Introduction
The cosmopolitan family Strigeidae Railliet, 1919 currently contains 13 genera with approximately 110 nominal species distributed worldwide (Niewiadomska, Reference Niewiadomska, Gibson, Jones and Bray2002). The type genus Strigea was established by Abildgaard, 1790 to accommodate species that have vitellarium uniformly distributed over both parts of the body and the presence of a pharynx (Dubois, Reference Dubois1968; Niewiadomska, Reference Niewiadomska, Gibson, Jones and Bray2002). Among strigeids, Strigea is considered the most diverse genus within the family, with approximately 47 nominal species associated mainly with strigiform, accipitriform, falconiform, ciconiiform, caprimulgiform, cariamiform, passeriform, gruiform, trogoniform and anseriform birds (Dubois, Reference Dubois1968; Drago et al., Reference Drago, Lunaschi and Draghi2014). Information on the life cycle of most species of Strigea is scarce, but it is thought to involve four hosts. Adult worms live and reproduce sexually in the digestive tracts of birds that serve as definitive hosts. Eggs are expelled into the environment with the faeces of the host. After the ingestion of the eggs by a planorbid snail, which serves as the first intermediate host, the parasites develop into cercariae. The cercariae emerge and swim to find and penetrate the second intermediate host (amphibians), where they develop into mesocercariae and in some cases it may cause severe morphological anomalies as the polydactyly (Sinsch et al., Reference Sinsch, Heneberg, Těšínský, Balczun and Scheid2019; Svinin et al., Reference Svinin, Bashinskiy and Litvinchuk2020, Reference Svinin, Bashinskiy, Ermakov and Litvinchuk2023). The amphibian with the mesocercaria is ingested by the third intermediate host (an amphibian, reptile, bird, or small mammal) and then the parasite develops into an encysted, tetracotyle-type metacercaria. Finally, these amphibians, reptiles, birds and mammals are some of the principal food resources of prey birds, in which the life cycle is completed (Pearson, Reference Pearson1959, Reference Pearson and Dawes1972; Odening, Reference Odening1967).
To date, Strigea contains 47 described species, nine of which are distributed in Asia, nine in Africa, five in Oceania and three in Europe (Dubois, Reference Dubois1968, Reference Dubois1988; Dubois & Beverley-Burton, Reference Dubois and Beverley-Burton1971). In the Americas, 21 species have been described, of which six are in North America (Strigea infundibuliformis Dubois, 1934; Strigea macroconophora Dubois and Rausch, 1950; Strigea elegans Chandler and Rausch, Reference Chandler and Rausch1947; Strigea sphaerula macrosicya Dubois and Rausch, 1950; Strigea gruis Dubois and Rausch, 1964 and Strigea macropharynx Dubois and Rausch, 1965); and 15 species in South America (Strigea caryophylla (Diesing, 1850) Mathias, 1925; Strigea elliptica (Brandes, 1888) Szidat, 1928; Strigea bulbosa (Brandes, 1888) Szidat, 1928; Strigea nugax Szidat, 1928; Strigea vaginata (Brandes, 1888) Szidat, 1928; Strigea falconis brasiliana Szidat, 1929; Strigea caluri Dubois, 1962; Strigea sphaerocephala (Westrumb, 1823) Dubois, 1937; Strigea microbursa Pearson and Dubois, Reference Pearson and Dubois1985; Strigea magniova Dubois, Reference Dubois1988; Strigea arcuata Dubois, Reference Dubois1988; Strigea meridionalis Lunaschi and Drago, Reference Lunaschi and Drago2009; Strigea inflecta Lunaschi and Drago, Reference Lunaschi and Drago2012; Strigea orbiculata Lunaschi and Drago, Reference Lunaschi and Drago2013 and Strigea proteolytica Drago, Lunaschi and Draghi, Reference Drago, Lunaschi and Draghi2014) (Dubois, Reference Dubois1968; Lunaschi & Drago, Reference Lunaschi and Drago2006, Reference Lunaschi and Drago2009, Reference Lunaschi and Drago2012, Reference Lunaschi and Drago2013; Drago et al., Reference Drago, Lunaschi and Draghi2014). The morphological identification of Strigea spp. is complex and problematic due to their small size and the difficulty in observing internal and external structures used for taxonomy and differentiation among species (Lunaschi & Drago, Reference Lunaschi and Drago2006, Reference Lunaschi and Drago2009, Reference Lunaschi and Drago2012, Reference Lunaschi and Drago2013; Drago et al., Reference Drago, Lunaschi and Draghi2014). Additionally, molecular data are scarce and only a few sequences of Strigea are currently available (Hernández-Mena et al., Reference Hernández-Mena, García-Varela and Pérez-Ponce de León2017; Heneberg et al., Reference Heneberg, Sitko, Těšínský, Rzad and Bizos2018; Svinin et al., Reference Svinin, Bashinskiy and Litvinchuk2020). In Mexico, the metacercaria of Strigea was recorded for the first time by Vidal-Martínez (Reference Vidal-Martínez1995) in two cichlid fish species in south-eastern Mexico (Pérez-Ponce de León et al., Reference Pérez-Ponce de León, García-Prieto and Mendoza-Gárfias2007). However, the specimens were not deposited, and therefore, the records could not be verified. Hernández-Mena et al. (Reference Hernández-Mena, García-Varela and Pérez-Ponce de León2017) recorded an adult of Strigea sp. in crested caracara (Caracara cheriway Jacquin) in Presa La Angostura, Chiapas, Mexico. Recently, strigeids in Mexico have started to receive attention and much effort has been made to incorporate morphological and molecular characteristics to describe and delineate the biodiversity of this group of parasites (Hernández-Mena et al., Reference Hernández-Mena, García-Prieto and García-Varela2014, Reference Hernández-Mena, García-Varela and Pérez-Ponce de León2017; López-Jiménez et al., Reference López-Jiménez, Hernández-Mena, Solórzano-García and García-Varela2021, Reference López-Jiménez, González-García and García-Varela2022).
