Highlights
-
• Implementation of ultra-low-field MRI in a remote area is feasible, demonstrating clinical and economic benefits.
-
• The use of ultra-low-field MRI improves access to neuroimaging and reduces diagnostic delays for both urgent and nonurgent neurological presentations.
-
• Ultra-low-field MRI is a valuable adjunct to conventional MRI and CT.
Introduction
MRI is an integral part of diagnostics for many neurological conditions; however, access to MRI in Canada continues to pose a challenge. In Canada, there are only 10 MRI machines per million of the population, Reference Chao, Sinclair, Morrison, Hafizi and Pyke1 which is considerably lower than the median of 16.5 scanners per million of all countries within the Organisation for Economic Cooperation and Development. 2
The disparity in access to imaging in Canada is particularly pronounced in rural and remote areas. For example, Weeneebayko General Hospital (WGH), which serves over 12,000 people in 6 communities along the James Bay Coast in Northern Ontario, has no access to conventional MRI onsite. Patients requiring MRI for either nonurgent or urgent indications are required to travel 314 km to Timmins or 841 km to Kingston, Ontario, by charter flight.
Conventional high-field MRI scanners (1.5 or 3 T) tend to be located in highly populated urban centers, largely due to their cost and infrastructure requirements. A 1.5 T MRI scanner costs around $2 million CAD and requires approximately $200,000 CAD in yearly service contract fees. Conventional MRI scanners are large, weighing over 5 tons, and require dedicated rooms with reinforced flooring and radio frequency shielding. Reference Manso Jimeno, Vaughan and Geethanath3 As conventional MRI scanners utilize superconducting magnets, they require cryogenic cooling and high-power infrastructure. Reference Cosmus and Parizh4
An additional limitation to the use of conventional MRI is the staffing requirements. The healthcare industry is facing a severe shortage of qualified MRI technologists, a challenge that is particularly pronounced in remote and underserved regions in Canada and elsewhere. The highly specialized nature of conventional MRI technology, combined with the extensive training and certification required, means that there is already a limited pool of qualified professionals nationwide. Recruiting these skilled technologists to rural or isolated areas further compounds the issue, as these locations often struggle to compete with the compensation, amenities, career development opportunities and personal/family choices available in urban centers. Furthermore, many remote communities experience a high rate of staff turnover, limiting the availability of qualified personnel to operate MRI and other radiology equipment.
Recent advances in ultra-low-field (less than 0.1 T) MRI have aimed to address the infrastructure costs and staffing limitations of high-field MRI and offer a potential solution to improve imaging access. The first such commercially available system is the Swoop portable MRI, an ultra-low-field (0.064 T) scanner for brain imaging (Hyperfine, Guilford, Connecticut, USA). Since the Swoop portable MRI received Health Canada approval in December 2021, it has started to be integrated into clinical practice in Canada, primarily for brain imaging in the intensive care unit (ICU). Reference Islam, Lin and Bharatha5 There are currently four units operating for clinical use in Canada, most of which are at large tertiary care adult and pediatric hospitals. The device is 140 cm tall by 86 cm wide (slightly larger than a portable ultrasound machine), weighs 630 kg, plugs into a standard 120 V wall outlet and does not have any additional power or infrastructure requirements.
The current cost of a unit is approximately $650,000 CAD with approximately $62,000 CAD in annual service contract fees. We previously reported the significant financial benefits of portable MRI, when implemented in a remote setting in Canada (Moose Factory, Ontario). Cost savings were $854,841 based on 50 patients receiving portable MRI over 1 year, and 5-year budget impact analysis showed nearly $8 million dollars saved. Reference DesRoche, Johnson and Hore13 The cost savings were primarily due to a reduction in patient transport expenses, with contribution from near zero infrastructure expenses.
From a staffing perspective, the training requirements to operate a portable MRI are considerably less compared to a conventional MRI. Typical training takes 1–2 hours for a healthcare worker to be able to safely operate the machine as the scanning procedure is notably automated, with sequence acquisitions seamlessly integrated into the imaging protocol. However, given that portable MRI is a new technology, it was not until recently encompassed within the authorized scope of practice for Canadian X-ray technologists or nurses. As of 2021, the Ontario Association of Medical Radiation Technologists has established that any duly qualified X-ray technologist is eligible to operate a portable MRI device, provided they have received a verbal or written directive from a physician. Reference Islam, Lin and Bharatha5 Consequently, this allows for greater staffing availability for the operation of portable MRIs.
