4.1 Introduction
In an article entitled ‘The Geologist as Historian’, one of the twentieth century’s foremost British geologists, H. H. Read (1889–1970), characterized geology as ‘earth-history’ (Reference Read1952: 409). Read’s point was that geology has a lot in common with human history, dealing as it does with the reconstruction of particular events that occurred in the past, albeit on a very different timescale (and minus a role for human agency). Read noted that in geology, as with its counterpart in the humanities, ‘no event has ever been exactly repeated’ (Reference Read1952: 411). A possible point of contention emerges, however, when this analogy is extended to the mode of explanation in geology. It is uncontroversial to state that explanations in history have a narrative form, but suggesting that ultimately all explanations of events in time are narrative in structure (as asserted by Reference Richards, Nitecki and NiteckiRichards 1992: 22–23) may well cause unease among many geologists who do not wish their science to be associated with a term which may seem vague and unscientific, or which is suggestive of ‘mere’ storytelling.Footnote 1
In this chapter, I will seek to emphasize and uphold the narrative nature of geology, a property which may not be widely understood or appreciated, even by its own practitioners, but which has a logic and rigour of its own (Reference FrodemanFrodeman 1995: 966). Unlike those in human history, narratives in geology are always strictly bounded by what is possible according to the general laws of physics and chemistry; they are also tightly constrained by what is geologically plausible, although there is always scope for daring or ‘outrageous’ hypotheses (Reference DavisDavis 1926), which are open to review and testing by the geological community.
Without being too prescriptive, the essence of a narrative statement can be usefully thought of as specifying two time-separated events, so that the prior event is understood to have given rise to, and thereby to explain, the later event;Footnote 2 it is typically expressed in the past tense (Reference DantoDanto 1962: 146). More complex narratives, involving multiple events and processes which have interconnected causal relationships, are built on this simple formulation. A narrative explanation therefore specifies the causal connections in a temporal sequence of events or processes. It is important to note that a narrative in a historical science such as geology is neither a chronicle (a chronological listing of disconnected events),Footnote 3 nor is it a merely descriptive exercise. It has been argued that a defining characteristic of historical sciences such as geology is that they rely on narrative sentences for understanding (Reference GriesemerGriesemer 1996: 66). As will become apparent in the course of this chapter, however, narratives in the geological literature are not always explicitly narrative.
4.1.1 Narrative Reasoning
While the most obvious function of narratives in geology is to communicate explanations or interpretations, narrative is also fundamental to geological reasoning. Mary Morgan (Reference Morgan2017) describes the mental process of ‘narrative ordering’, in which what may initially appear to be disconnected events are able to be woven together into a coherent whole, thereby imparting meaning to them. Narrative ordering may take place in a variety of settings, such as in the act of individual reflection, in the course of writing or sketching, or in the process of discussion with others. The idea expounded by Morgan refers to common practice in the social sciences, but it describes well the route a geologist might follow in order to make sense of a collection of puzzling observations. Interpreted events in geology also derive their meaning from being part of an overall story; that is, they only make sense when they contribute to and form a component of an overarching narrative. The theory of plate tectonics, involving the separation and collision of continents on a timescale of hundreds of millions of years, supplies the most obvious ‘big picture’ narrative in modern geology.Footnote 4 Robert Frodeman refers to this property in which ‘details are made sense of in terms of the overall structure of a story’ as ‘narrative logic’ (Reference Frodeman1995: 963). However, the term ‘narrative logic’ could usefully be extended to include the criteria employed in narrative ordering. Hence, narrative logic can be understood as having both an internal dimension, through the coherent ordering of related events, and an external dimension, via the relationship of those events to overarching ideas.
Counterfactual reasoning can serve as another powerful device in the geologist’s mental toolbox, although it may not be explicit in many written accounts. Deliberately changing elements of a geological narrative or filling in gaps in the data to see what difference it makes to the overall picture can reveal flaws or strengths in a particular argument. Hence, counterfactual reasoning can help to expose narratives that do not make geological sense (i.e., do not display narrative logic), clearing the way for ones that do.Footnote 5
4.1.2 The Impermanence of Geological Narratives
The evidence available to the geologist seeking to piece together ‘the fantastic drama of the earth’s crust’ (Reference ReadRead 1952: 409) consists of tracesFootnote 6 of events which ceased long ago but which have been left behind in rocks, fossils and landscapes. These traces, however, are prone to concealment, degradation, even complete destruction over the vast expanse of geological time: weathering and erosion act on rocks at the surface, while at depth, profound changes may be wrought by pressure, heat or geochemical reactions. It is also true that while some traces are particularly susceptible to elimination, certain geological processes leave no traces at all (e.g., Reference Tipper, Smith, Bailey, Burgess and FraserTipper 2015). As Kleinhans, Buskes and de Regt (Reference Kleinhans, Buskes and de Regt2005: 290) conclude, a result of this incompleteness is that ‘theories and hypotheses [in geology] usually are underdetermined by the available evidence’. Consequently, the word interpretation tends be used more frequently than explanation in geology.Footnote 7 The two terms are almost synonymous, although interpretation suggests something more provisional and hypothetical.Footnote 8 In the context in which it is generally used by geologists, an interpretation can be understood as a response to the question ‘What caused these traces?’ (see, for example, Reference Faye, Su, rez, Dorato and RédeiFaye 2010: 108–111).
