The increased prevalence of overweight and obesity represents a worldwide public health challenge( Reference Seidell and Halberstadt 1 ) and is the result of a chronic positive energy balance achieved via a long-term surplus of energy intake over energy expenditure( Reference Swinburn, Sacks and Hall 2 ). Although long-term weight loss is achievable with lifestyle modification( Reference Wing and Phelan 3 ), this is notoriously difficult due to the stimulation of physiological adaptations to weight loss that favour weight regain( Reference Greenway 4 ). Considering the challenges of weight loss, it remains essential to better understand the factors that may cause initial weight gain to provide guidance for prevention.
Current evidence suggests that increases in BMI during adulthood( Reference Østbye, Malhotra and Landerman 5 ) are the result of discrete periods of overconsumption, rather than smaller daily energy imbalances( Reference Yanovski, Yanovski and Sovik 6 – Reference Schoeller 9 ). Indeed, repeated episodes of overconsumption during weekends and public holidays may be sufficient to account for long-term weight gain( Reference Yanovski, Yanovski and Sovik 6 , Reference Racette, Weiss and Schechtman 8 ). To date, experimental investigations into the compensatory responses to overfeeding have primarily focused on changes in circulating appetite-related hormone concentrations; with mixed findings likely due to differences in the duration, magnitude and composition of the dietary interventions( Reference Parry, Smith and Corbett 10 – Reference Kolaczynski, Ohannesian and Considine 15 ). Although episodes of overconsumption often occur on only 1 d/week and with a balanced macronutrient profile( Reference Racette, Weiss and Schechtman 8 ), there has been little investigation into the compensatory responses to this model of overconsumption. In addition, an integrated assessment of appetite perceptions and subsequent energy intake alongside mechanistic variables (i.e. appetite-related hormones) is essential to fully understand the magnitude of compensatory responses.
Discrete periods of overconsumption may also impair metabolic control. In this regard, overfeeding with a high-fat diet (≥50 % increase in energy; ≥60 % fat content) has consistently been shown to impair insulin sensitivity in humans( Reference Parry, Smith and Corbett 10 , Reference Brøns, Jensen and Storgaard 14 , Reference Hulston, Churnside and Venables 16 – Reference Lundsgaard, Sjøberg and Høeg 18 ). A recent study by Lundsgaard et al.( Reference Lundsgaard, Sjøberg and Høeg 18 ) has further advanced these findings by demonstrating opposing regulatory effects of high-carbohydrate v. high-fat overfeeding on central and peripheral insulin sensitivity. In this landmark study, 3 d of overfeeding with a high-fat diet (+75 % kJ, 78 % fat) improved hepatic glucoregulation but impaired muscle insulin sensitivity, whereas overfeeding with a high-carbohydrate diet (+75 % kJ, 80 % carbohydrate) induced hepatic insulin resistance but increased insulin sensitivity in the muscle. This evidence suggests that divergent macronutrient intake may mediate the impaired metabolic control observed during overfeeding, and it remains unclear whether short-term overfeeding with a balanced macronutrient profile would provide sufficient stimulus to induce metabolic impairments.
The primary purpose of this study was to determine whether 1d of overfeeding with a balanced macronutrient profile induces compensatory changes in appetite perceptions, appetite-related hormone concentrations and energy intake during a mixed-meal tolerance test (MTT) the next day. The effects of overfeeding on fasted and postprandial markers of metabolic control during the MTT were also assessed. Participants were blinded to the overfeeding intervention to assess the physiological compensatory responses to overfeeding, while minimising the influence of psychological factors and participant bias. These findings contribute to understanding the consequences of common dietary practices and mechanisms of weight control.
Methods
Participants
This study was conducted according to the guidelines laid down in the Declaration of Helsinki, and all procedures were approved by the Ethics Advisory Committee at Leeds Beckett University. Twelve healthy men were recruited for the study and written informed consent was obtained from all participants. Participants were non-smokers, not taking medication, weight stable for at least 6 months before the study and were not dieting. The physical characteristics of participants are as follows: age 22 (sd 2) years, body mass 82·4 (sd 10·2) kg, BMI 26·1 (sd 4·2) kg/m2, waist circumference 86·2 (8·4) cm. This trial is registered at ClinicalTrials.gov (ID: NCT03301948).