Several studies have demonstrated the safety, feasibility and diagnostic utility of portable MRI in the both adult and neonatal ICU settings. Reference Sheth, Mazurek and Yuen6–Reference Sien, Robinson and Hu9 Ultra-low-field MRI has also been utilized to improve access to imaging in several low-resource settings. Reference Altaf, Baqai and Urooj10–Reference Ogbole, Adeyomoye, Badu-Peprah, Mensah and Nzeh12 However, to date, the clinical utility of portable MRI in a remote Canadian hospital that otherwise does not have onsite access to conventional MRI has not been explored.
This study reports the results of implementing portable MRI at WGH in Moose Factory, Ontario, over a 20-month period. Fifty patients underwent portable MRI, of which the interim results for 25 patients and economic cost analysis of implementation were reported previously. Reference DesRoche, Johnson and Hore13 The primary objective of this study was to evaluate the feasibility, clinical and operational impacts of utilizing a portable MRI in a remote setting in Canada to help guide future implementation in similar locales.
Methods
This single-site prospective cohort study was approved by the local institutional ethics review board and conducted in accordance with the OCAP principles for the governance of Indigenous Health Data. Reference Schnarch14 Health Canada Investigational Testing Authorization – Class II was received prior to study initiation.
Patients were recruited from those presenting to the emergency department, inpatient unit, and outpatient clinics at Weeneebayko General Hospital, Moose Factory, ON. Inclusion criteria comprised patients aged 18 years or older, presenting with any indication for neuroimaging, provided their treating team had ordered non-contrast head imaging (CT or conventional MRI), or if neuroimaging was indicated necessary by the treating physician. Potential candidates were screened by a research coordinator for study eligibility, and those with body size exceeding the portable MRI scanners 30 cm vertical opening, active implants such as a pacemaker, implanted defibrillator, deep brain stimulator, vagus nerve stimulator, cochlear implant or programable shunt or MRI incompatible surgical hardware were excluded. Informed consent was obtained in either English or Cree prior to study inclusion.
Fifty patients received a portable MRI, of which 25 were previously reported in the study interim results. Reference DesRoche, Johnson and Hore13 A portable low-field (0.064 T) MRI scanner (Swoop Portable MR Imaging System, Hyperfine, Guilford, Connecticut, USA) was delivered and installed at Weeneebayko General Hospital, Moose Factory, Ontario. The portable MRI installation and details of the study setup have been described previously. Reference DesRoche, Johnson and Hore13
Non-contrast MRI head images were acquired without the use of sedation. Standardized sequences consisting of axial T1-weighted fast spin echo, T2-weighted fast spin echo, T2-weighted fluid-attenuated inversion recovery (FLAIR) and diffusion-weighted imaging with apparent diffusion coefficient sequences were acquired following the manufacturer’s protocol. All indications for imaging were recorded. These were retrospectively compared to the volumes of MRI head examinations ordered the year prior to determining if the availability of portable MRI influenced referral patterns. Images were reported by fellowship-trained neuroradiologists at Kingston Health Sciences Center who provide 24-hour on-call coverage. A standardized dictation template for the study was created, which included sections for notes on image quality and the need for additional conventional MRI. Urgent findings, such as acute stroke, hemorrhage, hydrocephalus or herniation, were communicated directly to the referring physician over the phone.
Radiology reports were retrospectively analyzed for image findings and quality notes. Turnaround time was calculated from the time of image acquisition to the time the radiologist’s report was finalized. Descriptive statistics of clinical data are presented as frequencies and percentages for categorical variables and as medians with interquartile ranges (IQRs) for continuous variables.
Results
Health Canada Investigational Testing Authorization - Class II approval was granted for 50 patients to receive a portable MRI as part of this study. Over the study duration of 20 months (November 2021–June 2023), all patients who presented to WGH with an indication for neuroimaging meeting the study inclusion criteria were eligible to receive a portable MRI. Three patients declined to participate in the study. One patient was excluded as body habitus exceeded the portable MRI scanner’s 30 cm vertical opening, and one patient was excluded due to the presence of an active implant.