In a discipline in which previously concealed or overlooked traces have a habit of eventually turning up, new ideas, analytic techniques and interpretative methods are constantly being developed. Old theories are regularly replaced, so narratives tend to come with a degree of implied uncertainty and provisionality and are often not assumed to be the last word. When the evidence changes or when theories are superseded it is not unusual for geological narratives to be modified or even to be completely rewritten, although intellectual inertia might retard the process of revision.
4.1.3 Central Subjects in Historical Narratives
The construction of a historical narrative requires the identification of a central subject (Reference HullHull 1975). Its purpose is to provide the coherence necessary for intelligibility (Reference FrodemanFrodeman 1995: 965–966) and to form ‘the main strand around which the historical narrative is woven’, a key requirement being continuity in space and time (Reference HullHull 1975: 262). In the rest of this chapter, the role of central subject will be occupied by the outcrop of a particular stratum of ancient rock situated in Scotland. The changing historiography of this layer will serve to illustrate both the narrative nature of geology and the impermanent character of many geological narratives.Footnote 9 The focus of the case study is on how geologists communicate through papers and articles within the community of fellow practitioners.
4.2 The Case of the Stac Fada Member
The cliffs along the Assynt coastline of Sutherland, north-west Scotland, are formed of some of the oldest sedimentary rocks in the British Isles. The reddish-brown outcrop has been known informally as the Torridonian since the late nineteenth century when it was recognized to be of Pre-Cambrian age.Footnote 10 Despite their great antiquity, the rocks are acknowledged to be remarkably well preserved. They were originally assumed to be unfossiliferous, although eukaryotic microfossils are now known to be present (Reference Brasier, Culwick, Battison, Callow, Brasier, Brasier, McIlroy and McLoughlinBrasier et al. 2017).
Writing in 1897, J. G. Goodchild of the Geological Survey of Great Britain drew a direct analogy between the ancient sediments that formed the Torridonian rocks and the modern sands currently being deposited in ephemeral rivers and lakes in the Sinai Desert, on the basis of their remarkably similar form and composition:
To my mind one of the most striking and significant illustrations of the principle upon which geologists interpret the records of the Past, by the study of the Present, is to be found in the Torridonian areas of the North-West of Scotland. If we review the conditions obtaining in the Sinaitic Peninsular […] we find going on there to-day almost the exact counterpart of what must have taken place in Pre-Cambrian times in Sutherland and Ross.
The Geological Survey studied and mapped the rocks of north-west Scotland in a major campaign that ran from 1883 to 1897. The results were published in a substantial Memoir several years later (Reference Peach, Horne, Gunn and CloughPeach et al. 1907), in which descriptions of the Torridonian rocks occupied one of five subsections.
Following something of a hiatus during the early twentieth century, research on the Torridonian resumed in the 1960s. A research group was set up in the Geology Department of Reading University focused exclusively on furthering knowledge and understanding of the Torridonian (Reference StewartStewart 2002: 3). Initial reconnaissance work revealed the rocks to be ‘unexpectedly complex’ (Reference Gracie and StewartGracie and Stewart 1967: 182), and it took several years of careful fieldwork to unravel the stratigraphic relationships. Among the unexpected complexities encountered by the Reading group was a particular layer which was noted to be generally between 10 m and 30 m thick. In the redefinition of Torridonian stratigraphy undertaken by the Reading group, this layer was named the Stac Fada Member (Reference StewartStewart 2002: 5).Footnote 11 This rock unit, the outcrop of which stretches across more than 50 km of coastline (Figure 4.1), was regarded as unremarkable by the nineteenth-century Survey geologists,Footnote 12 but was noted by the Reading researchers to differ in a number of significant respects from the layers immediately above and below (Reference StewartStewart 2002: 9–11).