In the current study, adult specimens of the genus Strigea were collected from the intestine of roadside hawk (Rupornis magnirostris Gmelin) and Cooper's hawk (Accipiter cooperii Bonaparte) in six localities from the Neotropical region of Mexico. After a careful morphological examination, the specimens were determined to correspond to an undescribed species of the genus Strigea. In addition, other strigeids collected from the intestine of the great black hawk (Buteogallus urubitinga Gmelin) and common black hawk (Buteogallus anthracinus Deepe) were identified as Parastrigea macrobursa Drago & Lunaschi, Reference Drago and Lunaschi2011, a species previously described in South America.
The objectives of the present research were: (a) to provide a morphological description of the new species; and (b) to test the systematic position of P. macrobursa by using sequences of the internal transcribed spacers (ITS1-5.8S rDNA- ITS2) and large subunit (LSU) of the nuclear DNA and of the cytochrome c oxidase subunit I (cox 1) gene of the mitochondrial DNA. We then used the resulting phylogenetic trees as a framework to discuss host–parasite associations and begin to understand the evolutionary history of this group of strigeids.
Materials and methods
Specimen collection
A total of 17 hawks were collected between December 2019 and December 2021 in nine localities from Mexico (fig. 1; table 1). Ten individuals of roadside hawk (R. magnirostris), one Cooper's hawk (A. cooperii), two individuals of great black hawk (Buteogallus urubitinga) and four common black hawks (B. anthracinus). Birds were identified following Howell & Webb (Reference Howell and Webb1995) and the American Ornithologist’ Union (1998) guidelines. Strigeids were removed from the intestines of the birds and examined using a stereomicroscope. Digeneans collected were relaxed in hot distilled water and preserved in 100% ethanol for morphological and molecular analyses.

Fig. 1. Map of Mexico showing the sampled sites for the birds. Localities with a circle with colours green and red were positive for the infection with Strigea magnirostris n. sp. and Strigea macrobursa n. comb., respectively. Localities correspond to those in table 1.
Table 1. Specimens’ information for Strigea spp., locality, state, geographical coordinates, host name, number of host examined/infected (prevalence of infection) and GenBank accession number for specimens studied in the current study.

The sample number for each locality corresponds with the same number in Figure 1.
Morphological analyses
Digeneans preserved in 100% ethanol were stained with Mayer's paracarmine (Merck, Darmstadt, Germany), dehydrated in ethanol series, cleared in methyl salicylate and mounted in Canada balsam for morphological analysis. Specimens were examined using a compound microscope equipped with a bright field Leica DM 1000 light emitting diode microscope (Leica, Wetzlar, Germany). Measurements were taken using Leica Application Suite microscope software (Leica Microsystems GmbH, Wetzlar, Germany) and are given in micrometres and presented with the range followed by the mean in parentheses. Some specimens were dehydrated with an ethanol series, critical point dried, sputter coated with gold and examined with a Hitachi Stereoscan Model S-2469N scanning electron microscope operating at 15 kV. Voucher specimens from the present study were deposited in the Colección Nacional de Helmintos (CNHE) from Instituto de Biología, Universidad Nacional Autónoma de México (UNAM), Mexico City.
DNA isolation, amplification and sequencing
Strigeids preserved in 100% ethanol were placed individually in tubes and digested overnight at 56°C in a solution containing 20 mm sodium chloride, 10 mm Tris–hydrochloride (pH = 7.6), 100 mm ethylenedinitrilotetraacetic acid disodium salt dihydrate (pH = 8.0), 1% Sarkosyl and 0.1 mg/ml proteinase K. Following digestion, DNA was extracted from the supernatant using the DNAzol reagent (Molecular Research Center, Cincinnati, Ohio). The internal transcribed spacers (ITS1-5.8S rDNA- ITS2) of the nuclear ribosomal DNA were amplified using the forward primer BD1 5′-GTCGTAACAAGGTTTCCGTA- 3′ (Bowles & McManus, Reference Bowles and McManus1993) and the reverse primer BD2 5′-ATCTAGACCGGACTAGGCTGTG-3′ (Bowles et al., Reference Bowles, Blair and McManus1995). The partial fragments of domains D1–D3 of the large subunit of nuclear ribosomal RNA (LSU) were amplified with the forward primer 391 5′-AGCGGAGGAAAAGAAACTAA-3′ (Nadler et al., Reference Nadler, Hoberg, Hudspeth and Rickard2000) and the reverse primer 536, 5′ -CAGCTATCCTGAGGGAAAC-3′ (García-Varela & Nadler, Reference García-Varela and Nadler2005). The complete gene of the cytochrome c oxidase subunit 1 (cox 1; 850 base pairs (bp)) was amplified using the forward primers AphaF, 5′-TATGATTTTTTTYTTTTTRATG-3′ and the reverse primer JB4.5′-TAAAGAACATAATGAAATTG3′ (Bowles et al., Reference Bowles, Blair and McManus1992). Polymerase chain reactions (PCRs) were carried out in 25 μl reactions, consisted of 1 μl of each primer, 2.5 μl of 10 × buffer, 1.5 μl MgCl2, 0.5 μl of dNTP mixture, 0.125 μl of Platinum Taq DNA polymerase (Invitrogen Corporation, São Paulo, Brazil) and 2 μl of genomic DNA. PCR cycling parameters for amplifications consisted of denaturation at 94°C for 1 min, 35 cycles of 94°C for 1 min, 50°C for 1 min and 72°C for 1 min, followed by a post-amplification incubation at 72°C for 10 min. Sequencing reactions were performed using ABI Big Dye (Applied Biosystems, Boston, Massachusetts) terminator sequencing chemistry and reaction products were separated and detected using an ABI 3730 capillary DNA sequencer. Contigs were assembled and base-calling differences resolved using Codoncode Aligner version 9.0.1 (Codoncode Corporation, Dedham, Massachusetts) and submitted to the GenBank dataset (table 1).