Fifty patients (median age, 53 years [IQR, 41–69 years]; 52% women) underwent portable MRI over the duration of 20 months. All patients who received a portable MRI are included in the study analysis. Specific demographic characteristics of these patients are not included in alignment with the OCAP principles, that of ownership, control, access and possession governing the use of indigenous health data. Reference Schnarch14 The indications for the 50 portable MRIs ordered are listed in Table 1 with acute stroke (n = 10) being the most common, representing 20% of the portable MRI indications.
Table 1. Clinical indications for ordering portable MRI during the study period (November 2021–June 2023)
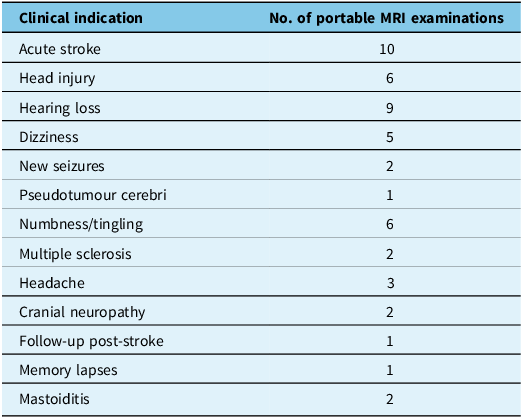
The implementation of portable MRI did not change referral volumes. In the first 12 months of the study, 35 portable MRIs were performed, compared to 38 conventional MRI heads during a 12-month period the year prior to portable MRI availability onsite.
The median time from scan completion to the time reported by a neuroradiologist for nonurgent indications was 10.6 hours (IQR, 2–27.5 hours). Urgent findings were immediately communicated to the referring physician over the phone prior to report finalization. Comments on suboptimal image quality were made by the reporting neuroradiologist for 12 (24%) of the portable MRI examinations. These included motion artifact (3), zipper artifact (4) (Figure 1), incomplete visualization