Diabetic nephropathy (DN) is one of the most serious microvascular complications of diabetes and a major cause of end-stage renal disease( Reference Zimmet, Alberti and Shaw 1 ). Diabetes mellitus (DM) was the fifth cause among the top ten causes of death in Taiwan in 2010. According to the 2004–8 Nutrition and Health Survey in Taiwan (NHST), the prevalence of DM was 9·2 %, and haemodialysis treatment being required by one-fourth to one-third of the patients is caused by DN.
Systemic hypertension leads to glomerular hypertension, renal microvascular complications and glomerular basement membrane thickening and accelerates renal failure. Renin and angiotensins released from the kidneys during kidney injury lead to elevated blood pressure. These factors may form a vicious cycle and aggravate renal dysfunction. About 50 % of the patients who suffered from type 1 DM for more than 30 years had hypertension( Reference Chukwuma 2 ). The German Society for Diabetes has also suggested that adjusting the blood sugar levels to a near-normal range and normalising the blood pressure are beneficial for preventing DN.
Hyperglycaemia may also lead to haemodynamic changes( Reference Ziyadeh and Wolf 3 ) and excretion of angiotensin II (Ag II)( Reference Dronavalli, Duka and Bakris 4 ). Both hyperglycaemia and elevation of Ag II levels induce the formation of reactive oxidative species. Exposure of podocytes to high glucose levels leads to an increase in reactive oxidative species generation and is associated with the up-regulation of cytochrome P450 4A (CYP4A) expression, and CYP4A inhibitors can ameliorate proteinuria in DM mice( Reference Eid, Gorin and Fagg 5 ). In addition, nephrin is a podocyte-specific protein and is a component of the filtration slits in the kidneys. In DN, hyperglycaemia and high Ag II levels are related to the dysregulation of nephrin expression and proteinuria( Reference Doublier, Salvidio and Lupia 6 , Reference Liebau, Lang and Bohm 7 ).
Modification of dietary habits is important in DM treatment. Soyabean is the main source of dietary plant protein source in Oriental diets. Previous studies have shown that soya protein has several health-promoting effects( Reference Takamatsu, Tachibana and Matsumoto 8 ), such as improvement of glucose tolerance, insulin sensitivity and hyperlipidaemia( Reference Banz, Davis and Peterson 9 ) and retardation of the progression of hypertension and renal dysfunction( Reference Yang, Chen and Chang 10 ). β-Conglycinin, a major storage protein of soyabeans, has angiotensin-converting enzyme (ACE)-inhibitory activity( Reference Kuba, Tanaka and Tawata 11 ) and can attenuate blood glucose and insulin levels in obese mice( Reference Moriyama, Kishimoto and Nagai 12 ). However, the effects of β-conglycinin on DN remain unclear. In addition, soya isoflavones are also considered to be another bioactive component of soya protein. Therefore, the aim of the present study was to investigate the effects of β-conglycinin and soya isoflavones on renal function, blood glucose levels and blood pressure in a rat model of streptozotocin (STZ)-induced DN.
Materials and methods
Animals, diets and experimental design
Male spontaneously hypertensive rats (SHR, 8 weeks old) were purchased from the National Laboratory Animal Breeding and Research Center. The rats were housed in individual cages in a room maintained at 23 ± 1°C with 55 ± 5 % humidity and a 12 h light–12 h dark cycle. Investigators at the Taipei Medical University Laboratory Animal Center followed the protocols described in the ‘Guide for the Care and Use of Laboratory Animals’. The rats were fed a standard rat chow diet (Rodent Laboratory Chow 5001; Purina Mills) for 1 week for acclimatisation. β-Conglycinin was purchased from Fuji Oil. Diabetes was induced in the rats by an intravenous injection of 25 mg/kg of STZ (dissolved in pH 4·5 citrate buffer), while the control rats were administered an equal volume of a vehicle. After 48 h, blood samples were collected from the tail vein, and rats with a fasting glucose concentration >2000 mg/l were divided into three groups: DN rats fed the AIN-93M diet (D group, n 6); DN rats fed the AIN-93M diet containing β-conglycinin (one-eighth of casein was replaced by β-conglycinin as the dietary protein source, B group, n 10); DN rats fed the AIN-93M diet containing soya isoflavones (equivalent to the isoflavone content in the B group, I group, n 9). The control rats were fed the AIN-93M diet (C group, n 8; Table 1). During the 4-week experimental period, food was provided with free access. The body weight and food intake were recorded daily. At the end of the experimental period, all the rats were killed, and blood and tissue samples were collected for analysis.