Alignments and phylogenetic analysis
Newly-generated sequences of ITS, LSU and cox 1 were aligned with other sequences of strigeids available in the GenBank dataset. Sequences of each molecular marker were aligned using the software CLUSTAL_X (Thompson et al., Reference Thompson, Gibson, Plewnlak, Jeanmougin and Higgins1997). The best nucleotide substitution model was selected for each molecular marker using jModelTest v2.1.7 (Posada, Reference Posada2008) and applying the Akaike information criterion. The best nucleotide substitution model for the ITS and LSU dataset were TVM + I + G and for cox 1 the dataset was TIM3 + I + G. Phylogenetic trees were reconstructed through maximum likelihood (ML) with the program RAxML v7.0.4 (Silvestro & Michalak, Reference Silvestro and Michalak2012), and Bayesian inference (BI) analyses were inferred with MrBayes 3.2.2 (Ronquist et al., Reference Ronquist, Teslenko and Van Der Mark2012) using the computational resource Cyberinfrastructure for Phylogenetic Research Science Gateway v3.3 (Miller et al., Reference Miller, Pfeiffer and Schwartz2010). ML analyses were inferred with the option GTRGAMMAI and 10,000 bootstrap replicates. BI analyses included Markov chain Monte Carlo searches of two simultaneous runs for 10 million generations, sampling every 1000 generations, a heating parameter value of 0.2 and a burn-in of 25%. Trees were drawn and edited using FigTree software v1.4.0 (Rambaut, Reference Rambaut2012). Genetic divergences were estimated using P uncorrected distances with MEGA v.6 (Tamura et al., Reference Tamura, Stecher, Peterson, Filipski and Kumar2013).
Results
Molecular characterization and phylogenetic analyses
Nuclear genes
The ITS dataset included 42 sequences with 1042 characters. The phylogenetic analyses performed with ML and BI showed that the genus Strigea is monophyletic and is subdivided into two major subclades (fig. 2). The first subclade was formed by 17 isolates of an undescribed species of Strigea sp. from the roadside hawk (R. magnirostris) and Cooper's hawk (A. cooperii) collected from six localities in Mexico. This clade is sister to another subclade formed by eight isolates identified morphologically as P. macrobursa recovered from the great black hawk (Buteogallus urubitinga) and common black hawk (B. anthracinus) from three localities in Mexico. All these relationships were supported with well-supported bootstrap values and Bayesian posterior probabilities (fig. 2). The intraspecific genetic divergence among 17 isolates of Strigea sp. was low, ranging from 0 to 0.3%, whereas that for P. macrobursa ranged from 0 to 0.2% for ITS. The LSU dataset consisted of 21 terminals and 1208 characters. The tree topologies inferred using the LSU dataset from the nuclear DNA showed that the genus Strigea is paraphyletic because the genus was subdivided into two major clades. The first major clade was formed by two sequences identified as Strigea robusta (MT075841 and MK585230) recovered from the marsh frog (Pelophylax ridibundus) and edible frog (Pelophylax esculentus), respectively, from Russia, and this clade was sister to a clade formed by species of the genera Parastrigea Szidat, 1928 and Apharyngostrigea Ciurea, 1927 (fig. 3). The second major clade was formed by four subclades. The first subclade contains an unidentified sequence of Strigea sp. (KT362372) from water frog (Pelophylax sp.) from France. The second subclade was formed by nine isolates of P. macrobursa from Mexico, which is a sister to the third subclade formed by a single sequence of an unidentified sample of Strigea sp. (MF398343) recovered from crested caracara (Caracara cheriway) from Presa La Angostura, Chiapas, Mexico. The fourth subclade was formed by 14 isolates of Strigea sp. recovered from the roadside hawk (R. magnirostris) and Cooper's hawk (A. cooperii) from six localities in Mexico (fig. 3). Finally, the intraspecific genetic divergence among 14 isolates of Strigea sp. was low, ranging from 0 to 0.10%, whereas that for nine isolates of P. macrobursa ranged from 0 to 0.08% for LSU.

Fig. 2. Phylogenetic trees inferred with Maximum Likelihood (ML) and consensus Bayesian Inference (BI) with the internal transcribed spacers dataset. Numbers near internal nodes show maximum likelihood bootstrap percentage values and Bayesian posterior probabilities.

Fig. 3. Phylogenetics trees inferred with Maximum Likelihood (ML) and consensus Bayesian Inference (BI) with the large subunit dataset. Numbers near internal nodes show ML bootstrap percentage values and Bayesian posterior probabilities.
