated food or water, direct animal contact, environmental exposure or person-to-person spread [Reference Launders1, Reference Byrne2].
Clinical presentation ranges from mild gastrointestinal symptoms to more severe illness involving bloody diarrhoea, abdominal pain, vomiting and fever. In a subset of patients, infection can progress to haemolytic uremic syndrome (HUS), a systemic condition characterised by haemolytic anaemia, thrombocytopenia, kidney malfunction and sometimes cardiac and/or neurological complications. STEC-HUS is the most common cause of renal failure in children in the UK [Reference Adams3, Reference Lynn4].
The key virulence factor is Shiga toxin, of which there are two types, Stx1 and Stx2. Since 1990, phage typing has been used at Public Health England (PHE) to type STEC O157. Phage typing is rapid and cost effective and has proven utility for detection of outbreaks, differentiating strains and demonstrating trends.
In England, STEC O157 is a notifiable infection, and in 2009, enhanced surveillance was established, requiring public health follow-up on every reported case infection. For the duration of the period of this study, the PHE national enhanced STEC surveillance system mainly focused on STEC O157 because this serogroup was perceived to be the cause of the highest burden and most severe clinical outcomes of all STEC serogroups in the UK. For each case, a risk assessment based on clinical presentation and an enhanced surveillance questionnaire (ESQ) is undertaken.
Despite a range of interventions implemented throughout the entire UK over the last four decades in catering, retail and meat hygiene sectors, the numbers of cases of STEC O157 in England between 1997 and 2012 remained constant, averaging 887 cases per year [Reference Adams3]. In this study, we describe the epidemiology of STEC O157 infection in England, by exploring the microbiological and clinical characteristics, the demographic and geographical distribution of cases and examining changes in environmental exposures over 11 years of enhanced surveillance.
Methods
Laboratory methods
In England, all faecal specimens from patients presenting to primary healthcare with suspected gastrointestinal infection are submitted to local hospital laboratories and tested for a range of gastrointestinal pathogens, including E. coli O157, following the UK Standards for Microbiology Investigations (https://www.gov.uk/government/collections/standards-for-microbiology-investigations-smi). Isolates identified as presumptive STEC O157 are then submitted to the PHE Gastrointestinal Bacteria Reference Unit (GBRU) for confirmation by PCR targeting stx1 and stx2, and further typing, including phage typing; both methods have been described previously [Reference Frost5, Reference Jenkins6]. Data from laboratory referral forms and microbiological typing results are entered and stored in the PHE laboratory information management system.
For patients with severe illness for whom faecal specimens are unavailable, serodiagnosis was used for the duration of this study to identify antibodies to the lipopolysaccharides of E. coli O157 [Reference Chart and Jenkins7].
National enhanced surveillance
The detection of presumptive STEC O157 infection in faecal samples is reported by local laboratories directly to PHE centres (PHEC), responsible for health protection. Each PHEC arranges for the STEC ESQ to be administered to patients. The ESQ collects data in the following categories: demographic details; risk status; clinical condition (including progression to HUS); household or other close contact details; exposures including travel, food and water consumption, contact with animals, and environmental exposures; case classification; outbreak status.
Completed questionnaires are then forwarded for inclusion in the National Enhanced Surveillance System for STEC (NESSS), which compiles these data with microbiological data from GBRU based on patient identifiable information. NESSS is managed by the Gastrointestinal Pathogens Unit within the PHE National Infection Service.
Epidemiological case definitions
Non-outbreak primary: A symptomatic case with no history of close contact with a confirmed case in the 7 days prior to onset of illness.
Non-outbreak co-primary: A symptomatic case within a group of close contacts with no history of close contact with a separate confirmed case in the 7 days prior to onset of illness.
Non-outbreak secondary: A case with a date of onset more than 4 days after the primary case or where transmission is believed to be through exposure to a primary case.
Non-outbreak unsure: It is not possible to determine whether the case is primary or secondary with the information available. This may be because the patient was lost to follow-up, is asymptomatic or where the chain of transmission is unclear.
Outbreak-associated: A case microbiologically and epidemiologically confirmed as part of an outbreak.
Travel-related: A case who reported being outside of the UK for any of the 7 days before onset of symptoms.
Data handling
Data on case demographics, exposures and microbiological typing were extracted from NESSS based on the receipt date of the isolate at GBRU.
For incidence rate calculations, mid-year population estimates for 2009–2019 by age, sex and lower super output area (LSOA) were obtained from the ONS website: https://www.ons.gov.uk/peoplepopulationandcommunity/populationandmigration/populationestimates/datasets/populationestimatesforukenglandandwalesscotlandandnorthernireland. The 2011 rural–urban classifications were obtained from the Open Geography Portal (https://geoportal.statistics.gov.uk/) also managed by the ONS.
Data manipulation was conducted using R version 3.6.3. Incidence rates and confidence intervals were calculated using Byar's method [Reference Breslow and Day8]. Confidence intervals for rate ratios were calculated using the Wald method.
Results
Cases reported to NESSS 2009–2019
From 2009 to 2019, 8295 cases of STEC O157 were reported to national surveillance, including 8082/8295 culture-confirmed cases and 213/8295 cases confirmed by serodiagnosis. Of these, 1472/8295 (18%) were defined as outbreak cases. ESQs were available for 8041/8295 of cases, of which 1706/8039 (21%) reporting having travelled outside of the UK for at least one of 7 days prior to symptom onset. Of the cases with ESQs, 6852/8041 (85%) were classified as primary or co-primary cases and 682 as secondary cases. For the other 507/8041 cases, an epidemiological definition could not be assigned (‘unsure’) (Fig. 1).