Table 1 Diet composition
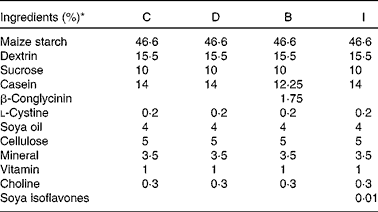
C, control group; D, diabetic nephropathy (DN) group; B, DN+β-conglycinin group; I, DN+soya isoflavone group.
* Casein (high N), cellulose (non-nutritive bulk), mineral mixture (AIN-93M) and vitamin mixture (AIN-93M) were obtained from ICN Biochemicals. Dextrin was obtained from Corn Products International, Inc. β-Conglycinin was procured from Fuji Oil Company Limited, and it contained 57 mg/100 g of isoflavones. Soya isoflavones were obtained from Corn Products Korea, Inc. Soya oil and sucrose were obtained from the Taiwan Sugar Corporation.
Sample collection and analysis
Blood pressure
Systolic blood pressure and diastolic blood pressure were measured by the tail-cuff method (MK-2000ST; Muromachi Kikai). After being starved for 12 h, the rats were put into restrainers, and at least five readings were recorded to calculate the blood pressure.
Blood samples
The rats were killed after being anaesthetised with sodium pentobarbital. Blood samples were collected from the interior vena cava into tubes containing an anticoagulant. Blood samples were immediately centrifuged, and the plasma was stored at − 80°C until being analysed. Plasma glucose, insulin, blood urea N, creatinine, uric acid, total cholesterol and TAG concentrations were determined using a Roche Modular P800 Autoanalyzer (Roche, Inc.). Malondialdehyde concentration was measured by the thiobarbituric acid-reactive substances (TBARS) method( Reference Griesmacher, Kindhauser and Andert 13 ). ACE activity was analysed as described previously( Reference Vermeirssen, Van Camp and Verstraete 14 ). Advanced glycation end product (AGE) and Ag II concentrations were determined using ELISA kits (STA-317, OxiSelect™; Cell Biolabs, Inc.; EA3501-1; ASSAYPRO). The insulin sensitivity index (ISI) was calculated using the following equation:

Urine samples
Using metabolic cages, 24 h urine samples were collected. The 24 h urine volume was recorded, and urinary protein, creatinine and glucose concentrations were determined using a Roche Modular P800 Autoanalyzer (Roche, Inc.). The creatinine clearance rate (Ccr) was calculated as follows:

Kidney samples
Kidney samples were homogenised with a buffer (50 mm-Tris base, 150 mm-NaCl and 1 % Triton-X 100), and suspensions were centrifuged at 1000 g at 4°C for 10 min. ACE activity and TBARS and Ag II concentrations in the kidneys were determined using the same method as described previously.
Western blot analysis
Kidney and gastrocnemius muscle samples were homogenised in radioimmunoprecipitation assay (RIPA) buffer (50 mm-Tris–HCl, 150 mm-NaCl, 1 % NP-40 and 0·1 % SDS), and suspensions were centrifuged at 14 000 g at 4°C for 10 min. Kidney suspensions were analysed for the expressions of CYP4A (using the polyclonal anti-rat CYP4A antibody, ABR PA3-033), nephrin (using the polyclonal anti-rabbit nephrin antibody, ab58968), tumour growth factor (TGF)-β (using the polyclonal anti-rat TGF-β antibody; Santa Cruz Biotechnology, and using goat anti-rat IgG conjugated with horseradish peroxidase or a goat anti-rabbit IgG peroxidase-conjugated secondary antibody). Gastrocnemius muscle suspensions were analysed for the expression of PPAR-γ (using the polyclonal anti-rabbit PPAR-γ antibody, D69, and a goat anti-rabbit IgG peroxidase-conjugated secondary antibody). The immune complex was developed using an enhanced chemiluminescence detection system (Western Lighting™; PerkinElmer), and bands were quantified by densitometry using Image-Pro Plus 4.5 (Media Cybernetic). Equal loading of the total protein was verified using a commercially available mAb against glyceraldehyde 3-phosphate dehydrogenase, and the results are expressed as the ratio of protein:glyceraldehyde 3-phosphate dehydrogenase.
Pathological analysis
Dissected kidney samples of the rats were fixed in 10 % formaldehyde. The samples were stained with the haematoxylin and eosin stain. Biopsies were examined on a blinded basis by a pathologist, using a 0–3 injury scale, with 0 indicating absent or minimal, 1 indicating mild, 2 indicating moderate and 3 indicating severe injury.