Mitochondrial gene
The newly completed sequences from cox 1 were aligned with other partial sequences downloaded from GenBank. The alignment included the first region of cox 1 with 42 sequences and 374 characters. The phylogenetic analyses performed with ML and BI showed that the genus Strigea is monophyletic (fig. 4). The clade was subdivided into three subclades. The first subclade was formed by 16 isolates of an undescribed species of Strigea sp. from the Neotropical region of Mexico. The second subclade was formed by an isolate of an unidentified sequence of Strigea sp. (MF398319) from Presa La Angostura, Chiapas, Mexico. The third subclade was formed by seven isolates identified morphologically as P. macrobursa. All these relationships had high bootstrap values and Bayesian posterior probabilities (fig. 4). The intraspecific genetic divergence ranged from 0 to 1% among isolates of Strigea sp. and from 0 to 1.3% for P. macrobursa for cox 1.
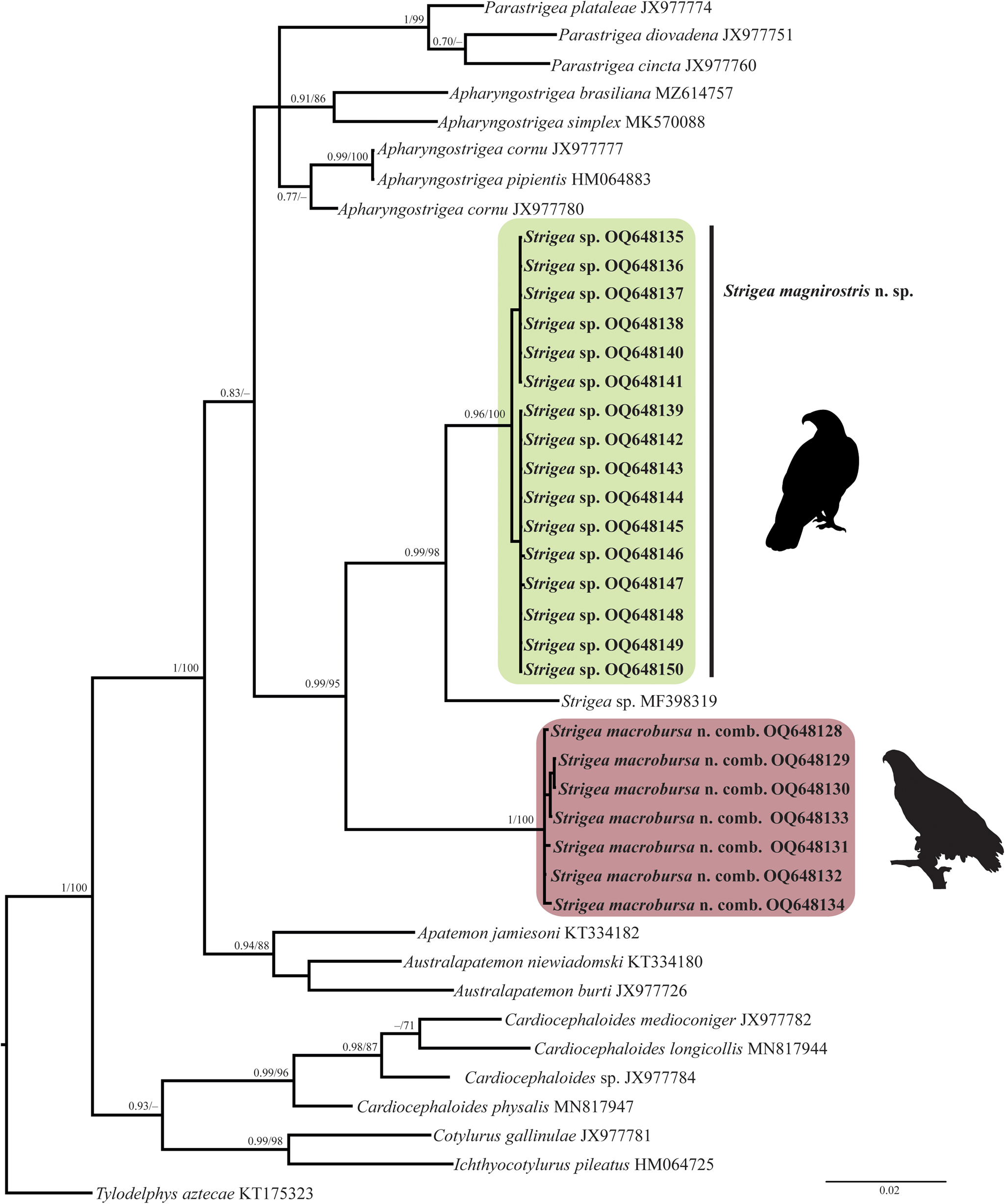
Fig. 4. Phylogenetics trees inferred with Maximum Likelihood (ML) and consensus Bayesian Inference (BI) with the cox 1 dataset. Numbers near internal nodes show ML bootstrap percentage values and Bayesian posterior probabilities.
In summary, the phylogenetic analyses performed with two nuclear markers and one mitochondrial molecular marker supported the monophyly of all new isolates of Strigea spp. from the Neotropical region (figs 2–4). The new ITS, LSU and cox 1 sequences revealed that our specimens of Strigea sp. recovered from the roadside hawk (R. magnirostris) and Cooper's hawk (A. cooperii) from six localities in the Neotropical region of Mexico formed an independent lineage, which is recognized herein as a new species and is described next as Strigea magnirostris n. sp., representing the first species to Mexico and the 22nd to the Americas. In addition, the specimens identified as P. macrobursa collected from the intestine of the great black hawk (B. urubitinga) (type host) and common black hawk (B. anthracinus) formed a clade nested inside Strigea, and as a result, it should be transferred to Strigea to form Strigea macrobursa n. comb. (figs 2–4).