Fig. 1. Breakdown of cases reported to NESSS from 2009 to 2019.
Over the 11-year period, the number of STEC O157 cases reported to national surveillance decreased, with the mean cases per year dropping from 887 for the period 2009–2014, to 595 for the period 2015–2019. While the number of cases attributed to outbreaks has fluctuated over the years and travel-related cases remain stable, the decline has been most striking amongst non-outbreak cases with domestically acquired infection (Fig. 2).

Fig. 2. Cases with evidence of STEC O157 infection by epidemiological category.
The number of outbreaks per year decreased from 2009 to 2019, although the median number of cases per outbreak fluctuated. With the exception of 2013, from 2009 to 2014, there were more than 10 outbreaks per year. From 2015, there were 10 or fewer outbreaks per year, dropping to as low as four outbreaks in 2018 and 2019. Conversely, the median number of cases per outbreak in 2018 and 2019 were higher than previous years, except 2014 (Fig. 3).

Fig. 3. Number of outbreaks and median number of cases per outbreak by year.
Microbiological subtypes
Of 8082 culture-confirmed cases, 5286 (65%) were infected by STEC O157 harbouring stx2 only, decreasing from 696/954 (73%) of cases in 2009 to 322/515 (63%) in 2019. In contrast, the proportion of cases infected with STEC O157 that had both stx1 and stx2 increased from 257/954 (27%) to 190/515 (37%) over the same timeframe (Fig. 4).

Fig. 4. Cases with culture-confirmed STEC O157 infection by stx profile.
The majority of STEC O157 belonged to either PT8 (2586) or PT21/28 (1933) accounting for 4519/8082 (56%) of culture confirmed cases. The decline in STEC O157 has been largely driven by a decrease in PT21/28 with a mean of 244 cases per year in the period 2009–2014 as compared to a mean of 94 cases per year in the period 2015–2019. There has been a slight increase in PT54, from a mean of 36 cases per year from 2009 to 2014, to a mean of 45 cases per year from 2015 to 2019 (Fig. 5).

Fig. 5. Percentage of culture-confirmed cases per year by phage type.
Incidence
From 2009 to 2014, the incidence rate of STEC O157 was 1.66 cases per 100 000 person-years (95% CI 1.62–1.71). In the period between 2015 and 2019, the incidence rate dropped to 1.07 cases per 100 000 person-years (95% CI 1.03–1.11).
From 2009 to 2019, STEC O157 incidence was highest among children between 1 and 4 years old, with a rate of 5.33 cases per 100 000 population (95% CI 4.96–5.72) among females and 5.31 cases per 100 000 population (95% CI 4.95–5.69) among males. There was no apparent difference by sex under the age of 20 years; however, incidence was significantly higher among females in age groups between 20 and 79 years (Fig. 6).