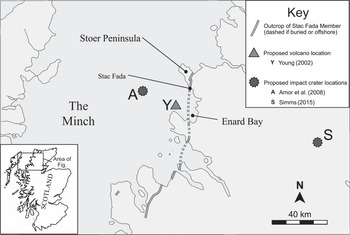
Figure 4.1 Location map of the Stac Fada outcrop
D. E. Lawson’s (1972) study of the Stac Fada Member described some of its constituents as angular shards of pumice, green particles of devitrified glassFootnote 13 and accretionary lapilliFootnote 14 (as shown in Figure 4.2). These were all interpreted as products of a nearby volcano. As with Goodchild’s Sinai Desert comparison, this interpretation was made by analogy with present-day processes. Accordingly, the Stac Fada Member was interpreted either as a pyroclastic flow – an airborne surge of fluidized ash and other fragments derived from a violent eruptive event (Lawson 1972) – or as more of a surface-bound volcanic mudflow (Reference StewartStewart 2002).
The volcanic interpretation of the Stac Fada Member initially seemed to fit the field observations well and it held sway for several decades. The absence of a volcanic vent in the surrounding landscape was not seen as problematic, given the long-term effects of erosion and burial. Based on the distance that present-day accretionary lapilli are known to travel through the air in an eruption, the volcanic vent was suggested to have lain a short distance offshore (Reference YoungYoung 2002: 7–8; point Y in Figure 4.1). However, it was apparent that there were some aspects that did not add up. For example, the lack of evidence for additional contemporaneous flows was regarded as ‘curious’ by Lawson: he explained that one ‘would not really expect volcanic activity to cease after a single eruption’ (Lawson 1972: 346, 360). Furthermore, several ‘thorny problems’ (Reference StewartStewart 2002: 10–11) were identified in the geological evidence. For instance, indicators of transport directions in the sediments showed that there had been an ‘abrupt change’ in the slope of the land from east to west ‘immediately prior to deposition of the Stac Fada Member’ (Reference StewartStewart 2002: 10–11). This was difficult to explain in terms of the volcanic hypothesis given that the outcrops along the coast were estimated to have been located too far from the putative volcano to have been affected by any associated land movements. Although several modes of volcanic emplacement had been proposed, it was concluded that none of them satisfactorily explained all of the field observations (Reference StewartStewart 2002: 10–11), and Stewart noted that, in 2002, the volcanic hypothesis remained ‘controversial’ (Reference StewartStewart 2002: 65). Despite these unresolved issues, the phenomenon of Stac Fada volcanism was incorporated, albeit with a degree of incongruity, into the body of literature on Scottish geology. For example, a major synthesis of the tectonic and magmatic evolution of Scotland included a short section on Stac Fada volcanism, in which the authors referred to it as ‘enigmatic’ (Reference Macdonald and FettesMacdonald and Fettes 2006: 232–233).
4.2.1 Old Evidence, New Discovery
Since 2004, the Torridonian outcrop has formed part of the North West Highlands Geopark and has become a popular destination for geology undergraduate field trips. On an Oxford University field course in 2006, postgraduate geologist Ken Amor was serving as an assistant to the teaching staff. Amor had recently returned from Ries in Bavaria where he had been studying the rocks around one of Europe’s few recognized meteorite craters. His attention was drawn to the distinctive green fragments of devitrified glass in the Stac Fada outcrop which had been highlighted by the Reading geologists. Although they were consistent with the prevailing volcanic interpretation, he had seen remarkably similar crystals close to the Ries crater, where they were interpreted to have been formed by the melting of the surface rocks in the impact event. There were no known instances of a major meteorite strike in the British Isles, but the green particles aroused Amor’s curiosity. A microscopic examination of thin sections of the rock in question would provide a test of Amor’s hunch, and on returning to Oxford he discovered that his department already held some thin sections that had been made from rocks collected on previous field trips.Footnote 15 However, he knew that the chances of finding anything new were not promising. ‘How many countless eyes of undergraduates had looked at these very same thin sections over several decades and not spotted anything unusual’?Footnote 16
What Amor hoped to see down his microscope were crystals of shocked quartz – a form of silica which bears the marks of the instantaneous application of stresses that are far higher than can occur in terrestrial processes, and which is regarded as an unequivocal indicator of a so-called ‘hypervelocity impact’. Against his expectations, Amor did find grains of shocked quartz in the Stac Fada thin sections (Figure 4.3), and the implications of his discovery soon dawned on him. He later reflected: ‘I remember thinking at the time that at that moment I was the only person […] to realise that the UK had been struck by an asteroid […] I didn’t tell my supervisor for two days because I wanted to hold on to that discovery moment for a little longer’.Footnote 17