Morphological description
Family Strigeidae Railliet, 1919
Subfamily Strigeinae Railliet, 1919
Genus Strigea Abildgaard, 1790
Strigea magnirostris n. sp.
Type host: R. magnirostris (roadside hawk) (Accipitriformes: Accipitridae).
Other host: A. cooperii (Cooper's hawk) (Accipitriformes: Accipitridae).
Type locality: Tamiahua, Veracruz, Mexico (21°18′02″ N, 97°26′56.2″ W).
Other locality: Tres Vidas, Guerrero, Mexico (16°43′59.8″ N, 99°42′48.9″ W).
Site in host: Intestine.
Prevalence: eight of 11 (72%).
Type material: Holotype CNHE 11118; paratypes CNHE 11119; voucher CNHE 11120.
GenBank accession number: ITS, OQ647940–56; LSU, OQ647909–22; cox 1, OQ648135–50.
Etymology: The epithet of the species refers to the specific name of the type host.
Description (figs 5 and 6; table 2)

Fig. 5. Adult of Strigea magnirostris n. sp. from Rupornis magnirostris; whole worm, holotype, lateral view. Scale bars = 500 μm.

Fig. 6. Scanning electron micrographs of Strigea magnirostris n. sp. from Rupornis magnirostris. (a) Whole worm, ventral view; (b) forebody, ventral view showing pseudo-suckers; (c) oral sucker with papillae; (d) tegumental spines, ventral view of the forebody; (e) copulatory bursa showing cone genital. Scale bars: (a) 400 μm; (b, e) 100 μm; (c) 50 μm; (d) 10 μm.
Table 2. Comparative measurements of Strigea magnirostris n. sp. and related species.

Gc/eggs, genital cone length/egg length; Hi/Fo, hind-body length/forebody length; Hi/Gc, hind-body length/genital cone length; Ph/Os, pharynx width/oral sucker width; Vs/Os, suckers width ratio.
a Calculated from original descriptions.
b Calculated from original descriptions by Drago et al. (Reference Drago, Lunaschi and Draghi2014).
Description (based on 17 adult specimens) (figs 5 and 6): Body 3.03–4.43 mm (3.93 mm) in total length. Tegument spines on the surface of the forebody (fig. 6d). Forebody is longer than is wide, covered with tiny rounded spines, 562–872 (749) long by 400–690 (583) wide, representing 20% of body length (BL) (figs 5 and 6b–d). Hind-body long, strongly curved dorsally with tegument smooth, 2440–3591 (3176) long by 352–632 (492) wide, almost four times longer than the forebody, with a ratio of hind-body length to forebody length of 1: 3.3–5.3 (4.2). Oral sucker terminal, well developed, 77–109 (97) long by 80–115 (100) wide, with several papillae around it (fig. 6c). Ventral sucker well developed, larger than oral sucker, 150–240 (187) long by 124–188 (158) wide. Ratio of ventral sucker length to oral sucker length is 1: 1.45–2.42 (2.0). Pharynx 65–103 (80) long by 64–90 (72) wide. Ratio of pharynx length to oral sucker length is 0.84–1.10 (0.97). Pseudosuckers well developed with conspicuous folds in anterior section, 114–248 (185) long by 77–12 (101) wide (fig. 6b). Holdfast organ lobes can be projected beyond the anterior margin of the forebody, proteolytic gland at base of forebody, 182–225 (200) long by 83–119 (106) wide. Testes in tandem, bilobed, situated near posterior end of the body, anterior testis 272–476 (370) long by 261–496 (378) wide, posterior testis slightly larger than anterior testis at 346–497(420) long by 280–512 (400) wide. Seminal vesicle long, sinuous, postesticular, slightly overlapping with posterior testis. Ovary reniform, pretesticular 139–190 (165) long by 126–214 (170) wide. Mehlis’ gland and vitelline reservoir in the intertesticular region. Vitelline follicles of different sizes in both body segments; in the forebody, small follicles extend into the holdfast organ and lateral body wall from the posterior margin of the sucker ventral, while in the hind-body, large follicles are mostly concentrated in the neck (pre-ovarian region) ventrally to the seminal vesicle or copulatory bursa (fig. 5). Copulatory bursa large triangle-shaped broadening in posterior end, 247–531 (390) long by 468–784 (630) wide (figs 5 and 6e). Muscular ring (Ringnapf) well developed. Genital cone large and well delimited from body parenchyma, 193–361 (280) long by 380–637 (512) wide, ejaculatory duct and uterus join at base of genital cone, forming hermaphroditic duct. Uterus with large and numerous eggs (20–50) (35), oval, 71–105 long by 40–65 (52) wide. Ratio of genital cone length to egg length is 1: 1.95–3.88 (2.9). Excretory pore terminal.