Experimental protocol
Overview
Each participant completed a screening session and two 28 h experimental trials separated by 1 week in a single-blind counterbalanced crossover design. The initial screening session involved the collection of anthropometric measures, health screening and confirmation of the acceptability of the foods to be provided during the study.
Standardisation
Participants completed a food diary detailing all foods and drinks consumed in the 24 h before their first experimental trial and repeated this before their second trial. Alcohol, caffeine and strenuous physical activity were not permitted during this period. All trials commenced between 08.00 and 09.00 hours after an overnight fast of at least 10 h, and participants exerted themselves minimally when travelling to the laboratory. Verbal confirmation of adherence to these standardisation procedures was obtained at the beginning of each experimental trial.
Day 1
On day 1 of each trial, participants visited the laboratory to consume breakfast (08.00–09.00 hours), lunch (12.00–13.00 hours) and an evening meal (17.00–18.00 hours). All meals were prepared by the research team, consumed in isolation and consumed at the same time of day in both trials. In one trial these meals provided the calculated energy requirements for each individual (energy balance (EB) trial). In the other trial, the meals were covertly manipulated to increase the energy content by 50 % (overfeed (OF) trial). Participants were required to consume all of the foodstuffs provided at each meal and this was confirmed by a member of the research team. The magnitude of overfeeding (+50 % kJ) was selected to align with previous research that investigated the appetite-related and metabolic responses over more prolonged periods of 5–7 d( Reference Parry, Smith and Corbett 10 , Reference Brøns, Jensen and Storgaard 14 , Reference Hulston, Churnside and Venables 16 ). The impairments in metabolic control observed during these studies suggest that overfeeding by 50 % provides a significant metabolic challenge, while we also deemed this to be a realistic target to enable covert dietary manipulation and participant blinding to the intervention.
Participants were permitted to leave the laboratory between meals but were required to remain on the university campus to minimise physical activity. Each participant was fitted with a SenseWear Pro3 Armband (BodyMedia) upon arrival at the laboratory on day 1 of each trial, and these were worn until arrival at the laboratory for day 2 of the respective trial. This was intended to discourage physical activity and was used to check that the energy expenditure of participants was matched between trials( Reference Rousset, Fardet and Lacomme 19 ). Participants returned home after consumption of the evening meal and came back to the laboratory the next morning having fasted overnight. Verbal confirmation of adherence to the overnight fast was obtained at the beginning of the second day of each trial for all participants.
Day 2
On day 2 of each trial, participants arrived at the laboratory between 08.00 and 09.00 hours to complete an MTT. Upon arrival, participants rested in a semi-supine position for 5 min before a cannula (Introcan Safety; B Braun) was inserted into an antecubital vein. A baseline blood sample and appetite visual analogue scale were collected approximately 10 min after the insertion of the cannula before the participant commenced the MTT.
The MTT involved consumption of white bread (toasted), butter, strawberry jam and orange juice. The energy content of the meal was relative to each participant’s estimated energy requirements by providing the same energy content as the porridge breakfast meal on day 1 of the EB trial (2748 (sd 198) kJ). This approach was used to standardise energy intake for differences in body mass/composition between participants( Reference Travers, Motta and Betts 20 ). The macronutrient composition of the MTT meal included 60 % carbohydrate, 32 % fat and 8 % protein, to increase ecological validity and provide a more ‘physiological response’ compared with glucose- or fat-only challenges( Reference Travers, Motta and Betts 20 , Reference Selimoglu, Duran and Kiyici 21 ).
Blood samples and appetite perceptions were collected every 30 min during the 180 min postprandial period, while participants rested within the laboratory (sitting, reading or listening to music). Upon completion of the postprandial period, participants were provided with an ad libitum pasta meal to assess energy intake. Water intake was measured during the first trial for each participant and replicated during the second trial (505 (sd 288) ml).
Overfeeding intervention
The meals consumed during day 1 of EB provided the estimated daily energy needs for each participant, which were calculated using the Mifflin–St Jeor equation( Reference Mifflin, Jeor and Hill 22 ) and a physical activity factor of 1·4 to represent the sedentary nature of experimental testing days. This approach to estimate energy requirements is consistent with previous literature( Reference Parry, Smith and Corbett 10 , Reference Brøns, Jensen and Storgaard 14 , Reference Parry, Woods and Hodson 17 , Reference Votruba, Kirchner and Tschöp 23 ) and was deemed preferable to designing the intervention based on self-report food diaries due to the established concerns over the accuracy of self-report measures( Reference Dhurandhar, Schoeller and Brown 24 ). The energy content of all meals comprised 50 % carbohydrate, 35 % fat and 15 % protein, which is in accordance with the UK dietary guidelines( 25 ). During day 1 of OF, the raw weight of foodstuffs included in the meals was increased by 50 %.