Amor’s moment of insight has led to the Stac Fada Member being reinterpreted as an ejecta blanket – the material violently thrown out of the crater formed by the impact of a massive extraterrestrial body, overturning the previous interpretation of the layer as the product of a volcanic eruption and resolving many of the inconsistencies surrounding that explanation. For example, the sudden change in the slope of the land now made sense as a consequence of the impact. Amor published his findings in a co-authored paper two years after the breakthrough discovery (Reference Amor, Hesselbo, Porcelli, Thackrey and ParnellAmor et al. 2008). In addition to shocked quartz, the paper presented further evidence of an impact origin including the shocked form of another mineral (biotite) and key geochemical indicators such as anomalous chromium isotope values and elevated abundances of platinum group metals such as iridium. Subsequently, grains of shocked zircon were discovered in the Stac Fada deposit (Reference Reddy, Johnson, Fischer, Rickard and TaylorReddy et al. 2015), further confirming the impact ejecta interpretation. The melange of angular fragments and partially melted material in the Stac Fada Member is now routinely referred to as a suevite, the term for such a deposit created by an extraterrestrial impact.Footnote 18 The particles of devitrified glass and accretionary lapilli which had been assumed by the Reading geologists to be uniquely diagnostic of volcanic eruptions were evidently also capable of being formed in major meteorite impacts. This had been recognized from evidence at the Ries impact site for some time (Reference Kölbl-EbertKölbl-Ebert 2015: 275), a fact of which Amor would have been aware. According to radiometric dating of the Stac Fada Member, the impact occurred approximately 1.2 billion years ago, placing it in the Mesoproterozoic Era (Reference Parnell, Mark, Fallick, Boyce and ThackreyParnell et al. 2011).Footnote 19
There is no sign of the impact crater from which the Stac Fada Member was ejected. As with the now discarded volcanic interpretation and the absence of a volcano, this is not surprising given the burial or removal by erosion of much of the Torridonian outcrop in the last billion or more years. Different lines of reasoning by different geologists, but based essentially on the same evidence, have resulted in two different locations being posited for the crater (A and S in Figure 4.1). Amor et al. (Reference Amor, Hesselbo, Porcelli, Thackrey and Parnell2008; Reference Amor, Hesselbo, Porcelli and Price2019) suggest that a point in the Minch Basin 15–20 km to the north north-west of Enard Bay, an area that would have been dry land at the time of impact, is the most likely location, while an alternative hypothesis by Simms has the crater deeply buried onshore about 50 km to the east of the outcrops along the coast (Reference SimmsSimms 2015). Further data-gathering work in the form of expensive geophysical surveys or borehole drilling would be required to confirm or deny both proposals. However, Simms’s location has been criticized as being inconsistent with the overarching narrative of the plate tectonic history of northern Scotland (Reference Butler and AlsopButler and Alsop 2019: 443), and, in an example of counterfactual reasoning, Amor et al. Reference Amor, Hesselbo, Porcelli and Price(2019) argue against Simms’s location by pointing out that there would have been a topographic obstruction blocking the path of the ejecta blanket if it came from the east (Reference Amor, Hesselbo, Porcelli and PriceAmor et al. 2019: 842).
Three years after the publication of the discovery paper (Reference Amor, Hesselbo, Porcelli, Thackrey and ParnellAmor et al. 2008), volcanologists Michael Branney and Richard Brown addressed the question of exactly how the Stac Fada Member might have been emplaced. Nothing remotely approaching the scale of the meteorite impact from which the deposit is believed to have originated has ever been observed in recorded history,Footnote 20 and terrestrial impact ejecta blankets are commonly not well preserved, limiting the availability of possible analogues. The example at Ries is a notable exception, however, and Branney and Brown (Reference Branney and Brown2011) highlight parallels between the Stac Fada deposit and the Ries suevite. The absence of a crater, however, means that there is no way of investigating the relationship between it and the ejecta.
The authors note that while there are ‘important differences’ between the ejecta from impacts and those from volcanoes – for example, the presence of shocked minerals and distinctive geochemistry – there are also ‘striking similarities’ (Reference Branney and BrownBranney and Brown 2011: 287–288). The distinctive components of the Stac Fada deposit, including the presence of devitrified glass and accretionary lapilli, as well as the order in which they were deposited, resemble those ejected from large explosive volcanic eruptions. These similarities have led them to deduce that emplacement mechanisms comparable to pyroclastic processes associated with volcanoes were at work and they have coined the analogous term, impactoclastic (Reference Branney and BrownBranney and Brown 2011: 276) to describe their model, which details how the Stac Fada impact ejecta blanket could have been deposited (as we can see in Figure 4.4).