due to patient position (2) and other mentions of artifacts on at least one of the image sequences (3). Of the examinations where a comment on image quality was made, it was recommended that two patients receive follow-up imaging with either conventional MRI or CT for further evaluation. The remaining 10 were deemed of sufficient diagnostic quality despite the presence of an artifact.
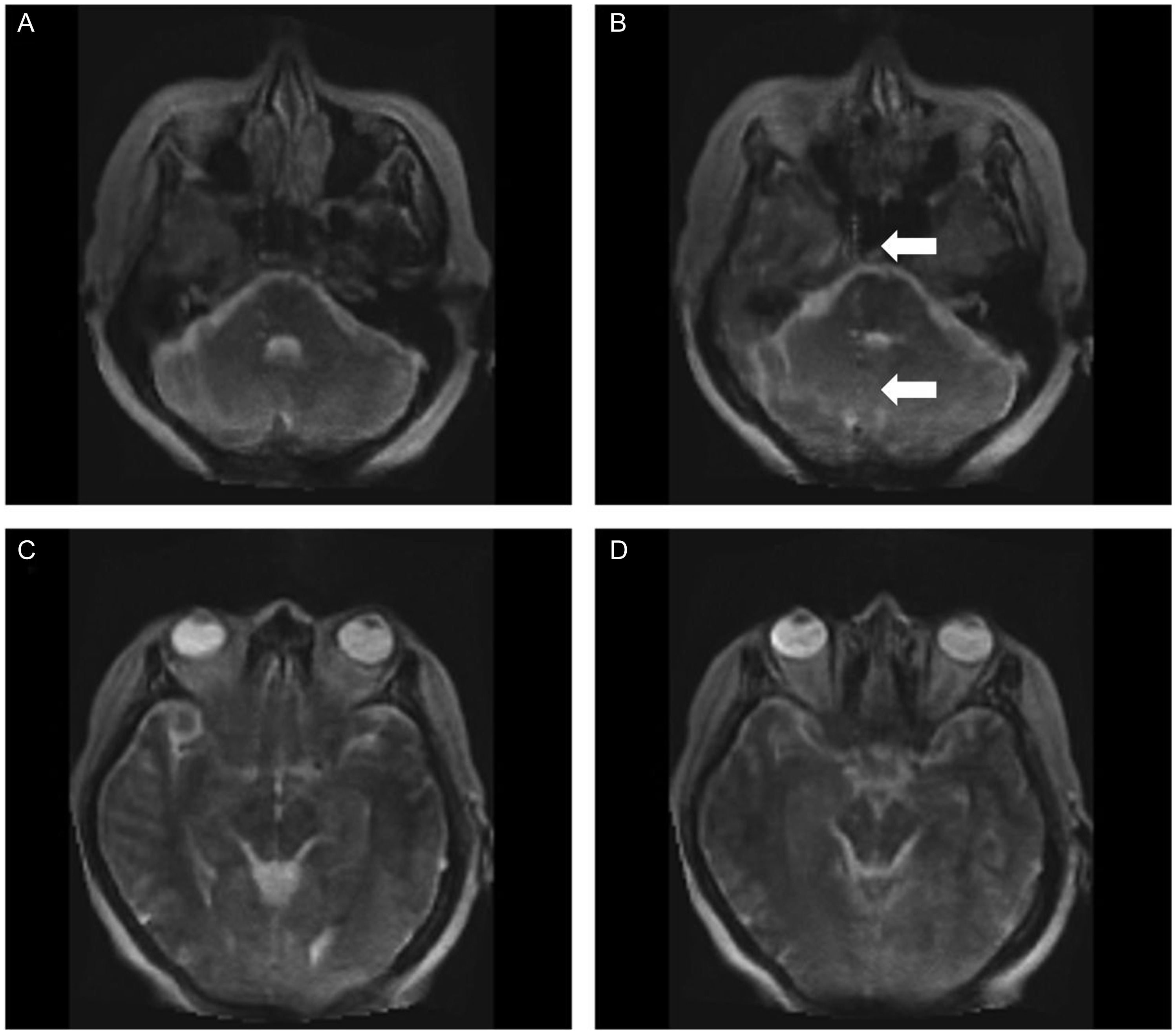
Figure 1. A patient presenting with 2 weeks of sudden intermittent dizziness with left ear tinnitus and left eye decreased vision. Select T2 images (A–D) through the brainstem show the normal appearance of the midbrain and pons. There is no mass in the cerebellopontine angle cisterns. Images from portable MRI are of sufficient quality for diagnostic interpretation. This case also depicts a zipper artifact in image B (arrows).
Image findings for the 50 portable MRI examinations are listed in Table 2. Twenty-eight (56%) were reported as unremarkable, indicating that there was no identified pathology and images were representative of a normal portable MRI head examination. Chronic findings such as frontoparietal volume loss and chronic small vessel ischemic disease were identified in five (10%) and four (8%) of the exams, respectively. Clinically significant findings were identified in five (10%) of the examinations, prompting immediate notification and discussion with the referring physician. These included acute infarct (Figure 