The manipulation of food weights was covertly achieved by adjusting the water content of meals, cooking duration, and through the addition of thickening agents to the meals provided during EB. To avoid any a priori awareness of the participants to the overfeeding intervention, this experiment was described as involving ‘nutrient manipulation’ during recruitment and throughout the study. The blinding of participants to the true aims of the study was deemed important to assess the physiological compensatory responses to overfeeding, while minimising the influence of psychological factors and participant bias. All participants completed a blinding assessment upon completion of the experiment and the true nature of the intervention was discussed. The meals provided were as follows: porridge (breakfast), pasta dish and soup (lunch) and rice dish (evening meal). A milkshake was provided alongside each meal which contained 837 kJ on EB and 1255 kJ on OF for all participants. The remaining energy intake was divided evenly across the three meals. The meal ingredients, preparation methods and quantities for an example participant are provided in Table 1.
Table 1 Ingredients, preparation methods and example quantities for the meals provided during day 1 of the energy balance (EB) and overfeed (OF) trials

* Note that a small proportion of maltodextrin was replaced with tomato ketchup in the EB trial when preparing the soup dish. This was deemed necessary to ensure blinding of the meals and this did not alter the macronutrient composition of the meal. The energy content of all meals comprised 50 % carbohydrate, 35 % fat and 15 % protein. Differences in preparation methods are denoted as the EB trial and OF trial to describe the specific procedures for each trial.
Appetite, palatability and energy intake assessment
Appetite perceptions (hunger, satisfaction, fullness and prospective food consumption) were assessed using 100 mm visual analogue scales with descriptors anchored at each end describing the extremes (e.g. ‘I am not hungry at all’/‘I have never been more hungry’)( Reference Flint, Raben and Blundell 26 ). These measures were collected before and after each meal on day 1, and in the fasted state and every 30 min during the MTT. A composite appetite score was calculated for each time point as the mean value of the four appetite perceptions after inverting the values for satisfaction and fullness( Reference Stubbs, Hughes and Johnstone 27 ). Palatability ratings (visual appeal, smell, taste, aftertaste and pleasantness) were obtained for all meals immediately after consumption( Reference Flint, Raben and Blundell 26 ). A composite palatability score was calculated as the mean value of the palatability subscales.
Upon completion of the 180 min postprandial period, an ad libitum meal was provided, consisting of penne pasta, cheddar cheese, tomato sauce and olive oil in accordance with previous research( Reference Deighton, Frampton and Gonzalez 28 ). Pasta was cooked in a microwave for 13 min in unsalted water at 700 W before being mixed with the remaining ingredients and re-heated for 2 min at 700 W. The macronutrient content of the meal was 50 % carbohydrate, 35 % fat and 15 % protein( 25 ). Participants consumed the ad libitum meal in isolation to prevent any social influences affecting food intake. Participants were provided with a bowl of the pasta meal, which was replaced by an investigator before the participant had emptied it and with minimal interaction. No time limit was set for eating, and participants were instructed to eat until ‘comfortably full’. Food intake was determined as the weighted difference in food before and after eating.
Blood sampling and biochemical analyses
At each time point, venous blood samples were collected into one 5-ml and one 9-ml pre-cooled EDTA monovette (Sarstedt). The 9 ml monovettes were used for the determination of plasma concentrations of glucose, insulin, TAG, NEFA, total glucagon-like peptide-1 (GLP-1) and total peptide YY (PYY). The 5-ml monovettes were used for the determination of plasma acylated ghrelin concentrations and were pre-treated on the morning of testing, to prevent the degradation of acylated ghrelin, with a 50-µl solution of PBS, p-hydroxymercuribenzoic acid and sodium hydroxide. Both monovettes were spun at 1500 g for 10 min at 4°C. Plasma from the 9 ml tube was immediately aliquoted into 2-ml Eppendorf tubes before storage at –20°C, whereas 1 ml of plasma from the 5-ml monovette was mixed with 100 µl of 1 m hydrochloric acid( Reference Hosoda, Doi and Nagaya 29 ) before storage at –20°C.