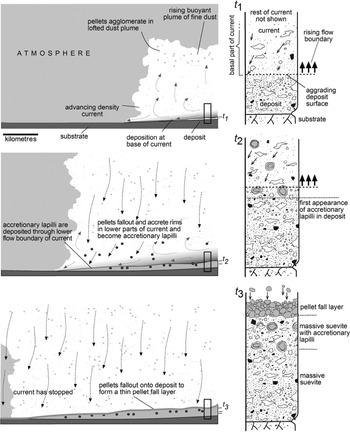
Figure 4.4 The impactoclastic emplacement of the Stac Fada ejecta blanket
For the benefit of the non-geologist reader, three pairs of images show the situation at successive points in time immediately following the meteorite impact. Each pair consists of a panel showing a cross-section through the dust plume thrown up by the impact (on the left) and a column representing the vertical accumulation of different types of debris deposited by the plume by that time (on the right). The time sequence, t1 to t3, runs from top to bottom. In the plume cross-sections, the crater lies out of frame to the right and the plume moves from right to left through the time sequence. Along the base of each of these cross-sections is the layer of debris deposited from the plume. This increases in thickness with time as marked by the ticks labelled t1, t2 and t3 at the bottom right of each cross-section. The location of each column of debris is marked by a rectangular outline in the bottom right of each corresponding plume cross-section. An understanding of how the overall diagram is put together, along with some technical (geological) knowledge, enables it to be read as a self-contained narrative.
4.3 Tracing the Narratives
4.3.1 Geological Narratives, Explicit and Implicit
At first glance, the writings of the geologists who have worked on the Torridonian outcrop since the late nineteenth century seem to contain few obvious instances of narrative statements of the kind discussed in the introduction, i.e., those which causally connect time-separated events. Notable instances include parts of Goodchild’s (Reference Goodchild1897) paper on the desert environment in which the Torridonian sediments were interpreted to have been deposited. For example, in the following passage he gives a straightforwardly narrative account of the weathering processes by which the Torridonian sands and shales would have been formed from the breakdown of pre-existing rocks based on observations made in the present-day Sinai environment:
[R]ain fell only occasionally, or practically never, and only on those occasions when thunderstorms happened to burst over the regions in question. At other times the arid conditions gave rise to great diurnal ranges of temperature. The rocks in consequence were heated soon after mid-day far above the temperature usual in more humid climates, and by early morning, owing to rapid radiation, had cooled down to the opposite extreme. In a rock composed of constituents of diverse mineral character differential expansion takes place, owing to their different coefficients of expansion. The felspars in the rocks […] gave way under the strain set up by extreme expansion and contraction, due to the rapid changes of temperature. The ferro-magnesian minerals […] in like manner splintered into fragments so small that they were easily blown away as dust by the wind. Little by little the rocks crumbled down, and of their wasted portions the larger part slid down the valley side as talus, to be eventually distributed and spread out in the bottoms of the wadies by the action of the occasional torrents arising during storms; the remainder, chiefly in the form of dust, was blown far and wide by the winds.
Another noteworthy narrative passage occurs as part of the impactoclastic model of Reference Branney and BrownBranney and Brown (2011). The following excerpt accompanies an explanatory ‘cartoon’ (the main part of which is reproduced here as Figure 4.4):
Time frames (t1–3) [depict] the generation and evolution of ash aggregates within an impactoclastic current. Turbulent entrainment of atmospheric air along the upper mixing zone of the current results in expansion and lofting, generating a buoyant dust plume. Within this [plume], ash pellets start to form (t1). Once these pellets become too large to be supported by turbulence in the lofted plume, they drop to lower parts of the current, dry out, accrete concentric ash rims (t2), and become deposited as fully formed accretionary lapilli, along with suevite from the base of the current. After cessation of the current, ash pellets fall out from the drifting buoyant dust plume and deposit directly on the top of the suevite (t3). The absence of accretionary lapilli in the lower parts of deposits is due to the time lag between the onset of deposition from the base of the current and the formation of pellets, their descent into the current, their growth within the current into accretionary lapilli, and their subsequent deposition.
