Trial Registration:
The meta-analysis was pre-registered on the international Prospective Register of Systematic Reviews (PROSPERO) (2023 CRD42023426953).
The randomized-controlled trial on which the current analysis is based on was registered in the International Clinical Trials Database, ClinicalTrials.gov (NCT number: NCT03466112, https://clinicaltrials.gov/ct2/show/NCT03466112?term=NCT03466112&draw=2&rank=1) and in the German Clinical Trials Register (DRKS-ID: DRKS00009804).
Data Set Information:
Details on the study design and data set are available under the following link: 10.1007/s00406-020-01175-2.
Introduction
The hippocampal formation (HF) is a key brain region that consists of several subfields such as the cornu ammonis (CA1–4), the dentate gyrus (DG) or the subiculum complex (Schultz & Engelhardt, Reference Schultz and Engelhardt2014). The HF plays a fundamental role in long-term episodic, semantic and short-term memory as well as spatial processing (Bird & Burgess, Reference Bird and Burgess2008). In this context, the HF subregions contribute differently to particular domains of memory functioning (Duncan, Ketz, Inati, & Davachi, Reference Duncan, Ketz, Inati and Davachi2012; Eldridge, Engel, Zeineh, Bookheimer, & Knowlton, Reference Eldridge, Engel, Zeineh, Bookheimer and Knowlton2005; Hainmueller & Bartos, Reference Hainmueller and Bartos2020; Zeineh, Engel, Thompson, & Bookheimer, Reference Zeineh, Engel, Thompson and Bookheimer2003).
In schizophrenia, volume reductions in the HF represent a consistent endophenotype (Adriano, Caltagirone, & Spalletta, Reference Adriano, Caltagirone and Spalletta2012; Brugger & Howes, Reference Brugger and Howes2017; Chopra et al., Reference Chopra, Segal, Oldham, Holmes, Sabaroedin, Orchard and Fornito2023; Honea, Crow, Passingham, & Mackay, Reference Honea, Crow, Passingham and Mackay2005; van Erp et al., Reference van Erp, Hibar, Rasmussen, Glahn, Pearlson, Andreassen and Turner2016), with a particularly pronounced decline in the CA1–4, DG, and subiculum (Falkai et al., Reference Falkai, Steiner, Malchow, Shariati, Knaus, Bernstein and Schmitt2016; Haukvik, Tamnes, Söderman, & Agartz, Reference Haukvik, Tamnes, Söderman and Agartz2018; Ho et al., Reference Ho, Iglesias, Sum, Kuswanto, Sitoh, De Souza and Holt2017; Mathew et al., Reference Mathew, Gardin, Tandon, Eack, Francis, Seidman and Keshavan2014; Nakahara et al., Reference Nakahara, Turner, Calhoun, Lim, Mueller, Bustillo and van Erp2020; Nakahara, Matsumoto, & van Erp, Reference Nakahara, Matsumoto and van Erp2018; Roeske, Konradi, Heckers, & Lewis, Reference Roeske, Konradi, Heckers and Lewis2021; Schmitt et al., Reference Schmitt, Tatsch, Vollhardt, Schneider-Axmann, Raabe, Roell and Schmitz2022). Decreases of both global and subfield volumes have been associated with impairments in numerous cognitive domains such as verbal short- and long-term memory, and processing speed (Antoniades et al., Reference Antoniades, Schoeler, Radua, Valli, Allen, Kempton and McGuire2018; Haukvik et al., Reference Haukvik, Tamnes, Söderman and Agartz2018; Khalil, Hollander, Raucher-Chéné, Lepage, & Lavigne, Reference Khalil, Hollander, Raucher-Chéné, Lepage and Lavigne2022; Nakahara et al., Reference Nakahara, Matsumoto and van Erp2018, Reference Nakahara, Turner, Calhoun, Lim, Mueller, Bustillo and van Erp2020; Pijnenborg et al., Reference Pijnenborg, Larabi, Xu, Hasson-Ohayon, de Vos, Ćurčić-Blake and Van der Meer2020) as well as with increased severity of positive and negative symptoms (Haukvik et al., Reference Haukvik, Tamnes, Söderman and Agartz2018; Ho et al., Reference Ho, Iglesias, Sum, Kuswanto, Sitoh, De Souza and Holt2017; Nakahara et al., Reference Nakahara, Matsumoto and van Erp2018).
In the last two decades, aerobic exercise interventions have been increasingly proposed as a promising add-on treatment to improve psychopathological symptoms and cognitive functioning in psychiatric disorders (Schmitt, Reich-Erkelenz, Hasan, & Falkai, Reference Schmitt, Reich-Erkelenz, Hasan and Falkai2019). Relevant large-scale evidence indicates that different types of exercise can improve psychiatric symptoms (Dauwan, Begemann, Heringa, & Sommer, Reference Dauwan, Begemann, Heringa and Sommer2016; Fernández-Abascal, Suárez-Pinilla, Cobo-Corrales, Crespo-Facorro, & Suárez-Pinilla, Reference Fernández-Abascal, Suárez-Pinilla, Cobo-Corrales, Crespo-Facorro and Suárez-Pinilla2021; Firth, Cotter, Elliott, French, & Yung, Reference Firth, Cotter, Elliott, French and Yung2015; Gallardo-Gómez et al., Reference Gallardo-Gómez, Noetel, Álvarez-Barbosa, Alfonso-Rosa, Ramos-Munell, Del Pozo Cruz and Del Pozo-Cruz2023; Guo, Liu, Liao, Qin, & Yue, Reference Guo, Liu, Liao, Qin and Yue2024; Kim, Lee, & Kang, Reference Kim, Lee and Kang2023; Maurus et al., Reference Maurus, Roell, Lembeck, Papazova, Greska, Muenz and Falkai2023; Sabe, Kaiser, & Sentissi, Reference Sabe, Kaiser and Sentissi2020; Vogel et al., Reference Vogel, van der Gaag, Slofstra, Knegtering, Bruins and Castelein2019; Wei et al., Reference Wei, Yang, Imm, Loprinzi, Smith, Zhang and Yu2020; Ziebart et al., Reference Ziebart, Bobos, MacDermid, Furtado, Sobczak and Doering2022), cognition (Fernández-Abascal et al., Reference Fernández-Abascal, Suárez-Pinilla, Cobo-Corrales, Crespo-Facorro and Suárez-Pinilla2021; Firth et al., Reference Firth, Cotter, Elliott, French and Yung2015; Shimada et al., Reference Shimada, Ito, Makabe, Yamanushi, Takenaka, Kawano and Kobayashi2022; Xu et al., Reference Xu, Cai, Fang, Zheng, Shan and Yang2022) and overall functioning (Dauwan et al., Reference Dauwan, Begemann, Heringa and Sommer2016; Fernández-Abascal et al., Reference Fernández-Abascal, Suárez-Pinilla, Cobo-Corrales, Crespo-Facorro and Suárez-Pinilla2021; Korman et al., Reference Korman, Stanton, Vecchio, Chapman, Parker, Martland and Firth2023) in people with schizophrenia, as is particularly the case for aerobic exercise interventions (Firth et al., Reference Firth, Stubbs, Rosenbaum, Vancampfort, Malchow, Schuch and Yung2017b; Sabe et al., Reference Sabe, Kaiser and Sentissi2020; Shimada et al., Reference Shimada, Ito, Makabe, Yamanushi, Takenaka, Kawano and Kobayashi2022; Vogel et al., Reference Vogel, van der Gaag, Slofstra, Knegtering, Bruins and Castelein2019; Xu et al., Reference Xu, Cai, Fang, Zheng, Shan and Yang2022).