Plasma glucose, TAG and NEFA concentrations were analysed from all blood samples photometrically with reagents from Instrumentation Laboratory and Wako Chemicals, respectively. Insulin was analysed from all blood samples using a commercially available enzyme immunoassay (IBL). Plasma acylated ghrelin, total GLP-1 and total PYY concentrations were analysed using commercially available enzyme immunoassays (SPI BIO; EMD Millipore). Due to the plate layout of the acylated ghrelin, total GLP-1 and total PYY ELISA, these analytes were measured at all time points except for 150 min. To eliminate inter-assay variation, samples from each participant were analysed in the same run. The within-batch CV were as follows: acylated ghrelin 3·3 %, total GLP-1 3·0 %, total PYY 5·1 %, glucose 3·2 %, insulin 4·3 %, TAG 3·7 % and NEFA 2·8 %.
Statistical analyses
Data were analysed using IBM SPSS version 24 for Windows. Sphericity of the data was assessed using Mauchly’s test of sphericity, with any violation corrected using the Greenhouse–Geisser method. Fasted measures and ad libitum energy intake were compared using paired t tests. The dynamic appetite, hormonal and metabolic responses to the MTT were compared using a two-way (trial×time) repeated-measures ANOVA. Significant interaction effects were explored using unadjusted paired t tests. Statistical significance was accepted at P<0·05. Effect sizes are presented as Cohen’s d and interpreted as <0·2 trivial, ≥0·2 small, ≥0·6 moderate, ≥1·2 large, ≥2 very large and ≥4 extremely large.
Results in text and tables are presented as means and standard deviations. Graphical representations of results are presented as means and standard errors of the mean. Appetite, hormonal and metabolic responses to the MTT are presented as line graphs to display changes over time. Time-averaged AUC values were calculated for these variables using the trapezoidal method, which are displayed in figures alongside the individual participant responses in the online Supplementary material to allow further examination of the findings.
Based on previous data from our laboratory( Reference Deighton, Frampton and Gonzalez 28 ), a sample size of twelve participants provided >80 % power to detect a 1250 kJ compensatory increase in energy intake at the ad libitum meal. This calculation was performed using G*Power with an α value of 5 %( Reference Faul, Erdfelder and Lang 30 ).
Results
Day 1
Energy intake was 10 755 (sd 593) and 16 132 (sd 889) kJ on the EB and OF trials, respectively. Estimated energy expenditure was 12 423 (sd 1340) and 12 450 (sd 1679) kJ on day 1 of the EB and OF trials, respectively (P=0·917).
Appetite was not different between trials during day 1 (online Supplementary Fig. S1; main effect of trial P=0·212, trial×time interaction P=0·783). Palatability of the meals provided on day 1 was not significantly different between trials, except for the milkshake consumed as part of the evening meal which was significantly more palatable on OF compared with EB (P=0·020; online Supplementary Table S1). Water intake was not different between trials (EB 2003 (sd 848) ml; OF 1876 (sd 842) ml; P=0·674).
Day 2
Fasted measures of appetite, plasma appetite-related hormone, glucose, insulin and TAG concentrations did not differ between trials (all P>0·188). Fasted NEFA concentrations were significantly higher in EB than in OF (P=0·005; Table 2).
Table 2 Fasted appetite perceptions, plasma appetite-related hormone concentrations and metabolite concentrations after a day of supervised feeding in accordance with estimated energy requirements (energy balance trial) or 50 % overfeeding (overfeed trial) (Mean values and standard deviations, n 12)
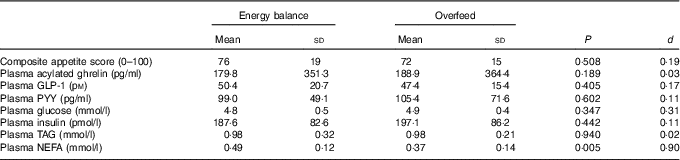
GLP-1, glucagon-like peptide-1; PYY, peptide YY.
Appetite changed over time (P<0·0005) in response to the MTT but without any differences in the magnitude or time course of these responses between trials (main effect of trial P=0·720, trial×time interaction P=0·706; Fig. 1(a)). Ad libitum energy intake upon completion of the MTT was not different between trials (P=0·781; d=0·05; Fig. 1(b)). Palatability of the MTT meal was not different between trials (EB 72 (sd 9); OF 72 (sd 10); P=0·885; d=0·03). Palatability of the ad libitum pasta meal was not different between trials (EB 69 (sd 12); OF 70 (sd 11); P=0·656; d=0·09).