The use of images to complement or clarify textual narratives is common in geology, and this text is designed to be read alongside the diagram. With appropriate geological knowledge and understanding of the context, however, the diagram itself could be read independently as a narrative, tracing as it does the temporal and spatial sequence of deposition caused by the transit of the waning dust plume.Footnote 21
In each of the passages by Goodchild (Reference Goodchild1897) and Branney and Brown (Reference Branney and Brown2011), the narrative structure of temporally arranged and causally connected sequences of events and processes is evident. Words and phrases denoting events and processes include ‘differential expansion’, ‘splintered into fragments’, ‘turbulent entrainment’ and ‘deposition’; while causal connections are signalled by ‘gave rise to’, ‘in consequence’, ‘results in’ and ‘generating’, among others. Both passages also illustrate the role of physical laws in constraining geological narratives. For example, Goodchild refers to the differential expansion of minerals when heated, and the effects of turbulence, expansion and gravity in a hot dust plume form part of the account of Reference Branney and BrownBranney and Brown.
While these passages constitute examples of explicit geological narratives, in most of the other literature on the Torridonian and the Stac Fada Member considered here, narratives are generally more covert and implicit. Consider the following two sentences taken from A. D. Stewart’s volume on the Torridonian:
Upward movement of the rift floor on the east arrested the growth of the alluvial wedge and formed a depression that trapped the Stac Fada mudflow and the lake sediments constituting the Poll a’ Mhuilt Member that follows.
The palaeosol grades up through sandy claystone with corestones of gneiss (locally cut by the unconformity), into dusky red claystone.
The first sentence is manifestly narrative, linking as it does a chain of events causally connected by the ‘Upward movement of the rift floor’. On the other hand, the second sentence appears at first to be a straightforward description. However, a geologist would also read this as a sequence of events. For example, the verb, ‘grades up’, while primarily a spatial expression, also serves as a proxy for temporal change due to the link between vertical succession and geological time in stratigraphy.Footnote 22 The change from palaeosol (fossil soil) to sandy claystone to red claystone indicates a series of environmental changes from humid to arid or semi-arid conditions; the corestones and the unconformity are also both the result of geological processes that have operated through time.Footnote 23 The sentence is therefore narrative when read in a certain way by a certain person (i.e., a geologist). The significance of phrases such as ‘grading up’ can be appreciated through the concept of scripts as described by David Herman in the field of cognitive narratology. Scripts allow the reader to ‘build up complex (semantic) representations of stories on the basis of few textual or linguistic cues’ (Reference HermanHerman 1997: 1051).Footnote 24 The ability to recognize cues entailed by geological terms derives from a geologist’s specific training and experience, rather than from a general familiarity with routine life situations as in Herman’s examples. Most of the narrative work in the geological papers referred to in this chapter is implicit and is performed by sentences which are nominally descriptive but which contain multiple geological cues. Unlike most (explicitly) narrative sentences, these tend to be written in the present tense.
Explicitly narrative sentences are particularly rare in some papers. Where they do occur, they tend to be restricted to the abstracts or the conclusion sections. For example, apart from one narrative sentence in the abstract of Reference Amor, Hesselbo, Porcelli, Thackrey and ParnellAmor et al. (2008), the entire paper is composed of dry geo-scientific prose in the form of (nominally) descriptive sentences laden with various cues which contain the implicit, underlying narrative of geological processes.Footnote 25 This form of presentation seems at odds with the cataclysmic drama of the discovery being reported. When he was interviewed for the BBC Radio 4 Today programme in 2019, Amor gave a very different style of account, which imagined the scene about 100 km from the point of impact:
The first thing you’d see would be this enormous fireball extending up from where the asteroid hit the surface. That would generate thermal radiation enough to ignite wood and paper. Shortly after that you would feel a seismic wave equivalent to a magnitude 8 earthquake. About 2.4 minutes later you would get the first debris – dust, hot bits of molten rock raining down on you. At 100 kilometres away it would be enough to cover about 6 inches depth. And then the final thing would be the 450 mph wind that would suddenly hit you as the air blast comes in.Footnote 26
The tone of Amor’s contribution was suggestive of the process of narrative ordering that he and his colleagues might have gone through when working out the causal sequence of events before the narrative got turned into the relatively bland text of a scientific paper.
4.3.2 Narrative Logic and Narratives Rewritten
The narrative sentences and statements discussed in the previous section may be thought of as narrative units or fragments,Footnote 27 each of which contributes to an extended narrative history of the Stac Fada Member. In little more than a century, the Stac Fada Member has been the subject of three of these narrative histories, each constituting a radical departure from its predecessor. The sequence might be summarized as follows:
1. The layer that came to be named the Stac Fada Member is an unremarkable part of the Torridonian outcrop, the sandstones and shales of which were deposited by rivers and lakes in a semi-arid environment (Geological Survey: late nineteenth century).