Statistical analysis
Data were analysed using one-way ANOVA and the least significant difference test using the SAS program (version 9.1; SAS, Inc.). Results are expressed as the mean with their standard errors. A P value < 0·05 was taken as the level of statistical significance.
Results
Body weight, food intake and blood pressure
At the end of the study, body weights of the three diabetic groups (D: 176·1 (sem 17·6) g; B: 182·6 (sem 11·2) g; and I: 196·8 (sem 5·6) g) were lower than that of the C group (350·0 (sem 6·5) g) (P =0·000), and food intake of the three diabetic groups (D: 32·3 (sem 1·2) g; B: 30·4 (sem 1·8) g; and I: 31·1 (sem 2·0) g) was also higher than that of the C group (21·3 (sem 2·5) g) (P =0·003). No difference in body weight and food intake was found among the D, B and I groups. The systolic blood pressure of the B group was significantly lower than that of the D group (P =0·014). There was no difference in diastolic blood pressure among all the groups (P =0·406; Table 2).
Table 2 Blood pressure, plasma and kidney biochemical analysis at the end of the study (Mean values with their standard errors)
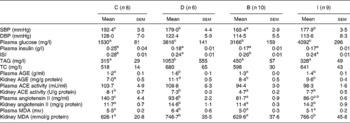
C, control group; D, diabetic nephropathy (DN) group; B, DN+β-conglycinin group; I, DN+soya isoflavone group; SBP, systolic blood pressure; DBP, diastolic blood pressure; ISI, insulin sensitivity index; TC, total cholesterol; AGE, advanced glycation end products; ACE, angiotensin-converting enzyme; MDA, malondialdehyde.
a,b,c,dMean values with unlike superscript letters were significantly different (P <0·05).
Plasma biochemical analysis
At the end of the study, the plasma glucose level of the C group was significantly lower (P =0·000) than those of the DN groups, and the plasma glucose level of the B group was significantly lower (P =0·036) than that of the D group, while no difference was found between the D and I groups (P =0·368) (Table 2). Plasma insulin levels were significantly lower in the three DN groups than in the C group (P =0·004). The ISI of the D group was also lower than that of the C group (P =0·005), while the B group had a significantly higher ISI than the D group (P =0·042) (Table 2). The plasma TAG level of the D group was higher than that of the C group (P =0·025). No difference was found in total cholesterol concentrations among all the groups (Table 2).
Advanced glycation end product levels
Plasma AGE levels of the D and I groups were significantly higher than those of the C and B groups (P =0·005). The kidney AGE level of the D group was significantly higher than that of the C group (P =0·000), and those of both the B and I groups were lower than that of the D group (P =0·003) (Table 2).
Renal function markers in the plasma and urine
Blood urea N levels of the three DN groups were significantly higher than that of the C group (P =0·001). The plasma uric acid concentration of the D group was higher than that of the C group (P =0·000), while no difference was found between the C group and the B and I groups. The 24 h urine volume and urinary glucose excretion of the three DN groups were significantly higher than those of the C group (P =0·000). Urinary protein excretion levels of the D and I groups were significantly higher than that of the C group (P =0·002), while no difference was found between the C and B groups (P =0·914). The Ccr of the DN groups were significantly lower than that of the C group (P =0·018), and there was no difference among the three DN groups (Table 3).
Table 3 Renal function markers at the end of the study (Mean values with their standard errors)
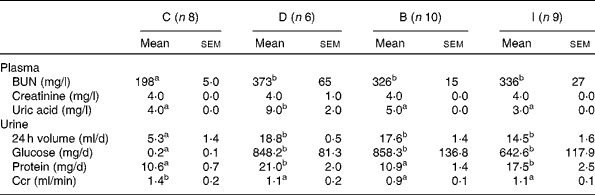
C, control group; D, diabetic nephropathy (DN) group; B, DN+β-conglycinin group; I, DN+soya isoflavone group; BUN, blood urea N; Ccr, creatinine clearance rate.
a,bMean values with unlike superscript letters were significantly different (P <0·05).
Angiotensin-converting enzyme activity and angiotensin II level
At the end of the study, no differences in plasma ACE activity were found among all the groups (P =0·082). However, the kidney ACE activity of the B group was significantly lower than that of the D group (P =0·000), while no difference was found between the D and I groups (P =0·562). Plasma and kidney Ag II concentrations of the B group were significantly lower than those of the D group (P =0·001), and there was no difference between the I and D groups (P =0·738) (Table 2).