Fig. 1 Composite appetite score (a) and ad libitum energy intake (b) during a mixed-meal tolerance test after a day of supervised feeding in accordance with estimated energy requirements (energy balance trial; ; solid line) or 50 % overfeeding (overfeed trial;
; dashed line). Values are means (n 12), with standard errors of the mean represented by vertical bars. Lines in (b) represent individual participants.
Plasma concentrations of appetite-related hormones changed over time (all P≤0·021) in response to the MTT but without any difference in the magnitude or time course of these responses between trials (main effect of trial, all P≥0·336; trial×time interaction, all P≥0·364; Fig. 2).
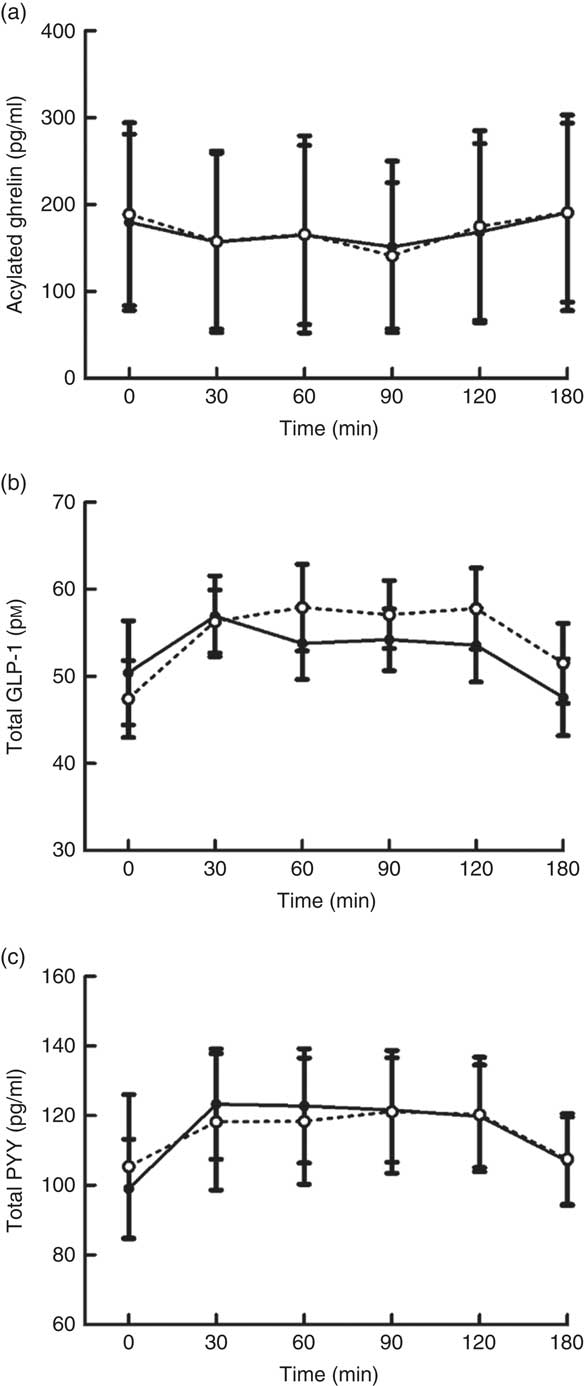
Fig. 2 Plasma acylated ghrelin (a), total glucagon-like peptide-1 (GLP-1) (b) and total peptide YY (PYY) (c) concentrations during a mixed-meal tolerance test after a day of supervised feeding in accordance with estimated energy requirements (energy balance trial; ; solid line) or 50 % overfeeding (overfeed trial;
; dashed line). Values are means (n 12), with standard errors of the mean represented by vertical bars.
Plasma concentrations of glucose, insulin, TAG and NEFA changed over time in response to the MTT (all P<0·0005) (Fig. 3). There were no differences in the magnitude or time course of these responses between trials for glucose and insulin concentrations (main effect of trial, both P≥0·929; trial×time interaction, both P≥0·716). Alternatively, plasma TAG concentrations diverged between trials as the duration of the postprandial period increased, resulting in a significant trial×time interaction effect (P=0·009) but no main effect of trial (P=0·219). A significant trial×time interaction effect was also detected for plasma NEFA (P=0·001) due to higher concentrations in EB than in OF in the fasted baseline state. In accordance with other plasma metabolites, there was no main effect of trial for plasma NEFA concentrations (P=0·113).