2. The Stac Fada Member was formed by a violent pyroclastic surge or volcanic mudflow derived from a nearby eruption (Reading Group: 1960s–2000s).
3. The Stac Fada Member represents the material violently ejected from the crater formed by a major meteorite impact (Oxford Group: since 2006).
The nineteenth-century geologists appear not to have recognized the distinctive nature or the significance of the Stac Fada Member, or perhaps they overlooked it altogether. This is not particularly surprising given the limited extent of the Stac Fada outcrop (Figure 4.1) and the extensive area and difficult terrain covered by the Survey geologists in mapping the north-west Highlands. The first change of narrative introduced the idea that the deposit was formed by volcanic activity, a familiar geological phenomenon. The second change, however, invoked a fundamentally different and novel causal explanation. The impact narrative was able to challenge the volcanic consensus largely on the basis of a piece of microscopic evidence which had been missed by all previous investigators. It also resolved some of the logical problems that had beset the volcanic narrative, such as the apparent occurrence of a solitary eruption and the evidence for the tilting of the land surface.
4.3.3 The Acceptance of the Impact Narrative: The Back Story
The volcanic interpretation of the Stac Fada Member was first expounded in the 1960s (Reference LawsonLawson 1965), a time when the possibility of extraterrestrial explanations was not even being considered by most geologists. Shocked quartz was not found in the deposit until 2006 because nobody had previously looked for it, a deficit that can be explained at least partly by the fact that the potential significance of shock metamorphism and its distinctive petrology only began to be reported in the 1960s. The tendency for evidence to be overlooked when it does not form part of the observer’s conceptual framework recalls an incident recorded by Charles Darwin (1809–82). Writing about a time before he knew of the theory of glaciation, Darwin recounted his experience of spending ‘many hours’ examining the rocks in a valley in North Wales ‘with extreme care’ while completely missing the abundant evidence for the glacial origin of the valley itself. He commented with hindsight that ‘these phenomena are so conspicuous that […] a house burnt down by fire did not tell its story more plainly than did this valley’ (Reference DarwinDarwin 1887: 57–58).Footnote 28 In the case of the Stac Fada Member, it should be noted that the failure to consider an impact origin is also mitigated to a significant extent by the absence of a crater, the interpretation of an impact ejecta blanket in the absence of a source crater being extremely rare. Even the Ries crater, which is well exposed, was interpreted as a volcanic edifice until shocked quartz was discovered there in the early 1960s.
The acceptance of the impact narrative should also be understood in the wider context of a disagreement that played out in the latter half of the twentieth century between the great majority of geologists who were only prepared to consider terrestrial explanations and those who were open to entertaining the possibility that solid bodies falling from space might act as geological agents (Reference Marvin, Craig and HullMarvin 1999). The dispute came to a head in 1980 with the publication of evidence that the impact of a major asteroid had caused the well-known mass extinction at the end of the Cretaceous, about 66 million years ago (Reference Alvarez, Alvarez, Asaro and MichelAlvarez et al. 1980). The initial response was ‘total uproar’ (Reference Marvin, Craig and HullMarvin 1999: 105–109) and, by 1984, geologist Eugene Shoemaker (1928–97), who had worked on the Ries crater and had campaigned largely unsuccessfully for the acceptance of the evidence for extraterrestrial impacts since the early 1960s (Reference Marvin, Craig and HullMarvin 1999), felt obliged to lament the closed minds of many of his colleagues:
[M]ost geologists just don’t like the idea of stones the size of hills or small mountains falling out of the sky. While they may concede, at an intellectual level, that such things might happen, at a visceral level, it still seems vaguely outrageous. In part this is due, I think, to an overdose of Lyellian uniformitarianism in their geological education, and in part, to their failure to view the Earth constantly as a member of the Solar system.