Beneficial effects of aerobic exercise on clinical health outcomes related to schizophrenia may be driven by improvements in physical health (Vancampfort, Rosenbaum, Ward, & Stubbs, Reference Vancampfort, Rosenbaum, Ward and Stubbs2015) and by multiple neural adaptations that occur in response to aerobic exercise treatments (Firth, Cotter, Carney, & Yung, Reference Firth, Cotter, Carney and Yung2017a; Maurus et al., Reference Maurus, Hasan, Röh, Takahashi, Rauchmann, Keeser and Falkai2019). Specifically, aerobic exercise interventions in people with schizophrenia have been found to increase the volume in the HF and its subfields, which is accompanied by improvements in short-term memory, working memory and overall functioning (Falkai et al., Reference Falkai, Maurus, Schmitt, Malchow, Schneider-Axmann, Röll and Keeser2021; Khonsari et al., Reference Khonsari, Badrfam, Mohammdi, Rastad, Etemadi, Vafaei and Zandifar2022; Lin et al., Reference Lin, Chan, Lee, Chang, Tse, Su and Chen2015; Pajonk et al., Reference Pajonk, Wobrock, Gruber, Scherk, Berner, Kaizl and Falkai2010; Woodward et al., Reference Woodward, Gicas, Warburton, White, Rauscher, Leonova and Lang2018). However, the body of literature as a whole remains heterogeneous, as several studies also report null-results in both patients with manifested schizophrenia (Malchow et al., Reference Malchow, Keeser, Keller, Hasan, Rauchmann, Kimura and Falkai2016; Rosenbaum et al., Reference Rosenbaum, Lagopoulos, Curtis, Taylor, Watkins, Barry and Ward2015; Scheewe et al., Reference Scheewe, van Haren, Sarkisyan, Schnack, Brouwer, de Glint and Cahn2013) and people at clinical high-risk for psychosis (Damme et al., Reference Damme, Gupta, Ristanovic, Kimhy, Bryan and Mittal2022; Dean et al., Reference Dean, Bryan, Newberry, Gupta, Carol and Mittal2017). Moreover, only a few studies included the HF subfields in their respective analyses despite their potential clinical relevance in schizophrenia (Damme et al., Reference Damme, Gupta, Ristanovic, Kimhy, Bryan and Mittal2022; Malchow et al., Reference Malchow, Keeser, Keller, Hasan, Rauchmann, Kimura and Falkai2016; Woodward et al., Reference Woodward, Gicas, Warburton, White, Rauscher, Leonova and Lang2018).
In order to summarize the current heterogeneous state of research, we conducted a systematic review and meta-analysis and analyzed data from our recent multicenter randomized-controlled exercise study in people with schizophrenia. First, we examined if aerobic exercise increases global hippocampal formation volume in schizophrenia. We addressed said question by means of synthesizing existing literature and our present findings in a meta-analysis. Second, based on our current study, we investigated if certain HF subfield volumes are particularly responsive to aerobic exercise. Third, we explored if increases in individual HF subfield volumes after six months of aerobic exercise are related to changes in clinical symptom severity, cognitive performance, and levels of general functioning.
Methods and materials
Meta-analysis
Search strategy
Our meta-analysis was pre-registered on the international Prospective Register of Systematic Reviews (PROSPERO) (2023 CRD42023426953). Preferred Reporting Items for Systemic Reviews and Meta-Analyses (PRISMA) standards were adhered to throughout the review process. Using the following keywords: ‘Schizophrenia’ OR ‘Psychosis’ AND ‘Aerobic exercise’ OR ‘Physical activity’ OR ‘Fitness’ AND ‘Hippocampus’ OR ‘Hippocampal volume’, TF and LR searched the ensuing databases: Cochrane, PubMed, Web of Science, ISRCTN, and Embase from inception until 25th April 2023 for published and unpublished literature. After removal of duplicates, titles and abstracts were reviewed independently by TF and LR using Rayyan software (Ouzzani, Hammady, Fedorowicz, & Elmagarmid, Reference Ouzzani, Hammady, Fedorowicz and Elmagarmid2016). Full-text papers were identified according to pre-specified eligibility criteria and primary outcome. In case of disagreement, a third reviewer (IM) was available to mediate. Data extraction began on 26th May 2023.
Eligibility criteria
Inclusion criteria comprised studies that: (1) focused on schizophrenia spectrum disorder, (2) included an aerobic exercise intervention at moderate intensity, e.g. bicycle ergometer, treadmill or similar, (3) included a control condition that does not amount to aerobic exercise, e.g. waitlists, table soccer, (4) reported total hippocampal formation volume assessed via structural magnetic resonance imaging and analyzed by means of manual or automated segmentation. Moreover, it was required that studies were published in English language and included an adult participant sample 18+ years of age.
Risk of bias and quality assessment
The most recent edition of the Cochrane risk-of-bias tool for randomized trials (RoB 2) was used to assess risk of bias (Sterne et al., Reference Sterne, Savović, Page, Elbers, Blencowe, Boutron and Higgins2019). The tool encompasses five domains: randomization process, deviations from intended interventions, missing outcome data, measurement of the outcome, and selection of the reported result. Based on answers to signaling questions, an algorithm determined the extent of bias relevant to a particular domain as well as an overall bias score ranging from ‘low risk of bias’ to ‘some concerns’ and ‘high risk of bias’ (online Supplementary Figs S1 and S2). Both TF and LR completed the risk of bias assessment using the RoB 2.0 excel template. Inconsistencies were resolved by means of discussion.
Data extraction
Our primary outcome was the change in total hippocampal formation volume from pre- to post-intervention. The following data were extracted independently from included reports by TF and LR: sample sizes, means and standard deviations of primary outcome data prior to and post aerobic exercise interventions for the experimental and control groups. If said data were not made available in the initial report, authors were contacted by either TF or LR.