Remarks
Currently, 21 species of the genus Strigea have been described in the Americas that parasitize strigiform, ciconiiform, falconiform, caprimulgiform, passeriform, gruiform, trogoniform and anseriform birds. Of the 21 described species, only five species (S. falconis brasiliana, S. elegans, S. microbursa, S. magniova and S. arcuata) share morphological characteristics with S. magnirostris n. sp., such as body shape, presence of a neck region in the hind-body and distribution of vitelline follicles in the forebody, which are scarce and extend into the lobes from the holdfast organ (Chandler & Rausch, Reference Chandler and Rausch1947; Dubois, Reference Dubois1968, Reference Dubois1988; Pearson & Dubois, Reference Pearson and Dubois1985; Lunaschi & Drago, Reference Lunaschi and Drago2006, Reference Lunaschi and Drago2009). The new species most closely resembles S. arcuata, S. microbursa and S. elegans by having pseudosuckers that are well developed in the forebody. However, S. arcuata can be distinguished from S. magnirostris n. sp. by having a smaller genital cone included in a circular muscular formation (125 × 145 vs. 193–361 × 296–637 in S. magnirostris). In addition, S. arcuata possesses lower limits for the following characteristics: pseudosuckers (105 × 80 vs. 118–248 × 64–125 in S. magnirostris); hind-body width (180 vs. 352–632); anterior testes (255 × 185 vs. 272–476 × 261–496); and posterior testes (340 × 260 vs. 300–497 × 280–512). The species S. microbursa can be distinguished from S. magnirostris n. sp. by having a smaller genital cone (100–180 × 80–140 vs. 193–361 × 296–637). In addition, S. microbursa possesses lower limits for the following characteristics: pseudosuckers (85–95 × 90–95 vs. 118–248 × 64–125 in S. magnirostris); forebody width (230–300 vs. 400–690); ovary length (55–140 vs. 139–190); and ovary width (80–106 vs. 126–214). Finally, S. elegans can be distinguished from S. magnirostris n. sp. due to its smaller BL (1550–2450 vs. 3030–4437), smaller copulatory bursa (350 diam. vs. 247–531 × 468–784) and larger eggs (115–220 vs. 71–105) (see table 2).
Morphological redescription
Strigea macrobursa n. comb.
Syn. Parastrigea macrobursa Drago and Lunaschi, Reference Drago and Lunaschi2011
Host: B. urubitinga (great black hawk) (Accipitriformes: Accipitridae).
Other host: B. anthracinus (common black hawk) (Accipitriformes: Accipitridae)
Locality: Isla Aguada, Campeche, Mexico (18°48′22.92″ N, 91°28′03.68″ W).
Other localities: Tecolutla, Veracruz, Mexico (20°33′49.8″ N, 97°05′57.7″ W).
Site in host: Intestine.
Prevalence: 3 of 6 (50%).
Voucher specimens: CNHE 11121, 11122.
GenBank accession number: ITS OQ647932–39; LSU OQ647923–31; cox 1 OQ648128–34.
Description (figs 7 and 8; table 3)

Fig. 7. Adult of Strigea macrobursa n. comb. from Buteogallus urubitinga. (a) Whole worm; (b) forebody, ventral view; (c) whole worm. Scale bars: (a) 300 μm; (b) 100 μm; (c) 250 μm.

Fig. 8. Scanning electron micrographs of Strigea macrobursa n. comb. from Buteogallus urubitinga. (a) Whole worm, ventral view; (b) forebody, ventral view; (c) oral sucker; (d) tegumental spines; (e) copulatory bursa. Scale bars: (a) 500 μm; (b, e) 400 μm; (c) 200 μm; (d) 10 μm.
Table 3. Comparative measurements of adults Strigea macrobursa Drago & Lunaschi, Reference Drago and Lunaschi2011 recorded in the Americas.

BL/Fo, body length/forebody length; BL/E, body length/eggs length; Hi/Fo, hind-body length/forebody length.
Description (based on 26 adult specimens) (figs 7 and 8): Body 957–2.88 mm (1920 mm) in total length. Forebody tulip-shaped, 344–775 (560) long by 238–562 (400) wide (fig. 7b). Tegument spines on the surface of the forebody (fig. 8d). Hind-body slightly plump with tegument smooth, two to three times longer than the forebody at 609–2184 (1400) long by 256–759 (508) wide, with some specimens having a neck region (nine individuals) and some specimens lacking a neck region (17 individuals) (fig. 7a, c). Ratio of BL to forebody length is 1: 2.5–4.1 (1: 3.3). Ratio of hind-body length to forebody length is 1: 1.5–3.1 (1: 2.3). Oral sucker subterminal, well developed, 64–95 (80) long by 57–86 (71) wide (fig. 8c). Ventral sucker oval, 74–106 (90) long by 55–98 (76) wide. Prepharynx absent, pharynx 33–66 (52) long by 31–59 (47) wide. Holdfast organ lobes reaching anterior end (fig. 8b, c), proteolytic gland at base of forebody 75 long by 43 wide. Testes in tandem, large, not lobed, anterior testis oval 95–281 (190) long by 132–449 (290) wide, posterior testis slightly larger than anterior testis at 137–392 (260) long by 155–474 (314) wide. Seminal vesicle long, postesticular. Ovary oval, pre-testicular or slightly overlapping anterior testis, 52–188 (120) long by 72–193 (130) wide. Laure's canal, opening dorsally between ovary and anterior testis. Mehlis’ gland and vitelline reservoir in the intertesticular region. Vitelline follicles similar in size in both body segments; in the forebody, they are in the dorsal lip of the holdfast organ forming two symmetrical masses situated between the ventral sucker and intersegmental constriction; in the hind-body, the vitelline follicles are concentrated in the pre-ovarian region, extending ventrally to the posterior testis or copulatory bursa (fig. 7a–c). Copulatory bursa large, delimited by pronounced constriction, occupying 30%–45% (40%) of hind-body length, 163–681 (422) long by 246–616 (430) wide (fig. 8e). Muscular ring (Ringnapf) absent. Genital cone well delimited from body parenchyma, 89–255 (170) long by 137–230 (180), ejaculatory duct and uterus join at base of genital cone forming hermaphroditic duct. Uterus with large and numerous eggs 3–50 (26) that are 72–117 (95) long by 45–67 (56) wide. Ratio of BL to egg length is 1: 10–28 (1: 19). Genital atrium very deep, genital pore terminal. Excretory pore dorso-subterminal at the level of the copulatory bursa (see table 3).