Shoemaker’s comment on ‘Lyellian uniformitarianism’ referred to the principle promoted by Charles Lyell (1797–1875), which, in its methodological sense, holds that ‘the observable present is a crucial resource in understanding the past’ (Reference OreskesOreskes 2013: 595), and is thus indispensable to geological practice as exemplified by Goodchild’s (Reference Goodchild1897) Torridonian-Sinai analogy (see section 4.3.1). Lyell, however, also implied that only explanations which invoked ‘gradual change by processes intrinsic to the Earth’ (Reference Marvin, Craig and HullMarvin 1999: 105) were admissible in geology, and he would not have been able to countenance the ‘outrageous’ possibility of a catastrophic meteorite impact – ‘a process of random violence, originating outside the Earth’ (Reference Marvin, Craig and HullMarvin 1999: 112) and capable of wreaking instantaneous devastation. The implication was that many of Shoemaker’s geological contemporaries were still in thrall to Lyell on this matter. By the early 1990s, however, the Alvarez hypothesis had been greatly reinforced when the probable crater was located in Mexico (Reference Marvin, Craig and HullMarvin 1999: 109–112), and with the subsequent accumulation of evidence for many other crater-forming events in the geological record, the role of meteorite impacts in geology and in palaeontologyFootnote 29 had become part of the mainstream by the early twenty-first century (e.g., Reference FrenchFrench 2004).
Finally, should we consider the impact interpretation of the Stac Fada Member to be the last word, the final narrative? The history of science exclaims an emphatic ‘No!’ There seems to be no reason why more new evidence of as yet unknown significance might not turn up, or why new theories might not lead in a different direction. The consensus for the impact interpretation is quite strong at present, with most geologists with an interest in the region or in impact deposits coming down in favour. However, there are a few dissenting voices. Osinski et al. (Reference Osinski, Preston, Ferrière and Prave2011) have cautioned that the Stac Fada deposit is ‘not what it seems’; they point to several inconsistencies which they believe cast serious doubt on some of the details of the impact interpretation of Reference Amor, Hesselbo, Porcelli, Thackrey and ParnellAmor et al. (2008). The Geological Excursion Guide to the North-West Highlands of Scotland, published by the Edinburgh Geological Society (Reference Goodenough and KrabbendamGoodenough and Krabbendam 2011), is also not convinced that the Stac Fada Member is an impact ejecta blanket.
Recently, evidence has emerged that shocked quartz can also be formed by lightning strikes, which threatens to remove its status as an unequivocal indicator of meteorite impacts (‘Impact Geologists, Beware!’ – Reference MeloshMelosh 2017). This potentially replicates the situation that affected accretionary lapilli when they were relegated from their status as unambiguous evidence of volcanic eruptions upon their discovery at impact sites. It should be pointed out, however, that the impact interpretation is also supported by additional evidence such as anomalous geochemical markers which are not consistent with potential alternatives such as lightning strikes. Nevertheless, it remains to be seen whether factors which may emerge in the future will cause a further rewrite of the Stac Fada narrative.
4.4 Conclusions
Robert Richards (Reference Richards, Nitecki and Nitecki1992: 23) is surely correct to claim that ‘all explanations of events in time are ultimately narrative in character’ (and it is evident that H. H. Read did not extend his analogy of geology with human history far enough explicitly to acknowledge this fact). Accordingly, the case of the interpretation and re-interpretation of the stratigraphic unit known as the Stac Fada Member demonstrates that geology is an inescapably narrative science that follows rigorous standards of internal and external narrative logic and is constrained by physical and chemical laws and by the norms of geology.
Narrative statements in the geological literature on the Stac Fada Member, and on the Torridonian of which it is a part, are commonly presented in the form of what appear at first to be descriptions of observations. However, these contain cues which the geologist automatically picks up, and by virtue of her training and experience she makes a range of default assumptions which are translated into causally connected temporal sequences. This phenomenon is particularly applicable to geology because of the relationship between space and time in the ways in which rocks accumulate, as well as in the clues to past environments which certain types of rock embody. More ‘traditional’ narrative passages that explicitly express the causal relationships between time-separated events also occur but are less common. Textual narratives may be accompanied by images that aim to add clarity but which may often be read as narratives themselves. Beyond the field of communication, a lot of narrative activity in geology is unseen, as it takes place in the minds and conversations of geologists as they try to make sense of observations that may initially be perplexing.
Geology is also an interpretive science, and narratives that attempt to answer the question, ‘What caused these traces?’, are inevitably accompanied by some degree of uncertainty. This is illustrated by the fact that key pieces of evidence, such as the presence of shocked quartz, may be overlooked for a variety of reasons, and by the observation that different conclusions may be drawn from the same field evidence – as illustrated by the disagreement over the most likely location of the missing crater. Geological narratives are therefore prone to be rewritten when new evidence or new ideas emerge. Whether the Stac Fada narrative will be rewritten again remains to be seen. In its latest version, the Stac Fada narrative provides a contribution to the body of knowledge relating to the susceptibility of the Earth to periodic meteorite impacts, a phenomenon which is now recognized as posing an existential threat to humanity.Footnote 30