Statistical analysis
Comprehensive meta-analysis software (V4) was used to conduct random-effects meta-analyses (Borenstein, Reference Borenstein, Egger, Higgins and Smith2022) based on all trials with control condition comparing the effects on hippocampal formation volume in an aerobic exercise intervention to a study-specific control group. Hedges' g statistic was computed using intervention effect sizes (differences between intervention and control groups) for the primary outcome measurement, as were 95% confidence intervals (CIs). Effect sizes expressed as Hedges' g were classified as small (0.2 ⩽ g < 0.5), medium (0.5 ⩽ g < 0.8), or large (g > 0.8). A sensitivity analysis was conducted by means of removing one study at a time in order to assess robustness of observed effects. Heterogeneity was assessed using Cochran's Q and Higgins' I2, which can be divided into considerable (>75%), substantial (50–75%), moderate (30–50%), and low (< 30%). Publication bias was addressed via producing a funnel plot and assessing asymmetry using Egger's regression coefficient as well as determining Begg and Mazumdar's rank correlation value with p < 0.05 indicative of bias (Begg & Mazumdar, Reference Begg and Mazumdar1994; Egger, Davey Smith, Schneider, & Minder, Reference Egger, Davey Smith, Schneider and Minder1997). In case of significant publication bias, a Duval and Tweedie trim-and-fill analysis was utilized (Duval & Tweedie, Reference Duval and Tweedie2000). Moreover, in exploratory intention, we conducted a second meta-analysis which included studies without control conditions (Rosenbaum et al., Reference Rosenbaum, Lagopoulos, Curtis, Taylor, Watkins, Barry and Ward2015; Woodward et al., Reference Woodward, Gicas, Warburton, White, Rauscher, Leonova and Lang2018) and underaged participants (Dean et al., Reference Dean, Bryan, Newberry, Gupta, Carol and Mittal2017). This analysis was based on all available studies comparing the HF volume prior to the aerobic exercise intervention with the volume after the aerobic exercise intervention. The rationale behind the exploratory analysis was the limited number of studies surrounding the effect of aerobic exercise compared to a control condition on HF volume in schizophrenia and the low risk of placebo effects when investigating HF volume as a standalone outcome.
Randomized-controlled trial
Study design and sample
The data presented here originate from the Enhancing Schizophrenia Prevention and Recovery through Innovative Treatments (ESPRIT) C3 study (NCT03466112, https://clinicaltrials.gov/ct2/show/NCT03466112?term=NCT03466112&draw=2&rank=1). The ESPRIT C3 study is a multicenter randomized-controlled trial that investigates the effects of aerobic exercise on multiple health outcomes in people with schizophrenia. The authors assert that all procedures contributing to this work comply with the ethical standards of the relevant national and institutional committees on human experimentation and with the Helsinki Declaration of 1975, as revised in 2008. All procedures involving human patients were approved by the ethics committee of the medical faculty of the Ludwig-Maximilians-University Munich (approval number: 706-15).
In total 180 participants gave written informed consent and were randomly assigned to either an aerobic endurance training (AET) or to a flexibility, strengthening and balance training (FSBT). Supervised by a sport scientist, both groups exercised up to three times per week, for approximately 40–50 min per session, for a total duration of six months. Patients in the AET group cycled on a stationary bicycle ergometer at a moderate exercise intensity which was determined by a lactate threshold test prior to the onset of the intervention. In the FSBT group, patients performed exercises covering stretching, mobility, stability, balance, and relaxation (Liu-Ambrose et al., Reference Liu-Ambrose, Nagamatsu, Graf, Beattie, Ashe and Handy2010). Ninety nine subjects initially agreed to undergo MRI sessions, but evaluable structural T1-weighted MR images from which HF subfield volumes could be computed at baseline and post-intervention were available for only 29 participants (9 in the AET group, 20 in the FSBT group). Table 1 depicts the sample characteristics. Details of the ESPRIT C3 study are described in the supplemental information and in the corresponding study design publication (Maurus et al., Reference Maurus, Hasan, Schmitt, Roeh, Keeser, Malchow and Falkai2020).
Table 1. Sample characteristics

AET, aerobic endurance training; FSBT, flexibility, strengthening and balance training; PANSS, Positive and Negative Syndrome Scale; pfisher, p value of Fisher's exact test for categorical data; p wilcox, p value of Wilcoxon signed-rank test for numeric data; s.d., standard deviation. The sample sizes per group refer to the number of participants that were considered for the statistical data analysis.
Structural MRI data and clinical variables
The hippocampal module of FreeSurfer v7.2 (Iglesias et al., Reference Iglesias, Augustinack, Nguyen, Player, Player, Wright and Van Leemput2015) was used to compute the volumes of HF subfields at a spatial isotropic resolution of 0.8 mm3 at study site Munich and 1 mm3 at study sites Mannheim and Berlin. Based on the proportion method (O'Brien et al., Reference O'Brien, Ziegler, Deutsch, Frazier, Herbert and Locascio2011), HF volumes were corrected via intracranial volume across all subjects and sessions and volumes of bodies and heads were summed up for each subfield. Because the FreeSurfer segmentation of all HF subfields based on MRI scans at a spatial isotropic resolution of 1 mm3 has been criticized due to issues regarding the detection of the boundaries between subfields (Wisse et al., Reference Wisse, Chételat, Daugherty, de Flores, la Joie, Mueller and Carr2021), we conducted an additional analysis segmenting the HF only into head, body, and tail.
The Positive and Negative Syndrome Scale (PANSS) was administered to assess positive and negative symptoms, and total symptom severity (Kay, Fiszbein, & Opler, Reference Kay, Fiszbein and Opler1987). Verbal semantic long-term memory comprised a composite score of the sixth and seventh run of the Verbal Learning and Memory Test (VLMT), whereas the average of the first and interference run was utilized to cover verbal semantic short-term memory (Helmstaedter & Durwen, Reference Helmstaedter and Durwen1990). The backward version of the Digit Span Test (DST) was employed to target verbal working memory (Tewes, Reference Tewes1994). The Global Assessment of Functioning scale (GAF) and the Functional Remission of General Schizophrenia scale (FROGS) were applied to assess general functioning (Endicott, Spitzer, Fleiss, & Cohen, Reference Endicott, Spitzer, Fleiss and Cohen1976; Llorca et al., Reference Llorca, Lançon, Lancrenon, Bayle, Caci, Rouillon and Gorwood2009). Further details on MRI data acquisition (online Supplementary Table S2), processing, and quality control procedures, as well as on the cognitive test batteries are provided in the supplemental information.
Statistical analysis
Statistical analysis was performed with Rstudio v1.4.1717 based on R v4.2.2 (R Core Team, 2021; RStudio Team, 2020) and visualizations were created with ggplot2 (Wickham, Reference Wickham2016).
To investigate the effect of AET on HF subfield volumes in comparison to FSBT (2nd research question), linear mixed effect models for repeated measures were calculated. Group (AET, FSBT), time (t0: baseline, t6: six months), the group × time interaction, age, sex, chlorpromazine equivalents, number of trainings, volume at baseline, study site (Munich, Mannheim, Berlin), and hemisphere (left, right) were included as predictors, while the corresponding five subfield volumes served as dependent variables. p values of the factor time and the group × time interaction were adjusted separately across the five linear mixed models using the false discovery rate (FDR) method (Benjamini & Hochberg, Reference Benjamini and Hochberg1995). In case of a significant group × time interaction (p fdr < 0.05) Tukey post-hoc tests within groups and between sessions were conducted.
Regarding the potential effects of individual changes in HF subfield volumes on clinical outcomes (3rd research question), we computed multiple linear regression models to examine the general associations between changes in HF subfield volume and changes in psychiatric symptoms, cognition, and functioning. Respective corresponding subfield volume differences between t0 and t6, group, age, sex, chlorpromazine equivalents, training number, study site, and years of education served as predictors. The difference in the corresponding behavioral scores between t0 and t6 was utilized as the dependent variable. p values of the volume differences were FDR-corrected.