Remarks
This species was originally described as P. macrobursa by Drago & Lunaschi (Reference Drago and Lunaschi2011) from the great black hawk (B. urubitinga) from Argentina. The specimens collected in the present study are similar to those of the original description by Drago & Lunaschi (Reference Drago and Lunaschi2011). For instance, a forebody tulip-shaped and vitelline follicles distributed in two lateral expansions and a large well-delimited copulatory bursa, with a well-delimited genital cone and deep genital atrium. However, the newly collected specimens from the great black hawk (type host) and common black hawk in three localities from the Neotropical region of Mexico showed some level of morphological intraspecific variation. For example, some of our specimens exhibit a neck in the hind-body, whereas other specimens do not. In addition, our specimens have tegumental spines that gradually diminish in size and number from the anterior to posterior region. However, apparently the presence or absence of spines could be related to the development of the worms. A similar pattern has been observed in specimens of two species, S. falconis brasiliana and S. elliptica, from the Neotropical region (Lunaschi & Drago, Reference Lunaschi and Drago2006, Reference Lunaschi and Drago2009). Finally, our specimens possess higher limits than original description for the following characteristics: hind-body length (609–2184 vs. 754–1451); ovary length (52–188 vs. 69–131); anterior testes width (132–449 vs. 188–262); and posterior testes width (155–474 vs. 193–304) (see table 3).
Discussion
The taxonomic history and species composition of the family Strigeidae have been complex and unsettled. Recent molecular evidence suggests that the family is paraphyletic. However, the genera Apharyngostrigea, Parastrigea and Strigea share a common ancestor (Blasco-Costa et al., Reference Blasco-Costa, Poulin and Presswell2016; Blasco-Costa & Locke, Reference Blasco-Costa, Locke, Rollinson and Stothard2017; Hernández-Mena et al., Reference Hernández-Mena, García-Varela and Pérez-Ponce de León2017; Locke et al., Reference Locke, Drago and López-Hernández2021; López-Jiménez et al., Reference López-Jiménez, González-García and García-Varela2022). The genetic library of some strigeid species of the genera Apharyngostrigea, Parastrigea and Strigea has recently increased and provides a large opportunity to clarify the taxonomy and species composition of these three genera (Blasco-Costa et al., Reference Blasco-Costa, Poulin and Presswell2016; Hernández-Mena et al., Reference Hernández-Mena, García-Varela and Pérez-Ponce de León2017; Locke et al., Reference Locke, Drago and López-Hernández2021; López-Jiménez et al., Reference López-Jiménez, González-García and García-Varela2022). In the current study, we combined morphological and molecular characteristics to describe a new species, S. magnirostris n. sp. that represents the first species in the Neotropical region of Mexico and the 22nd in the Americas. Morphologically, the new species is distinguished from other congeneric species from the Americas by having an oral sucker with several papillae around it, well-developed pseudosuckers, a tegument covered with tiny spines, a larger cone genital and a larger copulatory bursa. In addition, the phylogenetic trees established with three molecular markers supported that the isolates identified morphologically as P. macrobursa from B. urubitinga (type host) and B. anthracinus collected from three localities in Mexico are not closely related to other members of the genus Parastrigea because they were nested inside Strigea. Therefore, we transferred it to Strigea to form S. macrobursa n. comb., expanding its geographical distribution from Mexico to Argentina (Drago & Lunaschi, Reference Drago and Lunaschi2011), representing the first record in Mexico. Interestingly, our phylogenies established that S. magnirostris n. sp., S. macrobursa n. comb. and Strigea sp. were associated with accipitriform and falconiform birds from the Neotropical region on a clade, suggesting that at least two clades could be formed, one represented by the 22 described species from the Neotropical region and the second represented by the six species from the Nearctic region in the Americas. However, this hypothesis should be tested with more species from other biogeographical regions and primarily adult specimens because the sequences from the LSU available in GenBank are from larval forms of Strigea spp. (KT362372, MT075841 and MK585230) (Patrelle et al., Reference Patrelle, Portier, Jouet, Delorme and Ferte2015; Svinin et al., Reference Svinin, Bashinskiy and Litvinchuk2020).