2), aneurysm, demyelinating disease (Figure 3), otomastoiditis and an examination where an area of FLAIR hyperintense signal change in the left caudate and lentiform nucleus was identified but deemed nonspecific and correlation with follow-up CT or MRI was recommended (Figure 4C).
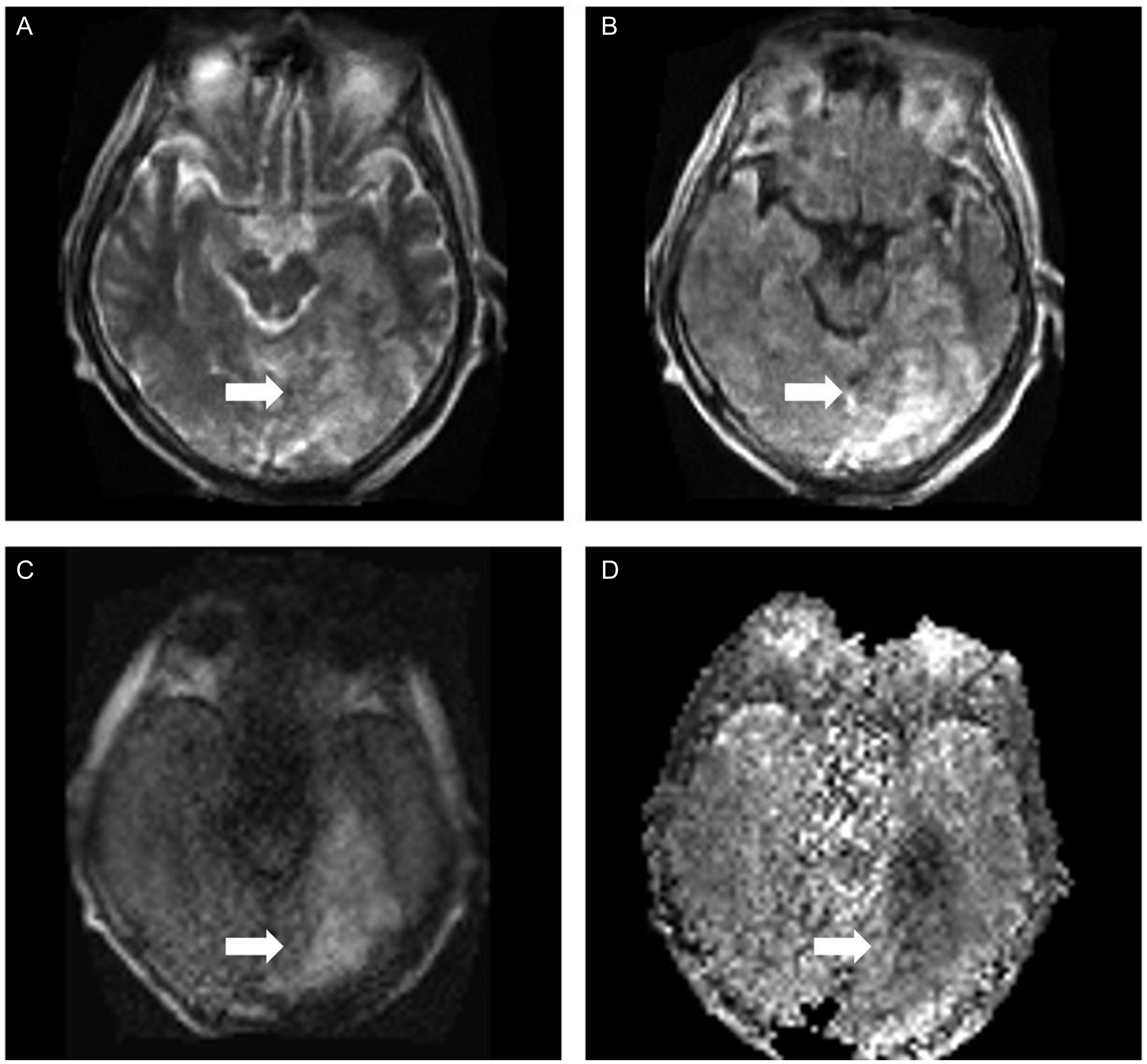
Figure 2. A 60-year-old male presented with right-sided weakness and dysphasia. Ultra-low-field MRI performed approximately 22 hours after symptom onset revealed hyperintensity in the left temporo-occipital lobe (arrows) on T2 (A) and fluid-attenuated inversion recovery (B), with corresponding bright signal intensity on diffusion-weighted imaging (C) and matching dark signal intensity on apparent diffusion coefficient (D). The ultra-low-field MRI features are consistent with acute posterior cerebral artery infarction.
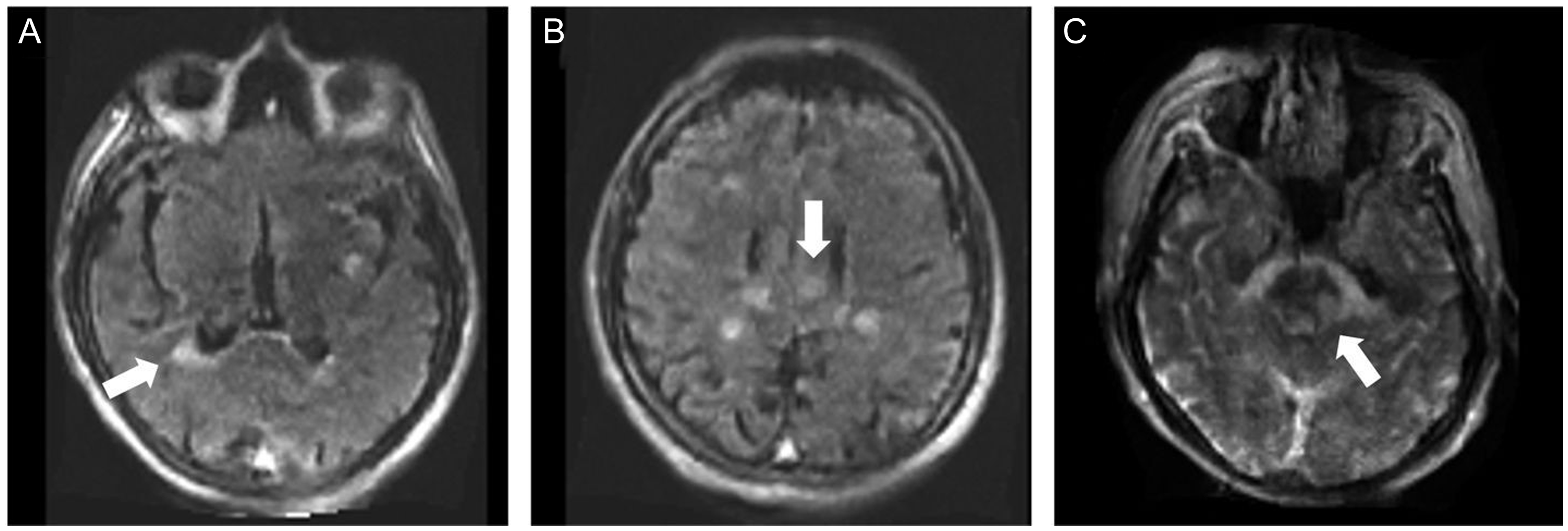
Figure 3. A 42-year-old male presented with 2 weeks of diplopia, right sixth nerve palsy, horizontal nystagmus and mild right facial weakness. CT and CT angiography were negative for any acute pathology. An ultra-low-field MRI was acquired. Fluid-attenuated inversion recovery (A) and (B) and T2 (C) images revealed multiple hyperintense lesions within the periventricular white matter, body of the corpus callosum and left lateral pons (white arrows), highly suspicious for demyelinating plaques.
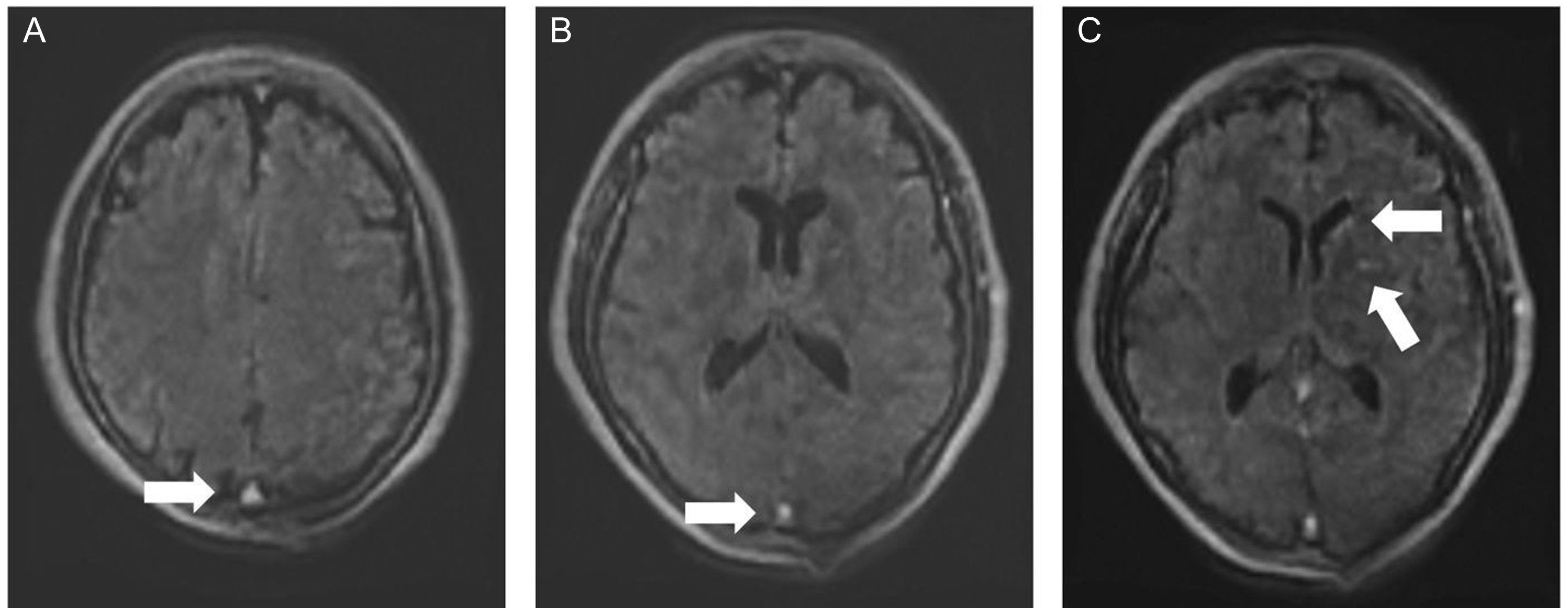