Results
Search results, included studies and participant details
As shown in Fig. 1, a total of 532 records were identified across five platforms. After removal of 400 duplicates, TF and LR screened 132 titles and abstracts, based on which an additional 120 studies were excluded. Of the remaining 12 records, 2 were not retrievable, 2 control conditions collapsed during primary analysis due to small sample sizes, 1 report included patients under the age of 18, and another only reported particular HF subfields. Thus, a total of six records were included in the primary analysis. The overall sample comprised 186 patients, 92 of which were assigned to aerobic exercise and 94 to control conditions. In total, 46% identified as female (n = 86) and 54% as male (n = 100), patients' mean age ranged from 24.6 to 39.3, and attrition rates from 0% to 79%. Studies were conducted in Germany (3x), Hong Kong, Iran, and the Netherlands (Khonsari et al., Reference Khonsari, Badrfam, Mohammdi, Rastad, Etemadi, Vafaei and Zandifar2022; Lin et al., Reference Lin, Chan, Lee, Chang, Tse, Su and Chen2015; Malchow et al., Reference Malchow, Keeser, Keller, Hasan, Rauchmann, Kimura and Falkai2016; Pajonk et al., Reference Pajonk, Wobrock, Gruber, Scherk, Berner, Kaizl and Falkai2010; Scheewe et al., Reference Scheewe, van Haren, Sarkisyan, Schnack, Brouwer, de Glint and Cahn2013). Detailed patient and intervention study characteristics included in the meta-analyses can be found in online Supplementary Table S1. Risk of bias assessment suggested high concerns for three reports including our original data due to high attrition rates (Lin et al., Reference Lin, Chan, Lee, Chang, Tse, Su and Chen2015; Scheewe et al., Reference Scheewe, van Haren, Sarkisyan, Schnack, Brouwer, de Glint and Cahn2013). Detailed results of the RoB analysis can be found in online Supplementary Figs S1 and S2.

Figure 1. PRISMA flow diagram showcasing the process of identifying literature with total hippocampal formation volume as the primary outcome. A total of 6 studies were included in the meta-analysis.
Effect of aerobic exercise on global hippocampal formation volume assessed in meta-analysis
As shown in Fig. 2a, the between-groups random-effects meta-analysis (k = 6) revealed a small to medium but non-significant effect of exercise interventions on global hippocampal formation volume (Hedges' g = 0.33, 95% CI −0.12 to 0.77, p = 0.15). Heterogeneity was substantial (Q = 11.52, df = 5, p = 0.04, I2 = 56.58), whereas publication bias was non-significant (Kendall's tau = 0.27, p = 0.45). A funnel plot (online Supplementary Fig. S3), explored for asymmetry by means of Egger's Test, confirmed a lack of publication bias (Egger's intercept = 3.31, s.e. = 4.72, p = 0.52). Via removing one study at a time, the effect size of aerobic exercise on hippocampal volume ranged from 0.10 to 0.43.
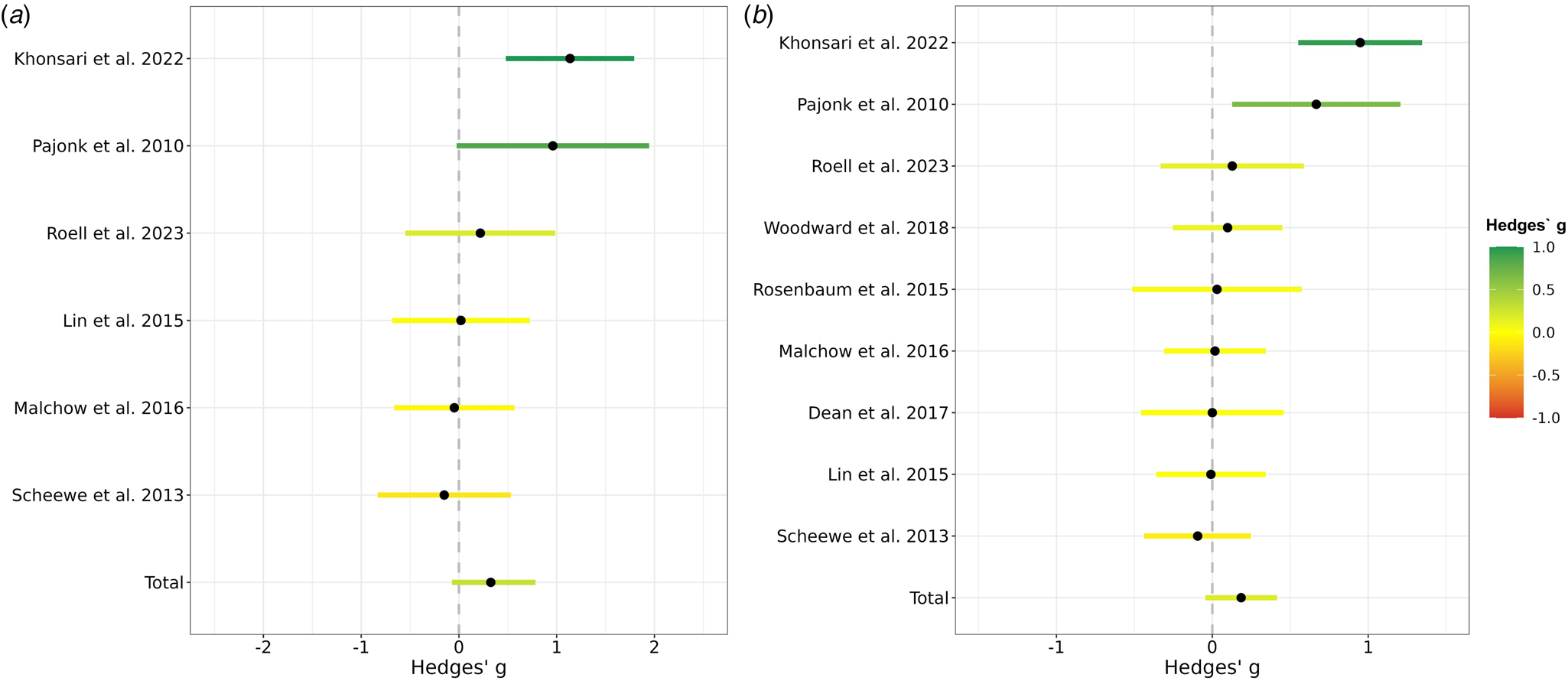
Figure 2. Effects of aerobic exercise on total hippocampal formation volume in people with schizophrenia. (a) Meta-analysis based on all trials with control condition comparing the effects on hippocampal formation volume in an aerobic exercise intervention to a study-specific control group. A positive Hedges g indicates that the volume increase in the aerobic exercise group was higher than in the control group. The 95% confidence intervals are represented by the colored lines. The pooled effect across all available studies is shown at the bottom and is labeled with ‘Total’. Roell et al. (2024) refers to the original data presented here. (b) Meta-analysis based on all available studies comparing the hippocampal formation volume prior to the aerobic exercise intervention with the volume after the aerobic exercise intervention. A positive Hedges' g indicates that there was a volume increase from baseline to the end of the intervention within the aerobic exercise group. The lines reflect the 95% confidence intervals. The pooled effect across all available studies is shown at the bottom and is labeled with ‘Total’. Roell et al. (2024) refers to the original data presented here.