Fig. 3 Plasma glucose (a), insulin (b), TAG (c) and NEFA (d) concentrations during a mixed-meal tolerance test after a day of supervised feeding in accordance with estimated energy requirements (energy balance trial; ; solid line) or 50 % overfeeding (overfeed trial;
; dashed line). Values are means (n 12), with standard errors of the mean represented by vertical bars. * Significant difference between trials determined via two-way ANOVA and post hoc paired t test analysis of a significant trial×time interaction (P<0·05).
AUC data and individual participant responses during the MTT are presented in the online Supplementary materials. There were no significant differences between trials in the AUC values for appetite, appetite-related hormone concentrations or plasma metabolites (all P≥0·175, d≤0·45).
Blinding assessment
In response to the exit questionnaire, eight out of the twelve participants stated that they noticed a difference between meals during day 1 of EB and OF. Of these eight participants, only one successfully guessed that the meals differed in energy content. The remaining participants guessed that the aim of the intervention was to manipulate the sweetness of meals (two participants), the sweetness and thickness of meals (two participants), the protein and fat content (one participant), the milk and water content (one participant) and one participant declined to guess the nature of the intervention.
Discussion
In the present study, we provide novel data demonstrating that 1d of overfeeding (+50 % kJ) with a balanced macronutrient profile does not elicit any compensatory changes in appetite perceptions, selected appetite-related hormone concentrations and ad libitum energy intake during an MTT the next day. In addition, although glucose and insulin responses were unaffected, 1 d of overfeeding elicited reduced plasma NEFA concentrations after an overnight fast and elevations in postprandial TAG concentrations during the MTT. These findings highlight the consequences of acute overfeeding as a stimulus for the accumulation of a positive energy balance and increased levels of TAG as a key CVD risk marker.
In addition to the absence of counter-regulatory appetite responses during the MTT, appetite perceptions also did not differ between trials during the day of energy intake manipulation (i.e. energy balance v. overfeeding). This observation contrasts with the established robust increases in appetite that occur during energy restriction( Reference King, Wasse and Ewens 31 – Reference Thivel, Finlayson and Miguet 34 ), even with modest deficits of <800 kJ per meal( Reference Deighton, Batterham and Stensel 35 ). Such divergent responses help to explain the ease of habitual overconsumption( Reference Yanovski, Yanovski and Sovik 6 , Reference Racette, Weiss and Schechtman 8 ) and the contrasting difficulties of sustained dieting( Reference Ikeda, Lyons and Schwartzman 36 ), especially in modern societies where energy-dense, highly palatable foods are abundant and easily accessible( Reference Swinburn, Sacks and Hall 2 ). The gradual accumulation of a positive EB through repeated discrete episodes of overfeeding seems plausible, considering the absence of any compensatory appetite and energy intake responses during the MTT the next day. Indeed, mean values for both of these variables differed by <2 % between trials, which further highlight the limited physiological response to defend against excess energy intake( Reference Schwartz, Woods and Seeley 37 ). These findings emphasise the need for conscious monitoring and adjustment of food intake around such episodes of overconsumption to prevent the gradual accumulation of a positive energy balance.
To understand the effects of overfeeding on physiological regulators of appetite control, circulating concentrations of selected appetite-related gastrointestinal hormones were measured during the MTT. In accordance with the findings discussed above, the overfeeding intervention did not stimulate any changes in fasted or postprandial concentrations of the orexigenic( Reference Karra and Batterham 38 ) hormone acylated ghrelin or the anorectic( Reference Karra and Batterham 38 , Reference Murphy and Bloom 39 ) hormones PYY and GLP-1. These peptides represent key markers of impaired appetite regulation in obese individuals, as depressed concentrations of PYY and GLP-1 and reduced ghrelin responses to feeding are thought to be implicated in reduced satiety and hyperphagia( Reference le Roux, Patterson and Vincent 40 – Reference Hussein, Abushady and Refaat 43 ). The findings from the present study support previous evidence that 3–7 d of overfeeding does not induce any changes in circulating ghrelin and GLP-1 concentrations( Reference Parry, Smith and Corbett 10 , Reference Hagobian, Sharoff and Braun 11 , Reference Brøns, Jensen and Storgaard 14 ). Importantly, this also suggests that the assessments made after these longer interventions did not mask any immediate compensatory changes in hormone concentrations. Alternatively, Brøns et al. ( Reference Brøns, Jensen and Storgaard 14 ) reported a borderline significant increase in fasted PYY concentrations after 5 d of high-fat overfeeding (+50 % kJ, 60 % fat). Thus, it seems likely that more prolonged or high-fat overfeeding is required to induce compensatory changes in PYY concentrations, which accord with evidence that PYY release is more potently stimulated by fat than carbohydrate consumption( Reference Essah, Levy and Sistrun 44 ). Ultimately, the findings from the present study demonstrate that circulating concentrations of key appetite-related hormones do not change to provide a defence against an acute episode of overconsumption. The lack of change in these hormones also suggests that obesity-related dysfunctions in the appetite-regulating endocrine system do not occur acutely and are most likely stimulated by weight gain.