Figure 4. A 44-year-old female presented with 1 week of tingling on the right side of her lips, which spread to the right side of her face, without weakness. Physical examination was otherwise normal. On ultra-low-field MRI, the axial fluid-attenuated inversion recovery (FLAIR) (A) and (B) demonstrated bright signals in the posterior and anterior aspects of the superior sagittal sinus (arrows). There was no corresponding bright signal abnormality on T1 (not shown). This is a normal finding on ultra-low-field portable MRI and does not equate to venous sinus thrombosis. The explanation for this high signal on FLAIR on ultra-low-field MRI is unknown. On the axial slice (C), there are tiny foci of FLAIR hyperintense signal change in the left caudate and lentiform nuclei (white arrows), which are nonspecific and likely artifactual. Follow-up imaging with high-field MRI was recommended.
Table 2. Portable MRI image findings
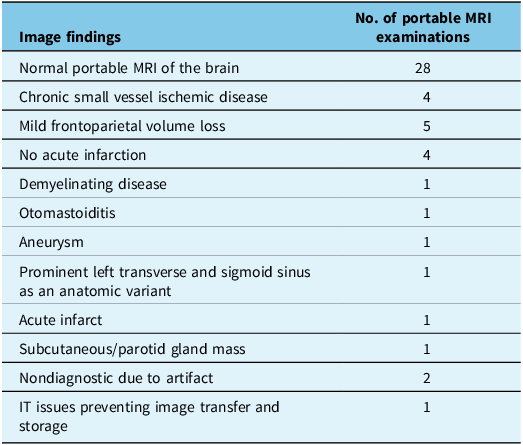
Retrospectively analyzing the clinical presentation, indication for imaging, portable MRI image quality and findings, it is estimated that 27 patients (54%) would not require transfer to a center with conventional MRI imaging due to the availability of portable MRI onsite. For example, a 60-year-old male presenting with right sided weakness and dysphasia underwent a portable MRI for query acute stroke. The portable MRI findings demonstrated a hyperintensity in the left temporal lobe on T2 (Figure 2A) and FLAIR (Figure 2B), with corresponding bright signal intensity on diffusion-weighted imaging (Figure 2C) and dark signal intensity on apparent diffusion coefficient map (Figure 2D). In this case, the patient was diagnosed with acute posterior cerebral artery infarction and did not require transfer for further conventional imaging.
An exemplar case where a patient would require transfer for conventional MRI is shown in Figure 3. A 44-year-old male presented with a 2-week history of diplopia, right-sided facial weakness, right sixth cranial nerve palsy and horizontal nystagmus on physical examination. A previous CT head and CT angiography were negative for any acute pathology. The patient underwent a portable MRI, which demonstrated multiple hyperintense lesions within the periventricular white matter, body of the corpus callosum and left lateral pons (Figure 3A–C). These findings were suggestive of demyelinating disease, likely multiple sclerosis (MS) with a moderate to severe burden of disease. It was recommended that the patient undergo a conventional MRI head and spine with contrast to further document the extent of the disease.
Discussion
These results demonstrate that the implementation of portable MRI at a remote Canadian site is most certainly feasible and offers valuable clinical information. In the context of the Canadian healthcare system, geographic access is one challenge to the provision of equitable services. Portable MRI offers an opportunity to improve access to imaging in such scenarios.