As illustrated in Fig. 2b, the within-group random-effects meta-analysis (k = 9) revealed a small but non-significant effect of exercise interventions on global hippocampal formation volume in schizophrenia and related disorders (Hedges' g = 0.19, 95% CI −0.05 to 0.42, p = 0.11). Heterogeneity was substantial (Q = 23.08, df = 8, p < 0.003, I2 = 65.34), whereas publication bias was non-significant (Kendall's tau = 0.42, p = 0.12). A funnel plot (online Supplementary Fig. S4), explored for asymmetry by means of Egger's Test, confirmed a lack of publication bias (Egger's intercept = 2.98, s.e. = 3.22, p = 0.39). Via removing one study at a time, the effect size of aerobic exercise on hippocampal volume ranged from 0.06 to 0.23. All test statistics are available in online Supplementary Table S3.
Effect of aerobic exercise on hippocampal formation subfield volumes assessed in RCT
After FDR correction, we observed significant effects for the factor time and group × time interaction for the following subfields: CA1 (F = 5.11, p FDR = 0.033), CA2/3 (F = 7.62, p FDR = 0.033), CA4 (F = 5.29, p FDR = 0.033), and DG (F = 5.67, p FDR = 0.033). Thus, indicating significant differences in volume changes between AET and FSBT groups over time regarding all subfields except for the subiculum. Tukey post-hoc tests revealed significant volume increases between timepoints t0 and t6 for CA1 (d = 0.89, CI [0.22–1.55], p tukey = 0.009), CA2/3 (d = 1.27, CI [0.61–1.93], p tukey < 0.001), CA4 (d = 1.06, CI [0.40–1.73], p tukey = 0.002), and DG (d = 1.04, CI [0.38–1.70], p tukey = 0.002) in the AET, but not in the FSBT group. Figure 3 illustrates the effects on HF volumes in both exercise groups and Fig. 4 visualizes the subfields CA1–4 and the DG.
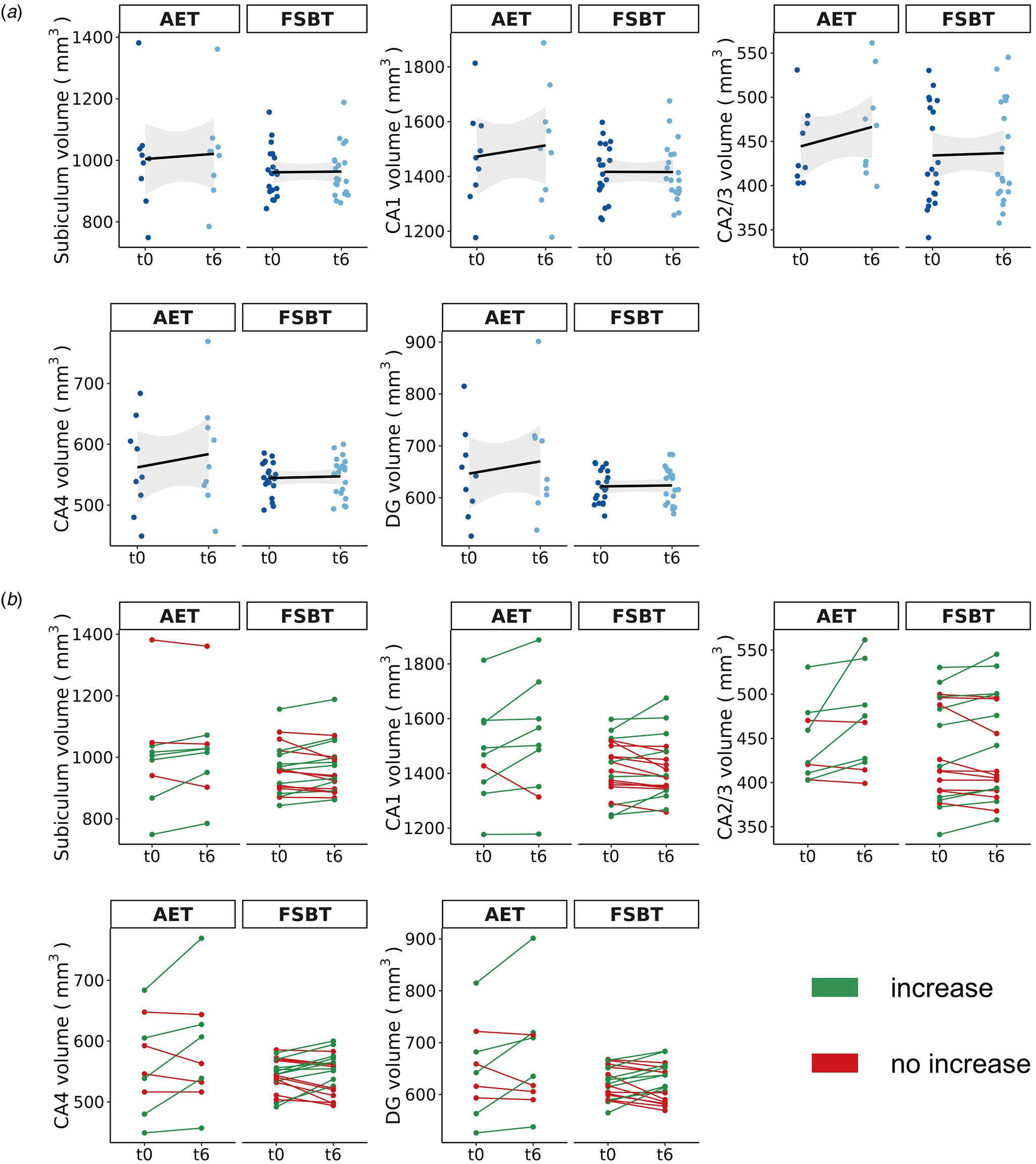
Figure 3. Exercise-induced volume changes of the HF subfields. AET, aerobic endurance training, FSBT, flexibility, strengthening and balance training; CA, cornu ammonis; DG, dentate gyrus; t0, baseline time point; t6, time point after six months of exercise. (a) The mean volume changes per subfield within each exercise group from time point t0 to t6 are shown. The shadowed area represents the 95% confidence interval. (b) The individual volume changes per subfield within each exercise group from time point t0 to t6 are displayed.

Figure 4. Hippocampal formation subfields. Sagittal (left), axial (middle) and coronal (right) view of the segmentation of CA1–4 and the DG. The subiculum is not displayed because no exercise effects were observed in this case. CA1–4 and DG are separated into head and body in this figure, but volumes from both parts were summed up for the statistical analysis. Images were acquired at a spatial isotropic resolution of 0.8 mm3 at study site Munich and 1 mm3 at study sites Mannheim and Berlin. CA, cornu ammonis; DG, dentate gyrus.