Nephrin, cytochrome P450 4A and PPAR-γ protein expressions
Nephrin protein expression of the D group was significantly lower than those of the C and B groups (P =0·004), and no difference was found between the I and D groups (P =0·148) (Fig. 1(A)). CYP4A protein expression of the D group was significantly higher than that of the C group (P =0·024). CYP4A expressions of the B and I groups were also lower than that of the D group (Fig. 1(B)) (P =0·048). Gastrocnemius muscle PPAR-γ protein expression of the D group was significantly lower than those of the C and B groups (P =0·003), but there was no difference between the I and D groups (P =0·119) (Fig. 1(D)).
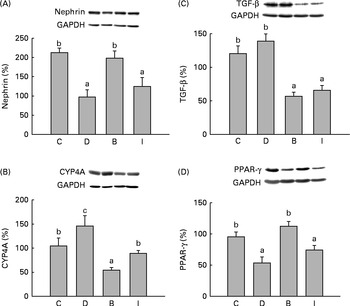
Fig. 1 (A) Nephrin, (B) cytochrome P450 4A (CYP4A) and (C) tumour growth factor (TGF)-β protein expressions in the kidneys and (D) PPAR-γ protein expression in the gastrocnemius muscle at the end of the study. Values are means, with their standard errors represented by vertical bars. a,b,cMean values with unlike letters were significantly different (P <0·05). C, control group (n 8); D, diabetic nephropathy (DN) group (n 6); B, DN+β-conglycinin group (n 10); I, DN+soya isoflavone group (n 9). GAPDH, glyceraldehyde 3-phosphate dehydrogenase.
Thiobarbituric acid-reactive substances and tumour growth factor-β protein expressions
Plasma and kidney TBARS levels of the D group were significantly higher than those of the C group (P =0·036). Plasma TBARS levels of both the B and I groups were lower than that of the D group (P =0·002), while only the B group had a lower kidney TBARS concentration than the D group (Table 2). TGF-β protein expressions of the B and I groups were also lower than that of the D group (P =0·000) (Fig. 1(C)).
Pathological analysis
At the end of the study, we found mild renal vessel congestion in the D group. The score of the B group was significantly lower (P =0·000) than that of the D group, but no significant difference was found between the I and D groups (P =0·050) (Fig. 2). No significant glomerulonephritis or interstitial inflammation was observed in all the groups, and the results indicated that our diabetic rats were only undergoing early-stage nephropathy.
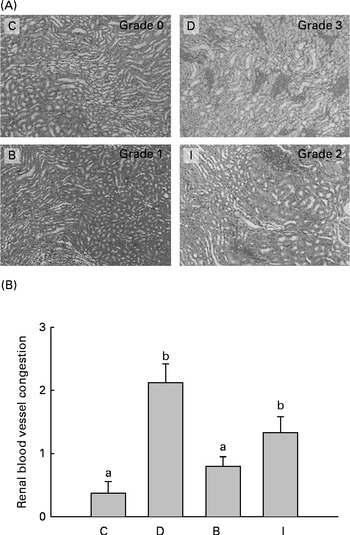
Fig. 2 (A) Histopathology of renal blood vessel congestion (haematoxylin and eosin stain, 40 × ) and (B) score at the end of the study. Values are means, with their standard errors represented by vertical bars. a,bMean values with unlike letters were significantly different (P <0·05). C, control group (n 8); D, diabetic nephropathy (DN) group (n 6); B, DN+β-conglycinin group (n 10); I, DN+soya isoflavone group (n 9). Grade 0, absent or minimal; grade 1, mild; grade 2, moderate; grade 3, severe. (A colour version of this figure can be found online at http://www.journals.cambridge.org/bjn)
Discussion
In the present study, we treated SHR with a single dose of STZ, and the rats showed typical symptoms of diabetes, such as hyperglycaemia, weight loss, polyuria and glycosuria. In addition, the STZ-treated SHR showed higher blood urea N and urinary protein excretion and a lower Ccr, characteristics of renal dysfunction. These results indicate that we have successfully established a rat model of early STZ-induced DN. Although all the diabetic rats exhibited increased food intake, no difference was found among the three groups. The B group orally ingested the diet containing 1·75 % β-conglycinin with 0·01 % soya isoflavones and the D group consumed the same amount of soya isoflavones since both the groups exhibited the same degree of food intake. Therefore, we can confirm that the effects β-conglycinin on diabetic rats are due to its protein composition or soya isoflavones through a comparison of the results of the two groups.