Heneberg et al. (Reference Heneberg, Sitko, Těšínský, Rzad and Bizos2018) performed one of the most comprehensive taxonomic reviews of strigeids that included samples of the genera Strigea, Parastrigea, Apharyngostrigea, Cotylurus Szidat, 1928 and Apatemon Szidat, 1928 from Central Europe. These authors sequenced the small subunit and the ITS2 from nuclear ribosomal DNA and the second region of the barcode from cox 1 and nicotinamide adenine dinucleotide dehydrogenase subunit 1 from mitochondrial DNA. However, these authors could not compare their sequences with other sequences of strigeids previously analysed by Hernández-Mena et al. (Reference Hernández-Mena, García-Prieto and García-Varela2014, Reference Hernández-Mena, García-Varela and Pérez-Ponce de León2017) and Blasco-Costa et al. (Reference Blasco-Costa, Poulin and Presswell2016) because these authors sequenced the ITS (ITS1-5.8S rDNA- ITS2), the domains D1–D3 of the LSU from nuclear DNA and the cox 1 barcode, the first region from the mitochondrial DNA. These three molecular markers have proven very useful for delineating species and inferring phylogenetic relationships at the genus level within Strigeidae. Herein, we compared the sequences of Heneberg et al. (Reference Heneberg, Sitko, Těšínský, Rzad and Bizos2018) with other sequences available in GenBank and with the newly generated sequences. We generated two new alignments. The first includes 60 sequences of ITS2 with 320 bp, representing 30% (1042 bp) of our original dataset that contains ITS1-5.8S rDNA-ITS2. Our phylogenetic trees established with ITS2 were similar to the tree inferred by Heneberg et al. (Reference Heneberg, Sitko, Těšínský, Rzad and Bizos2018), including the polyphyly of Strigea, with weak bootstrap support and posterior probabilities. In addition, the isolates of the new species S. magnirostris n. sp. plus S. macrobursa formed a clade together with S. falconis (MF628087) (see online supplementary fig. S1). The second alignment contained 46 sequences of cox 1 (including the newly generated sequences in the current study) with 297 bp of the second region of the barcode. The phylogenetic trees placed all the species of Strigea, including the new species S. magnirostris n. sp. and S. macrobursa in a clade. However, the species Parastrigea flexilis Dubois, 1934 (MF628065) was nested inside Strigea, suggesting that P. flexilis should be transferred to Strigea (see online supplementary fig. S2). To clarify the taxonomy of the genera Strigea, Parastrigea and Apharyngostrigea, it is necessary to review the taxonomy of the species that share diagnostic characteristics among the three genera. For instance, Parastrigea is characterized by the distribution of vitellaria (two symmetrical masses on the forebody), which are present in S. falconis, S. strigis (Schrank, 1788) Abildgaard, 1790, S. robusta (Szidat, 1928) Heneberg and Sitko, 2018, Apharyngostrigea brasiliana Szidat, 1928 (Dubois, 1964) and S. macrobursa n. comb., (Dubois, Reference Dubois1968; Heneberg et al., Reference Heneberg, Sitko, Těšínský, Rzad and Bizos2018; López-Jiménez et al., Reference López-Jiménez, González-García and García-Varela2022).
In summary our phylogenetic trees established with ITS and cox 1 supported the monophyly of Strigea. However, the LSU tree showed that Strigea is paraphyly because two sequences of larval forms identified as S. robusta (MT075841 and MK585230) were nested inside other clades. In addition, the genetic divergence among the species of the first clade of Strigea, S. magnirostris n. sp., S. macrobursa and two isolates of Strigea sp. (MF398343 and KT362372), ranged from 0.6% to 1.6% and from 2.4% to 2.8% with respect to S. robusta. These high ranges of divergence are similar between Strigea and Apharyngostrigea, which ranged from 1.9% to 2.2% for the LSU marker. The phylogenetic analyses established with the LSU, in combination with the high genetic divergence, of the two sequences of larval forms identified as S. robusta suggests that they do not belong to Strigea. However, the ITS2 tree (see online supplementary file S1) placed five isolates of S. robusta of adult and larval forms (MF537205, MT075803, MK295777, MF537208 and MF628100) from Germany, Russia and Poland in a single clade that is a sister to the type species.
In the current study, we described a new species of Strigea, collected from the intestines of two hawk species (R. magnirostris and A. coperii) which is named S. magnirostris n. sp. In addition, the species P. macrobursa was transferred to Strigea to form S. macrobursa n. comb. To clarify the taxonomy of the genus Strigea, it is necessary to sequence more species (including the type species, S. strigis) from diverse biogeographical regions with the ITS (ITS1-5.8S rDNA- ITS2), the D1–D3 domains from the LSU and the first region from the cox 1 gene. Finally, the current integrative study represents a continuation of our effort in describing and understanding the biodiversity of strigeids in the Neotropical region.
Supplementary material
To view supplementary material for this article, please visit https://doi.org/10.1017/S0022149X23000196.
Acknowledgements
We are grateful to Laura Marquez and Nelly López for their help during the sequencing of the DNA fragments. We also thank Berenit Mendoza Garfias for her help in obtaining the scanning electron microphotographs.
Financial support
ALJ and MTGG thank the support of the Programa de Posgrado en Ciencias Biológicas, UNAM and CONACYT (ALJ. CVU. No. 706119; MTGG. CVU. No. 956064) for granting a scholarship to complete her PhD program and his Master program, respectively. LAG thanks the Coordinación de la Investigación Científica and Dirección General de Asuntos de Personal Académico (DGAPA-UNAM) Mexico for the Postdoctoral Fellowship granted. This research was supported by the Programa de Apoyo a Proyectos de Investigación e Innovación Tecnológica (PAPIIT-UNAM) IN201122.
Competing interests
None.
Author contributions
ALJ and MGV conceived and designed the study. ALJ and MTGG conducted data gathering. ALJ, LAG and MTGG performed statistical analyses. ALJ, LAG and MGV wrote and edited the article. ALJ, MTTG, LAG and MGV collected the samples. ALJ performed the methodology.
Ethical standards
The sampling in this work complies with the current laws and animal ethics regulations of Mexico.