We did not observe any significant effects for the factor hemisphere (CA1: p = 0.864, CA2/3: p = 0.658, CA4: p = 0.335, DG: p = 0.392), suggesting that volume changes occur bilaterally to the same extent.
As part of our additional analysis, Tukey post-hoc tests indicated significant volume increases in the aerobic exercise group between timepoint t0 and t6 for the hippocampal head (d = 0.86, CI [0.20–1.53], p tukey = 0.010) and body (d = 1.16, CI [0.50–1.82], p tukey = 0.001), but not for the tail (d = 0.50, CI [−0.16 to 1.16], p tukey = 0.137).
Clinical relevance of changes in HF volumes online Supplementary Table S4 and Figs S7, S8 and S9 summarize the full test statistics of the multiple linear regressions and the associations between changes in HF subfield volumes and changes in symptoms, cognition, and functioning, respectively. No stable correlations between longitudinal volumetric and psychopathological or cognitive changes were observed.
Discussion
Our meta-analysis reveals that there was no significant effect of aerobic exercise on total HF volume. Rather, preliminary data from our randomized-controlled trial suggested that aerobic exercise may lead to significant volume increases in certain HF subfields, namely CA1, CA2/3, CA4, and DG. However, the clinical impact of said volume changes remains subject to further investigation.
Structural deteriorations within the HF play a key role in the pathophysiology of schizophrenia and contribute to cognitive deficits as well as positive and negative symptom severity (Adriano et al., Reference Adriano, Caltagirone and Spalletta2012; Antoniades et al., Reference Antoniades, Schoeler, Radua, Valli, Allen, Kempton and McGuire2018; Brosch et al., Reference Brosch, Stein, Schmitt, Pfarr, Ringwald, Thomas-Odenthal and Kircher2022; Brugger & Howes, Reference Brugger and Howes2017; Haukvik et al., Reference Haukvik, Tamnes, Söderman and Agartz2018; Ho et al., Reference Ho, Iglesias, Sum, Kuswanto, Sitoh, De Souza and Holt2017; Honea et al., Reference Honea, Crow, Passingham and Mackay2005; Khalil et al., Reference Khalil, Hollander, Raucher-Chéné, Lepage and Lavigne2022; Nakahara et al., Reference Nakahara, Matsumoto and van Erp2018, Reference Nakahara, Turner, Calhoun, Lim, Mueller, Bustillo and van Erp2020; Opel et al., Reference Opel, Goltermann, Hermesdorf, Berger, Baune and Dannlowski2020; Pijnenborg et al., Reference Pijnenborg, Larabi, Xu, Hasson-Ohayon, de Vos, Ćurčić-Blake and Van der Meer2020; van Erp et al., Reference van Erp, Hibar, Rasmussen, Glahn, Pearlson, Andreassen and Turner2016). Therefore, the implementation of interventions that promote hippocampal health constitute an essential approach towards improving treatment outcomes in schizophrenia. Although our meta-analysis did not demonstrate a significant effect of aerobic exercise on HF volume in people with schizophrenia, a noticeable trend in favor of aerobic exercise v. control conditions was observable. This is in line with previous meta-analyses conducted among various populations suggesting trends towards aerobic exercise-induced volume increases of the total HF (Firth et al., Reference Firth, Stubbs, Vancampfort, Schuch, Lagopoulos, Rosenbaum and Ward2018; Li et al., Reference Li, Huang, Li, Tao, Zheng and Chen2017), but heterogeneity between studies is high. For instance, the included trials differ in several important properties such as the duration and the exact type of the utilized aerobic exercise programs, the kind of control group or the clinical status of the underlying study population. We systematically reviewed these properties, aiming at identifying a pattern that may explain why certain trials observe HF volume increases and others do not, but based on the available information no stable and systematic pattern was detectable. Hence, so far, we can only conclude that aerobic exercise interventions in people with schizophrenia may not consistently lead to HF volume increases on a group level, but rather affect HF volumes only under certain conditions:
Firstly, temporally restricted aerobic exercise interventions in the context of scientific studies may not be sufficient to observe stable HF volume increases. Instead, large-scale evidence demonstrates that an increased HF volume in healthy subjects is associated with a higher aerobic fitness level which in turn depends on the individual's longitudinal engagement in aerobic exercise (Wittfeld et al., Reference Wittfeld, Jochem, Dörr, Schminke, Gläser, Bahls and Grabe2020). Our previous cross-sectional findings in people with schizophrenia support this notion (Maurus et al., Reference Maurus, Roell, Keeser, Papazov, Papazova, Lembeck and Falkai2022a, Reference Maurus, Röll, Keeser, Karali, Papazov, Hasan and Falkai2022b), insofar as improvements of aerobic fitness during temporarily restricted aerobic exercise interventions have been linked to more pronounced increases of HF volume (Pajonk et al., Reference Pajonk, Wobrock, Gruber, Scherk, Berner, Kaizl and Falkai2010). Correspondingly, a recent meta-analysis comprising studies of various populations suggested that aerobic exercise interventions with a minimum duration of at least six months lead to more consistent increases in HF volume (Wilckens et al., Reference Wilckens, Stillman, Waiwood, Kang, Leckie, Peven and Erickson2021). Hence, we conclude that stable increases in HF volume as elicited by aerobic exercise are subject to long-term processes not always observable across the whole study population in the context of time-restricted intervention studies. Therefore, future cohort studies examining the long-term disease course of schizophrenia should collect measures of physical activity and fitness to better understand how frequently and over what period of time aerobic exercise should be performed to observe stable restorative effects on the HF volume across the whole study sample.
Second, however, aerobic exercise-induced HF volume increases can still be achieved within a shorter period of time (Khonsari et al., Reference Khonsari, Badrfam, Mohammdi, Rastad, Etemadi, Vafaei and Zandifar2022; Pajonk et al., Reference Pajonk, Wobrock, Gruber, Scherk, Berner, Kaizl and Falkai2010), which may be attributable to the respective sample characteristics. Accordingly, previous evidence indicates that both a higher general polygenic risk for schizophrenia and a higher genetic burden associated with oligodendrocyte precursor cells and radial glia inhibit neuroplasticity within the HF during aerobic exercise (Papiol et al., Reference Papiol, Popovic, Keeser, Hasan, Schneider-Axmann, Degenhardt and Malchow2017, Reference Papiol, Keeser, Hasan, Schneider-Axmann, Raabe, Degenhardt and Falkai2019). These findings emphasize that certain genetic subgroups of patients are less likely to benefit from aerobic exercise interventions, which may in turn partially explain a lack of significance in observed effects on the group level. Hence, future research should identify multimodal factors that explain why certain patients with schizophrenia reveal increases in HF volume and others do not in order to enable individualized aerobic exercise treatments in future health care.