Many studies have reported that soya protein can retard the progression of renal failure( Reference Anderson 15 ). β-Conglycinin is the major storage protein of soyabeans and is considered to be one of the bioactive components in soya protein. In the present study, β-conglycinin was used to replace one-eighth of casein as the dietary protein, and we found that β-conglycinin decreased urinary protein excretion. Nephrin is a protein that helps maintain the filtration barrier integrity in the kidneys( Reference Mundel and Shankland 16 ). A human study has indicated that diabetic patients, before the onset of albuminuria, exhibited increased urinary nephrin excretion( Reference Benigni, Gagliardini and Tomasoni 17 ). Thus, nephrin may be an early indicator of renal injury( Reference Wolf and Ziyadeh 18 ). In animal experiments, renal nephrin expression was also lower in diabetic SHR( Reference Davis, Forbes and Thomas 19 ). We found that β-conglycinin intervention significantly increased nephrin expression and decreased urinary protein excretion in the DN rats. However, we did not find changes in nephrin expression or urinary protein excretion in the I group, which consumed the same amount of isoflavones as the B group. These results suggest that β-conglycinin supplementation retards renal injury in diabetic rats, and this effect cannot be completely explained by its isoflavone content.
A previous study has reported that β-conglycinin improves glucose tolerance by increasing insulin sensitivity( Reference Tachibana, Iwaoka and Hirotsuka 20 ). In the present study, β-conglycinin decreased the fasting blood glucose levels in the DN rats, but it did not affect plasma insulin concentrations. In addition, we also found lower plasma and kidney AGE concentrations in rats consuming the diets containing β-conglycinin. AGE can also interrupt renal nephrin expression( Reference Doublier, Salvidio and Lupia 6 ). Therefore, β-conglycinin might ameliorate hyperglycaemia by increasing insulin sensitivity and thus decrease plasma and kidney AGE levels, which may help to preserve nephrin expression in DN rats. Thiazolidinedione, a PPAR-γ ligand, is a common diabetic medication that can increase insulin sensitivity and ameliorate hyperglycaemia( Reference Willson, Lambert and Kliewer 21 ). However, thiazolidinedione is still restricted in clinical use because of its side effects, such as liver toxicity( Reference Lebovitz 22 ). PPAR-γ is found in many tissues, and PPAR-γ expression in skeletal muscle is important for regulating insulin sensitivity( Reference Lopez-Soriano, Chiellini and Maffei 23 ) and carbohydrate metabolism( Reference Sugita, Kamei and Akaike 24 ). In the present study, gastrocnemius muscle PPAR-γ expression was significantly lower in the DN rats than in the control rats, and the ISI was also lower in the DN rats. A previous study has found that soya protein may stimulate PPAR-γ expression and improve insulin sensitivity( Reference Ronis, Chen and Badeaux 25 ). In the present study, gastrocnemius muscle PPAR-γ expression in the β-conglycinin diet-fed rats was significantly higher than that in the DN group and the ISI was also higher than that of the DN group. The present results suggest that β-conglycinin supplementation may increase insulin sensitivity via the stimulation of gastrocnemius muscle PPAR-γ expression. Although some studies have also reported that soya isoflavones stimulate insulin secretion in vivo and in vitro ( Reference Hamden, Jaouadi and Carreau 26 ), we did not find significant effects of soya isoflavones on insulin or blood sugar levels.
In the present study, rats with STZ-induced DN exhibited lower blood pressure than the control rats, which was consistent with the finding in a previous study that a STZ injection may interfere with the development of hypertension in SHR( Reference Sato, Nara and Kato 27 ). Studies have suggested that the renin–angiotensin system (RAS) in local tissues is more important for long-term blood pressure maintenance( Reference Gibbons 28 ) and cardiorenal remodelling( Reference Mackenzie and Brenner 29 ). Glomerular hypertension leads to increased permeability in Bowman's capsule and urinary albumin excretion, leading to the progression of DN( Reference Wolf and Risler 30 ). In clinical studies, ACE inhibitors can reduce blood pressure and urinary protein excretion and retard the progression of DN( Reference Grundy, Benjamin and Burke 31 , Reference Cravedi, Ruggenenti and Remuzzi 32 ). In addition, high concentrations of Ag II in vivo may also inhibit renal nephrin expression( Reference Jia, Ding and Zhu 33 ). We found that the β-conglycinin diet-fed rats exhibited lower systolic blood pressure, kidney ACE activity and Ag II concentrations. These results suggest that the ACE-inhibitory effect of β-conglycinin in the kidneys leads to a decrease in the production of Ag II and consequently plays a role in decreasing blood pressure and increasing nephrin expression. CYP4A expression in the rat kidney converts arachidonic acid to hydroxyeicosatetraenoic acid. Hydroxyeicosatetraenoic acid is a vasoactive substance that stimulates Ag II release from renal vessels and leads to an elevation in blood pressure( Reference Zhang, Qian and Wang 34 ). In the present study, both β-conglycinin and soya isoflavones reduced kidney CYP4A expression, and thus, the effect of β-conglycinin on blood pressure may partially have been caused by the inhibition of CYP4A expression and the inhibitory effect of β-conglycinin on CYP4A may partially have been caused by its isoflavone content.