Fig. 6. Incidence rate of STEC O157 infection per 100 000 population per year by age group and sex between 2009 and 2019.
Comparing the periods of 2009–2014 and 2015–2019, incidence decreased across all age groups, but the greatest decreases were among children: Incidence in 1–4 years old dropped 48% (RR = 0.52, 95% CI 0.56–0.58) and 59% in 5–9 years old (RR = 0.41, 95% CI 0.36–0.47). Among adults, the greatest decrease was among 60–69 years old, dropping 41% (RR = 0.59, 95% CI 0.50–0.70) (Fig. 7).

Fig. 7. Incidence rate of STEC O157 infection per 100 000 population per year by age group and time-period between 2009 and 2019.
Rurality
Two cases were excluded due to invalid postcodes. Comparing the period 2009–2014 with 2015–2019, the incidence in both rural and urban areas decreased. However, the decrease was larger in rural areas, dropping 44% (RR = 0.56, 95% CI 0.51–0.62). In urban areas, incidence decreased 33% (RR = 0.67, 95% CI 0.64–0.71). While the association with PT8 incidence and rurality was equivalent across the time periods 2009–2014 and 2015–2019, for PT21/28 the magnitude of the association weakened (RR = 2.14, 95% CI 1.92–2.40; to RR = 1.86, 95% CI 1.51–2.27) (Table 1).
Table 1. Rate ratios comparing rural to urban LSOAs by phage type and time period
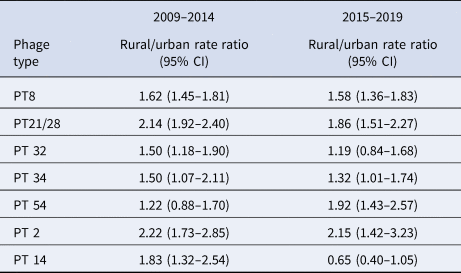
Demographics
Among 8295 cases with evidence of STEC O157 infection, the median age was 22 years (IQR: 6–47 years) and 4660 (56%) were female. Among female cases, the median age was 26 years, and among males, 17 years. Males and females between 1 and 4 years old were consistently the most-affected groups from 2009 to 2014, comprising 562/5321 (11%) and 534/5321 (10%) of cases respectively. However, from 2015 to 2019, the proportion of cases that were 20–29 years old females rose to 289/2974 (10%), while the proportion that were males and females 1–4 years old dropped to 246/2974 (8%) and 237/2974 (8%), respectively (Fig. 8).

Fig. 8. Proportion of cases by time period in each age–sex group.
Age–sex by Stx profile
Cases infected with Stx2-only strains were mostly female (2893/5287, 55%), and predominantly children between 1 and 4 years old (1238/5287, 23%). Cases infected with stx1 + stx2 strains had a higher female proportion (1618/2751, 59%) and were predominantly females aged 20–29 years old (286/2751, 10%). There were only 44 stx1-only strains, which were predominantly adolescents and adult females (Fig. 9).

Fig. 9. Percentage of cases by age and sex within each Stx profile.
Age–sex by phage type
STEC O157 belonging to PT21/28 infected the highest proportion of children between 1 and 4 years old (506/1933, 26%), whereas the majority of PT34 and PT8 strains infected adults 20 years and older (327/494, 65% and 1645/2586, 64%). Eleven per cent (53/494) of PT 34 cases were males between 1 and 4 years old, but only 30/464 (6%) were females in that age group, whereas 44/494 (9%) cases were females 20–29 years old. PT8 cases were most heavily concentrated in females 20–29 years old (251/2586, 10%), with only 314/2586 (12%) cases in the 1–4 years age group (Fig. 10).

Fig. 10. Percentage of cases by age and sex within each of the most frequent phage types.
Clinical features
ESQs were completed for 8041/8295 (97%) individuals with evidence of STEC O157 infection. Of the 8041 with ESQs, 7598 (94%) reported being symptomatic. Asymptomatic individuals were identified during contact tracing and targeted sampling of contacts of a symptomatic case, specifically children under the age of 6, food handlers, healthcare workers and vulnerable people.
Of 7598 symptomatic cases with evidence of STEC O157 infection, 2597 (34%) cases were admitted to hospital, and 348 (5%) developed HUS. Of the 1040 cases for whom duration of hospitalisation was available, the median duration was 3 days (IQR: 1–4). Among the 348 cases with HUS, 163 (47%) were under 5 years of age.
Comparing the time periods 2009–2014 and 2015–2019, the proportion of cases reporting diarrhoea and abdominal pain increased, while the proportion of cases that developed HUS decreased (Table 2).
Table 2. Percentage of cases reporting various clinical features by time period