Thirdly, the potential effects of aerobic exercise on the HF may not be observable as a whole, but rather via subfields CA1, CA2/3, CA4 and DG, as indicated by our present preliminary study data. Accordingly, previous evidence indicates that different areas of the CA were particularly responsive to aerobic fitness and exercise (Maurus et al., Reference Maurus, Roell, Keeser, Papazov, Papazova, Lembeck and Falkai2022a; Woodward et al., Reference Woodward, Gicas, Warburton, White, Rauscher, Leonova and Lang2018), whereas the subiculum, for example, was not (Damme et al., Reference Damme, Gupta, Ristanovic, Kimhy, Bryan and Mittal2022). Moreover, there may also exist a hemisphere-specific effect of aerobic exercise on HF volume (Firth et al., Reference Firth, Stubbs, Vancampfort, Schuch, Lagopoulos, Rosenbaum and Ward2018; Li et al., Reference Li, Huang, Li, Tao, Zheng and Chen2017). With regard to the underlying mechanisms, beneficial structural adjustments within the HF may result from several exercise-induced neural adaptations such as upregulations of neurotrophic factors, facilitated neuroplastic processes including neurogenesis, angiogenesis and gliogenesis or increases in dendritic density and length (Kandola, Hendrikse, Lucassen, & Yücel, Reference Kandola, Hendrikse, Lucassen and Yücel2016; Liu & Nusslock, Reference Liu and Nusslock2018; Maurus et al., Reference Maurus, Hasan, Röh, Takahashi, Rauchmann, Keeser and Falkai2019). Additionally, ameliorations of physical health after aerobic exercise treatment (Vancampfort et al., Reference Vancampfort, Rosenbaum, Ward and Stubbs2015) may modulate neural plasticity in the HF subfields. Particularly, impaired physical and metabolic health plays a substantial role in schizophrenia (Tian et al., Reference Tian, Di Biase, Mosley, Lupton, Xia, Fripp and Zalesky2023) and thus can lead to widespread neural deteriorations in both brain structure and function (Herrmann, Tesar, Beier, Berg, & Warrings, Reference Herrmann, Tesar, Beier, Berg and Warrings2019; Li et al., Reference Li, Yu, Zhong, Liu, Wei, Meng and Wang2022; Parsons, Steward, Clohesy, Almgren, & Duehlmeyer, Reference Parsons, Steward, Clohesy, Almgren and Duehlmeyer2022; Syan et al., Reference Syan, McIntyre-Wood, Minuzzi, Hall, McCabe and MacKillop2021). Improving physical and metabolic health through aerobic exercise treatments may thus yield the potential to stimulate regenerative processes in relevant brain regions such as the HF. Considering that CA subfields are especially prone to volume loss in schizophrenia (Ho et al., Reference Ho, Iglesias, Sum, Kuswanto, Sitoh, De Souza and Holt2017; Park et al., Reference Park, Jeon, Khan, Dempster, Chakravarty, Lerch and Palaniyappan2021), it remains particularly important to emphasize that exercise-induced volume increases are assumed to be restorative in their nature, thereby counteracting age- and/or disorder-dependent volume decline (Firth et al., Reference Firth, Stubbs, Vancampfort, Schuch, Lagopoulos, Rosenbaum and Ward2018; Li et al., Reference Li, Huang, Li, Tao, Zheng and Chen2017). However, as indicated by our data, the group effect of volume increases in the CA and DG is mainly driven by a few subjects in the aerobic exercise group that show particularly pronounced volume increases (Fig. 3b). This suggests that there is a subgroup of patients with schizophrenia that is specifically responsive to aerobic exercise, potentially modulated by lower general polygenic risk for schizophrenia and a higher genetic burden associated with oligodendrocyte precursor cells and radial glia (Papiol et al., Reference Papiol, Popovic, Keeser, Hasan, Schneider-Axmann, Degenhardt and Malchow2017, Reference Papiol, Keeser, Hasan, Schneider-Axmann, Raabe, Degenhardt and Falkai2019). Importantly, more large-scale exercise trials in people with schizophrenia are required to identify stable subgroups of patients that consistently show increased neuroplasticity in the CA and DG after aerobic exercise. To conclude, aerobic exercise potentially mitigates neurodegeneration of one of the most affected HF subfields in people with schizophrenia, at least for a subgroup of patients.
Structural decline of the HF and its subfields is associated with severe cognitive impairments and schizophrenic symptoms (Antoniades et al., Reference Antoniades, Schoeler, Radua, Valli, Allen, Kempton and McGuire2018; Haukvik et al., Reference Haukvik, Tamnes, Söderman and Agartz2018; Ho et al., Reference Ho, Iglesias, Sum, Kuswanto, Sitoh, De Souza and Holt2017; Khalil et al., Reference Khalil, Hollander, Raucher-Chéné, Lepage and Lavigne2022; Nakahara et al., Reference Nakahara, Matsumoto and van Erp2018, Reference Nakahara, Turner, Calhoun, Lim, Mueller, Bustillo and van Erp2020; Pijnenborg et al., Reference Pijnenborg, Larabi, Xu, Hasson-Ohayon, de Vos, Ćurčić-Blake and Van der Meer2020). Persistent cognitive deficits prevent long-term improvements in the patients' social and occupational functioning and thus contribute to an unfavorable disease outcome (Green, Reference Green2016). In this regard, current large-scale evidence appears promising, demonstrating that exercise treatments improve both cognitive functioning (Dauwan et al., Reference Dauwan, Begemann, Heringa and Sommer2016; Fernández-Abascal et al., Reference Fernández-Abascal, Suárez-Pinilla, Cobo-Corrales, Crespo-Facorro and Suárez-Pinilla2021; Korman et al., Reference Korman, Stanton, Vecchio, Chapman, Parker, Martland and Firth2023) and daily life functioning (Dauwan et al., Reference Dauwan, Begemann, Heringa and Sommer2016; Fernández-Abascal et al., Reference Fernández-Abascal, Suárez-Pinilla, Cobo-Corrales, Crespo-Facorro and Suárez-Pinilla2021; Korman et al., Reference Korman, Stanton, Vecchio, Chapman, Parker, Martland and Firth2023) in schizophrenia. Given the aforementioned link between structural decline within the HF and clinical and cognitive symptoms in schizophrenia, we expected individual-specific HF volume changes post exercise intervention to be linked to changes in clinical outcomes. However, we did not observe said effects, thereby contradicting previous studies suggesting volume increases in the HF to be linked to improvements in short-term memory and global functioning (Falkai et al., Reference Falkai, Maurus, Schmitt, Malchow, Schneider-Axmann, Röll and Keeser2021; Pajonk et al., Reference Pajonk, Wobrock, Gruber, Scherk, Berner, Kaizl and Falkai2010). As corresponding evidence in people with schizophrenia is still limited, we cannot yet derive definite conclusions regarding the clinical relevance of exercise-induced structural adaptations of the HF. Because the stable detection of brain-behavior associations in general requires well-powered large-scale studies, those are needed to clarify the clinical implications of aerobic exercise-induced increases in HF subfield volumes in people with schizophrenia. Thereby, future trials should include comprehensive HF-related cognitive test batteries, covering domains such as episodic or visuospatial memory.