The focus of the present study on appetite, appetite-related hormones and energy intake responses precluded the additional measurement of energy expenditure during the MTT. Although this represents an important outcome to complete the energy balance equation, previous evidence suggests that mass-independent increases in resting energy expenditure and diet-induced thermogenesis do not occur during chronic or short-term overfeeding( Reference Dirlewanger, Di Vetta and Guenat 13 , Reference Müller, Enderle and Bosy-Westphal 45 , Reference Siervo, Fruhbeck and Dixon 46 ). Differences in energy expenditure observed in response to energy intake manipulation appear to be the result of changes in light-intensity activity( Reference Betts, Richardson and Chowdhury 47 ), which was not permitted during the MTT in the present study. Light-intensity activities may also have been limited during the day of dietary manipulation based on the guidance to minimise physical activity levels. Nevertheless, it must be acknowledged that these activities often occur subconsciously( Reference Betts, Richardson and Chowdhury 47 ) and therefore that the lack of difference between trials during the day of dietary manipulation may represent a genuine absence in compensatory movement responses. Future investigations into the free-living responses to acute overfeeding would be beneficial to further investigate these effects.
The standardisation of physical activity levels during the first day of each trial was essential for the accurate assessment of appetite-related and metabolic responses to the intervention during the MTT. However, although energy expenditure was matched between trials, estimates from SenseWear armbands were approximately 1650 kJ higher than the predictive equations used to calculate the feeding interventions. The extent to which these values deviated from ‘true’ energy expenditures is unclear without the inclusion of a criterion measure in the present study, but a recently published meta-analysis suggests that SenseWear Pro3 armbands significantly overestimate energy expenditure during sedentary activities as performed during day 1 of each trial( Reference O’Driscoll, Turicchi and Beaulieu 48 ). Regardless of these discrepancies in energy expenditure estimates, the use of predictive equations to prescribe energy intake is consistent with previous overfeeding interventions( Reference Parry, Smith and Corbett 10 , Reference Brøns, Jensen and Storgaard 14 , Reference Parry, Woods and Hodson 17 , Reference Votruba, Kirchner and Tschöp 23 ) and is supported by the prescribed meals inducing an appropriate degree of satiation during the trials.
One day of overfeeding with a balanced macronutrient composition did not induce any changes in fasted or postprandial glucose and insulin concentrations during the MTT. This contrasts with the previously reported impairments in glycaemic control after high-fat overfeeding interventions (+50 % kJ, ≥60 % fat) lasting for 5–7 d( Reference Parry, Smith and Corbett 10 , Reference Brøns, Jensen and Storgaard 14 , Reference Hulston, Churnside and Venables 16 ), and more extreme high-fat overfeeding for a single day (+78 % kJ, 68 % fat)( Reference Parry, Woods and Hodson 17 ). Although the shorter duration of moderate overfeeding in the present study may have reduced the stimulus for metabolic disturbance, these findings are also likely to reflect the impact of overfeeding with a balanced macronutrient composition. In this regard, recent evidence demonstrated that whole-body insulin sensitivity decreased after 3 d of high-fat overfeeding but increased after 3 d of high-carbohydrate overfeeding( Reference Lundsgaard, Sjøberg and Høeg 18 ). These differences appeared to be primarily mediated by changes in substrate oxidation at the muscle, which highlights the importance of divergent macronutrient intake for stimulating short-term changes in glycaemic control.