Of 8041 cases with ESQs, cases between 10 and 19 years of age had the highest proportion of bloody diarrhoea, reported by 364/506 (72%) of males in that age group and 346/494 (70%) of females. Cases in this age group also had the highest proportion of hospitalisation, as 226/506 (45%) of males and 210/494 (43%) of females were hospitalised.
Female cases 1–4 and 5–9 years old had the highest proportion of HUS (91/749, 12%, and 44/467, 9% respectively). Male cases of the same age had a slightly lower proportion: 64/783 (8%) among 1–4 years old, and 32/480 (7%) among 5–9 years old (Fig. 11).

Fig. 11. Percentage of cases with ESQs in each age group by indicator of severe illness.
Of 7864 culture-confirmed cases with ESQs, severe illness was most frequently reported in cases with PT21/28 and PT2 strains. Of 1859 cases with PT21/28 stx2 strains, 1249 (67%) reported bloody diarrhoea, 798 (43%) were hospitalised, and 144 (8%) developed HUS. Of 361 cases with PT2 stx2 strains, 268 (74%) reported bloody diarrhoea, 158 (44%) were hospitalised, and 25 (7%) developed HUS.
In cases infected with the most common PT, PT8, bloody diarrhoea was as commonly reported for stx1+2 strains (1458/2164, 67%) as in PT21/28 stx2-only strains (1249/1859, 67%), but hospitalisation and HUS were reported by a smaller proportion (Table 3).
Table 3. Percentage of cases reporting indicators of clinical severity by the 10 most detected phage types

a Stx1-only strains not included due to low numbers (<20 cases for any phage type).
Travel
Of 8041 cases with ESQs, 1706 (21%) reported any travel outside of the UK during the 7 days before onset of illness. From 2009 to 2014, 978/5140 (19%) cases reported travel as compared to 728/2901 (25%) of cases from 2015 to 2019.
Adult cases were more likely than child cases to have travelled during the incubation period, and female cases were more likely to report any travel abroad (1054/4531, 23%) than male cases (652/3510, 19%).
Among the most frequently reported phage types, the proportion of PT8 cases reporting travel increased from 400/1597 (25%) in the 2009–2014 period to 293/933 (31%) in the 2015–2019 period, with the most frequent destinations being Turkey, Spain and Malta, followed closely by Egypt. PT21/28 cases had the lowest proportion of reported travel (Table 4).
Table 4. Proportion of cases reporting travel by the top 10 phage types among culture-confirmed cases

Animal contact
ESQs were available for 6852 primary or co-primary cases. There was some evidence (P = 0.06) that proportion of cases reporting contact with any animal increased over time, driven in an increase in contact with dogs, reported by 1384/4261 (32%) of cases between 2009 and 2014 reporting contact with a dog to 929/2591 (36%) from 2015 to 2019. Direct contact with farm animals decreased as did farm visits (606/4261, 14% to 285/2591, 11%). However, the percentage of cases reporting living on or access to a private farm increased, from 112/4261 (3%) in 2009–2014, to 193/2591 (7%) in 2015–2019 (Table 5).
Table 5. Animal contact and exposure by time period