Limitations of this study include that the conclusions regarding the HF subfields are preliminary and require further replication in a larger independent sample. As the MRI assessments were not part of the primary endpoint of the ESPRIT C3 study (for details see Maurus et al. (Reference Maurus, Hasan, Schmitt, Roeh, Keeser, Malchow and Falkai2020) and Maurus et al. (Reference Maurus, Roell, Lembeck, Papazova, Greska, Muenz and Falkai2023)) and thus were not financially compensated, only a subgroup of patients agreed to participate. Consequently, future studies should ensure adequate financial compensation for patients undergoing MRI assessments since reservations and fears regarding this method are particularly pronounced in schizophrenia. Furthermore, the FreeSurfer segmentation strategy for the HF subfields based on MRI scans at a spatial isotropic resolution of 1 mm3, as applied at study sites Mannheim and Berlin, has been criticized due to issues regarding the detection of the boundaries between subfields (Wisse et al., Reference Wisse, Chételat, Daugherty, de Flores, la Joie, Mueller and Carr2021). However, we still observe distinct patterns of volume increases within the HF when subdividing it only into head, body, and tail, which is less prone to bias. Moreover, the current data basis was not sufficient to identify subgroups of people with schizophrenia who show pronounced volume increases after aerobic exercise. Lastly, methodological differences across the considered exercise studies in schizophrenia complicate a reasonable quantitative approach to summarize findings regarding the clinical relevance of potential HF volume changes in response to aerobic exercise.
In sum, we conclude that aerobic exercise interventions in people with schizophrenia do not lead to significant increases in the total HF volume on the population level. However, our study provides preliminary evidence that aerobic exercise may lead to increases in specific subfields of the HF, namely CA1, CA2/3, CA4, and DG. Large-scale aerobic exercise studies in people with schizophrenia are required to further investigate said effects of aerobic exercise on HF subfield volumes, to identify subgroups of patients that respond particularly well to aerobic exercise, and to elucidate the clinical implications of volume changes within the HF.
Supplementary material
The supplementary material for this article can be found at https://doi.org/10.1017/S0033291724001867
Data availability statement
The data that support the findings of this study are available on request from the corresponding author, LR. The data are not publicly available because it contains information that could compromise the privacy of research participants. All analysis scripts and documentation sheets will be published on OSF (DOI 10.17605/OSF.IO/TR3NX). A preprint is available under https://doi.org/10.31219/osf.io/y2phs.
Acknowledgements
The work was supported by the German Federal Ministry of Education and Research (BMBF) through the research network on psychiatric diseases ESPRIT (Enhancing Schizophrenia Prevention and Recovery through Innovative Treatments; coordinator, Andreas Meyer-Lindenberg; grant number, 01EE1407E) to AML, PF, AH, and AS. Furthermore, the study was supported by the Else Kröner-Fresenius Foundation with the Research College ‘Translational Psychiatry’ to PF, AS, and IM (Residency/PhD track of the International Max Planck Research School for Translational Psychiatry [IMPRS-TP]). The ‘Studienstiftung des Deutschen Volkes’ provided a PhD scholarship to LR. The study was endorsed by the Federal Ministry of Education and Research (Bundesministerium für Bildung und Forschung [BMBF]) within the initial phase of the German Center for Mental Health (DZPG) (grant: 01EE2303C to AH and 01EE2303A, 01EE2303F to PF, AS).
The authors thank the Clinical Trials Centre Cologne (CTC Cologne) and the Institute for Medical Statistics and Computational Biology of the Medical Faculty of the University of Cologne for developing the database and the secure web-based randomization system and performing data management and monitoring. Furthermore, they express their appreciation to the Clinical Open Research Engine (CORE) at the University Hospital LMU (Munich, Germany) for providing the computational infrastructure to run the CPU-intensive MRI analysis pipelines.
Author contributions
AH, BM, AS, AML, PF: funding, conceptualization, and study design; IM, AR, ES, CET, Chu, BV, WT: recruitment of study participants. IM, LR, IP, SM, ES, CET, BV, CHu, AR: data assessment, supervised by HW; BW, KH, DH, ML, KKV, SMo, DG: conduction of exercise trainings, data assessment. IM, LR, TF: interpretation of data. LR and TF: conduction of statistical analyses supervised by TSA. IM, LR and TF: manuscript writing. DK: supervision in neuroimaging data analysis. BP and LR: Conduction of MRI measurements. SS and BEW: Ensuring of optimal imaging conditions at the Department of Radiology of the LMU Hospital. All authors were involved in revising the article and read and approved the final version of the manuscript.
Competing interests
AS was an honorary speaker for TAD Pharma and Roche and a member of Roche advisory boards. AH is an editor of the German (DGPPN) schizophrenia treatment guidelines and first author of the WFSBP schizophrenia treatment guidelines; he has been on the advisory boards of and has received speaker fees from Janssen-Cilag, Lundbeck, Recordati, Rovi, and Otsuka. PF is a co-editor of the German (DGPPN) schizophrenia treatment guidelines and a co-author of the WFSBP schizophrenia treatment guidelines; he is on the advisory boards and receives speaker fees from Janssen, Lundbeck, Otsuka, Servier, and Richter. AML has received consultant fees from Boehringer Ingelheim, Elsevier, Brainsway, Lundbeck Int. Neuroscience Foundation, Lundbeck A/S, Sumitomo Dainippon Pharma Co., Academic Medical Center of the University of Amsterdam, Synapsis Foundation-Alzheimer Research Switzerland, IBS Center for Synaptic Brain Dysfunction, Blueprint Partnership, University of Cambridge, Dt. Zentrum für Neurodegenerative Erkrankungen, Zürich University, Brain Mind Institute, L.E.K. Consulting, ICARE Schizophrenia, Science Advances, Foundation FondaMental, v. Behring Röntgen Stiftung, The Wolfson Foundation, and Sage Therapeutics; in addition, he has received speaker fees from Lundbeck International Foundation, Paul-Martini-Stiftung, Lilly Deutschland, Atheneum, Fama Public Relations, Institut d'investigacions Biomèdiques August Pi i Sunyer (IDIBAPS), Janssen-Cilag, Hertie Stiftung, Bodelschwingh-Klinik, Pfizer, Atheneum, University of Freiburg, Schizophrenia Academy, Hong Kong Society of Biological Psychiatry, Fama Public Relations, Spanish Society of Psychiatry, Italian Society of Biological Psychiatry, Reunions I Ciencia S.L., and Brain Center Rudolf Magnus UMC Utrecht and was awarded the Prix Roger de Spoelberch grant and the CINP Lilly Neuroscience Clinical Research Award 2016. BEW is a central radiology reader for Bayer Healthcare and her spouse is an employee of Siemens Healthineers. LR, TF, DK, BP, ML, IP, DG, SM, TSA, ES, CT, BV, SM, CH, AR, KKV, BW, SS, KH, BW, WT, HW, DH and IM report no conflicts of interest.
Clinical trials registration
The underlying study of this manuscript was registered in the International Clinical Trials Database, ClinicalTrials.gov (NCT number: NCT03466112, https://clinicaltrials.gov/ct2/show/NCT03466112?term=NCT03466112&draw=2&rank=1) and in the German Clinical Trials Register (DRKS-ID: DRKS00009804).