Hyperglycaemia increases oxidative stress and reactive oxygen species generation, inflammatory factor release( Reference Calcutt, Cooper and Kern 35 ) and RAS activation( Reference Ruggenenti, Cravedi and Remuzzi 36 ) and promotes AGE generation( Reference Decleves and Sharma 37 ). High blood glucose, Ag II and AGE concentrations also stimulate TGF-β expression( Reference Ziyadeh and Wolf 3 ). TGF-β overexpression may lead to inflammation, basement membrane extracellular matrix accumulation and renal injury( Reference Dronavalli, Duka and Bakris 4 ). In the present study, β-conglycinin intervention decreased TBARS concentration and TGF-β expression and soya isoflavones also reduced oxidative and inflammatory indicators in the DN rats. Previous studies have indicated that soya protein exhibits renal protective effects in animal models subjected to various renal diseases, which may be related to the effects of its isoflavone content, such as anti-inflammatory and antioxidative effects( Reference Anderson 15 ). For example, genistein down-regulated TGF-β gene expression at the transcriptional level( Reference Kim, Peterson and Barnes 38 ). Therefore, in addition to improving hyperglycaemia and inactivating the RAS, the soya isoflavones of β-conglycinin may also normalise oxidative stress and decrease TGF-β expression in DN rats.
Hyperlipidaemia also plays an important role in the progression of renal diseases( Reference Kasiske, Lakatua and Ma 39 ). Hyperlipidaemia may increase glomerular vascular permeability and lead to renal dysfunction. Improvement of plasma lipid profiles can retard renal failure( Reference Kallerhoff, Gotz and Grone 40 ). In the present study, the DN rats exhibited hypertriacylglycerolaemia. β-Conglycinin was reported to reduce TAG levels by decreasing sterol regulatory element-binding protein 1 (SREBP1) mRNA expression( Reference Moriyama, Kishimoto and Nagai 12 ) or regulating adiponectin levels( Reference Tachibana, Iwaoka and Hirotsuka 20 ), and these two mechanisms are affected by insulin. Therefore, β-conglycinin can reduce plasma TAG concentrations in DN rats and may improve insulin sensitivity. Soya isoflavones may act as ligands of oestrogen receptors( Reference Bairey Merz, Johnson and Braunstein 41 ) or regulate lipid metabolism via protein signalling( Reference Ronis, Chen and Badeaux 25 ). In the present study, we also found that soya isoflavones lowered plasma TAG concentrations in the DN rats. The results suggest that β-conglycinin may also reduce plasma levels because of its isoflavone content.
In summary, β-conglycinin increased PPAR-γ expression and improved insulin sensitivity and thus ameliorated hyperglycaemia and AGE generation. In addition, β-conglycinin inhibited the activity of ACE, decreased the generation of Ag II and retarded the elevation of blood pressure and oxidative stress. It also improved renal nephrin expression and may be beneficial for maintaining the renal filtration barrier. Although soya isoflavones can protect against oxidative stress and hyperlipidaemia in DN, they alone were unable to retard the progression of renal dysfunction in the present study. Therefore, the beneficial effects of β-conglycinin cannot be completely explained by its isoflavone content.
Acknowledgements
The present study was funded by the National Science Council (NSC 101-2320-B-038-016, Taipei, Taiwan). The contribution of each author was as follows: H.-Y. Y., L.-Y. W. and W.-J. Y. performed the experiments and contributed to the writing of the manuscript; J.-R. C. designed the study and was the promoter, investigator and coordinator. All authors read and approved the final contents of the manuscript. The authors declare that there are no conflicts of interest and that they have adhered to the Committee on Publication Ethics guidelines on research and publication practice.