Discussion
Since the introduction of enhanced microbiological detection of STEC O157 in England in 1996, the number of cases identified remained relatively stable over the following two decades [Reference Byrne2, Reference Adams3]. However, over the 11-year period of this study, the incidence of STEC O157 has followed a downward trend [9]. This decrease has been reported in Scotland as well, with a 43% decrease in cases from 2014 to 2019 [10, 11].
In England, while the annual number of cases associated with outbreaks fluctuated and the number of travel-related cases remained stable, the annual number of non-outbreak-associated, domestically acquired infections decreased. The decline in STEC O157 infections appears to be mirrored by the decrease in cases infected with PT21/28, the phage type which almost exclusively possesses stx2 only, and showed a marked decreased between 2009 and 2019. Comparing 2009–2014 with 2015–2019, the proportion of strains harbouring stx2 only decreased, while the proportion of strains harbouring stx1 and stx2 increased.
The decrease in STEC O157 being pronounced in a specific phage type may suggest changes in behaviours and/or exposure risks. Evidence presented here and in previous studies has shown that PT21/28 is most commonly associated with children in the 1–4 years age group, cases resident in rural areas and with environmental exposures [Reference Launders1, Reference Byrne2]. There is some evidence from the analysis of outbreak data that outbreaks of PT21/28 are more likely to be associated with the consumption of contaminated meat or unpasteurised dairy products than produce [Reference Byrne12–Reference Treacy14]. While changes in the patterns of food consumption and food safety improvements in the meat and dairy industries may be contributing factors, we may be witnessing a decline in the prevalence of PT21/28 in the animal reservoir, as supported by data from a recent study [Reference Henry15]. Strain replacements in the UK cattle population have been documented previously [Reference Dallman16, Reference Yara17]. In the 1980s, PT2 was the most frequently detected STEC O157 subtype among human cases but numbers decreased rapidly in the 1990s when PT21/28 and PT8 became more prevalent [Reference Adams3].
Cases infected with STEC O157 PT21/28 more frequently resulted in severe clinical outcomes, including HUS [Reference Launders1]. A previous study during the same time period suggests that severity is associated with the presence of the Shiga toxin subtype Stx2a, which is hosted on a bacteriophage harboured by this PT [Reference Byrne18, Reference Byrne19]. Although the percentage of cases that developed HUS decreased, the percentage of cases reporting bloody diarrhoea and hospitalisation remained stable, contributing to a growing body of evidence that other factors such as possessing multiple subtypes of stx genes [Reference Byrne19] or harbouring stx1a [Reference Vishram20] may contribute to severity. Consequently, while the incidence of STEC O157 with PT21/28 has decreased, and with it the proportion of HUS cases, STEC O157 PT8 stx1+2 strains appear to maintain the proportion of cases reporting bloody diarrhoea or hospitalisation.
From 2009 to 2019, the number of outbreaks decreased though the median number of cases per outbreak increased. In the context of overall decreasing incidence, fewer outbreaks occurred, though more refined typing methods linked more cases to each outbreak, with the routine use of molecular typing from 2013 and the implementation of whole genome sequencing in 2015 facilitating outbreak case ascertainment [Reference Dallman21].
The routine collection of enhanced epidemiological data following the implementation of NESSS in 2009 has enabled us to monitor trends in demography, exposures and clinical outcomes for over a decade [Reference Launders1, Reference Byrne2, Reference Byrne18, Reference Byrne19, Reference Adams22, Reference Elson23]. There is evidence that a decrease in the prevalence of the highly pathogenic STEC O157 PT21/28 in the animal reservoir appears to have had a direct impact on the overall burden of STEC O157 in England. Although the risk to children developing STEC-HUS caused by STEC O157 may have decreased, hospitalisations in all age groups remain high compared to other common gastrointestinal pathogens [Reference Brien24]. Enhanced surveillance data can support detection of emerging strain types and novel sources and transmission routes [Reference Byrne18, Reference Vishram20, Reference Kaindama25]. Integration of epidemiological data with microbiological typing data is essential to understanding the changes in the burden of STEC infection, assessment of the risks to public health, and the prediction and mitigation of emerging threats.
Acknowledgements
We would like to thank all diagnostic laboratories in the National Health Service for referral of specimens and isolates and our colleagues in GBRU for their microbiological expertise. We would also like to acknowledge Bhavita Vishram, Nalini Purohit, Mike Harte, Thomas Thackray and Sanjay Chaudhry for their contributions to NESSS and to all public health practitioners who report to the scheme.
Financial support
This study is funded by the National Institute for Health Research (NIHR) Health Protection Research Unit in Gastrointestinal Infections, a partnership between the UK Health Security Agency, the University of Liverpool and the University of Warwick. The views expressed are those of the author(s) and not necessarily those of the NIHR, the UK Health Security Agency or the Department of Health and Social Care.
Conflict of interest
None.
Data availability statement
The data that support the findings of this study are provided within this manuscript. If required, additional data may be available from the corresponding author with the permission of PHE. Restrictions apply to the availability of these data, which were used under licence for this study.