Although markers of glycaemic control did not differ between trials, the overfeeding intervention induced significant elevations in postprandial TAG concentrations during the MTT. This is an important outcome, considering that postprandial lipaemia is an established independent risk factor for CVD( Reference Bansal, Buring and Rifai 49 , Reference Nordestgaard, Benn and Schnohr 50 ). Furthermore, the divergence in TAG concentrations between trials towards the end of the 180- min postprandial period suggests that this effect is likely to continue in response to subsequent feeding( Reference Arjunan, Deighton and Bishop 51 ). The mechanisms underlying the observed elevations in postprandial lipaemia are unclear but are likely to relate to the increased consumption of absolute amounts of carbohydrate and fat. In this regard, increased insulin release during high-carbohydrate overfeeding has been suggested to exaggerate postprandial lipaemia by increasing VLDL-TAG production and/or decreasing hydrolysis of circulating TAG due to reduced muscle lipoprotein lipase activity( Reference Lundsgaard, Sjøberg and Høeg 18 ). This potential role of elevated insulin concentrations during the day of dietary manipulation is also supported by the observed lower fasted concentrations of plasma NEFA after the day of overfeeding. Alternatively, increases observed after high-fat overfeeding have been suggested to be the result of increased storage and subsequent release of TAG within the enterocyte pool( Reference Parry, Woods and Hodson 17 ). While further research is required to elucidate the mechanisms of this effect, these findings demonstrate that even short-term episodes of overfeeding with habitual macronutrient distributions can exert negative effects on metabolic control.
The present study has provided novel insights into the effects of an ecologically relevant episode of energy overconsumption on appetite-related and metabolic responses. Nevertheless, some limitations must be acknowledged. First, the blinding of participants to the overfeeding intervention may have prevented the occurrence of psychologically driven compensatory responses and subsequent reductions in energy intake during the MTT. Although this is feasible, the aim of this study was to isolate the physiological responses to excess energy consumption, which required the removal of potential psychological influences. The lack of counter-regulatory physiological changes in response to the overfeeding intervention highlights the ease with which energy overconsumption can occur, especially considering the increased prevalence of eating away from the home( Reference Adams, Goffe and Brown 52 ) and limited awareness of required portion sizes more generally( Reference Roberto and Khandpur 53 ). Second, although this is the first study to investigate the effects of 1d of mixed-macronutrient overfeeding, these findings must be extended to investigate the consequences of repeated bouts of such overconsumption. Evidence from chronic overfeeding interventions suggests that additional compensatory responses are unlikely to occur with repeated exposure( Reference Siervo, Fruhbeck and Dixon 46 ), but future investigations remain essential to confirm the role of repeated discrete episodes of overconsumption in the accumulation of a positive energy balance. Third, the consequences of dietary practices for potential weight gain and metabolic impairments in presently healthy young men were investigated. However, although the prevention of weight gain has been highlighted as a major public health priority( Reference Lawlor and Chaturvedi 54 ), these findings may not generalise to women or obese participants. Future investigations in these populations would be beneficial, especially in obese participants to further understand the effects of dietary manipulation on energy balance and weight control.
In conclusion, this study demonstrated that 1d of overfeeding with a balanced macronutrient profile does not elicit any compensatory changes in appetite perceptions, selected appetite-related hormone concentrations and ad libitum energy intake during an MTT the next day. Appetite perceptions during the day of overfeeding were also unaffected. Taken together, this absence of compensatory appetite-related responses to an ecologically relevant overfeeding protocol supports the concept that repeated discrete episodes of overconsumption may promote weight gain. Increases in postprandial TAG concentrations during the day after overfeeding further emphasise the risks of acute dietary excess. These findings highlight the need for dietary awareness and conscious compensatory behavioural adjustments should episodes of overconsumption occur.
Acknowledgements
The authors thank all of the volunteers for their participation in this study. The authors also thank James Taylor and Emma Kellett for their help with data collection.
This research received no specific grant from any funding agency, commercial or not-for-profit sectors.
K. D. and A. H. conceived the study; K. D., A. J. K., J. M., O. M. S. and A. H. designed the study; all authors contributed to data collection, analysis and interpretation; K. D. drafted the manuscript; all authors revised and approved the final version of the manuscript.
The authors declare that there are no conflicts of interest.
Supplementary material
For supplementary material/s referred to in this article, please visit https://doi.org/10.1017/S0007114519000205