Previous studies have described integrating portable MRI into the ICU, emergency department and low resource settings, allowing for the triaging of patients and earlier identification of pathology. Reference Sheth, Mazurek and Yuen6–Reference Sien, Robinson and Hu9 We suggest that there is a role for portable MRI implementation at sites that do not have access to conventional high-field MRI. Our previous work has shown that the ability of portable MRI to triage which patients require transfer to a center with conventional MRI has economic benefits. Reference DesRoche, Johnson and Hore13 There is also the consideration of the sociocultural benefits of providing care closer to home, and while not the focus of this study, environmental considerations such as the use of portable MRI offer the potential for reduced environmental impact, decreased greenhouse gas emissions and sustainable practices both with respect to decreased patient transfer and lower power and infrastructure requirements.
When discussing the clinical utility of portable MRI, it is important to appreciate that the role of portable MRI is not to replace conventional MRI or CT but to be employed as an adjunct point-of-care device. While advances have been made in hardware design Reference Webb and O’Reilly15 and post-processing image reconstruction algorithms, Reference Zhu, Liu, Cauley, Rosen and Rosen16,Reference Jimeno, Ravi, Jin, Oyekunle, Ogbole and Geethanath17 the ultra-low-field strength results in a lower signal-to-noise ratio per unit time and effectively lower resolution images when compared to images from a conventional 1.5 or 3 T MRI. We noted that neuroradiologists commented on image quality in 24% of cases with mention of either incomplete visualization, motion or zipper artifact. However, there were only two cases (4%) where the image quality was thought to be diagnostically limiting.
Diffusion-weighted imaging can be performed using portable MRI, highlighting its value to help diagnose or exclude acute stroke. However, the current slice thickness for diffusion sequences using portable MRI is 5.8 mm, compared to 3 or 4 mm on conventional MRI that limits the confidence of excluding small posterior fossa strokes. Additionally, portable MRI has limited ability to detect old hemorrhages and calcifications or other forms of brain mineralization as echoplanar sequences such as gradient echo and susceptibility-weighted imaging are not currently available on portable MRI. MR angiography cannot be performed using portable MRI excluding indications such as cerebral aneurysm screening. Further, patients who require intravenous contrast administration (gadolinium-based agents) cannot be scanned with portable MRI, as currently, gadolinium-based contrast agents do not exist that have been approved for clinical use with portable MRI.
Despite these limitations, portable MRI possesses advantages over other imaging modalities, such as a lack of radiation exposure and better visualization of soft tissue compared with CT. Despite the lower image quality, portable MRI offers valuable clinical information as represented by the exemplar cases presented: the identification of acute infarct (Figure 2) and demyelinating disease (Figure 3). These cases demonstrate the value of portable MRI for urgent neurological conditions such as acute stroke or head trauma and for conditions that require frequent reimaging such as MS. There is also clinical value in cases where portable MRI demonstrates no acute pathology. The quality of portable MRI is such that we can confidently rule out acute infarction and detect hydrocephalus, change in ventricular caliber and herniation.
It may be beneficial to adopt a model in which high-field scanners are located in tertiary hospitals, while ultra-low-field scanners are more widely available. Ultra-low-field portable MRI offers the option of augmenting standard-of-care imaging by allowing patient triage, reducing scheduling demands on high-field scanners and decreasing diagnostic delays. Reference Ditkofsky, Lin, Mathur and Bharatha18,Reference Arnold, Freeman, Litt and Stein19
Limitations
This study is limited by the lack of available comparison between portable MRI and CT or conventional MRI. This was a result of the patient population and safeguards with respect to accessing the health information of Indigenous patients for research purposes. Given that the patient population in Moose Factory is largely Indigenous and in consultation with community elders, a chart review of participants’ additional medical data was deemed unnecessary to meet the objective of this study, as the comparison between portable MRI and conventional MRI has been previously validated. Reference Mazurek, Cahn and Yuen7,Reference Wang, Siddiqi and Marino8,Reference Kuoy, Glavis-Bloom and Hovis20–Reference Yuen, Prabhat and Mazurek22 Future work should include a focus on refining clinical indications for portable MRI in remote settings.
Conclusion
The use of ultra-low-field portable MRI in a remote setting in Canada is highly feasible and offers valuable clinical information. It has previously been shown to be of economic benefit. The use of ultra-low-field portable MRI in centers that do not have access to conventional imaging can help improve access to imaging and allow for triage of urgent and emergent clinical presentations. An understanding of the indications and limitations of ultra-low-field MRI is required for appropriate use. This may continue to evolve with improvements in the technology and image reconstruction and post-processing algorithms. Based on this work, we recommend a model where portable MRI is implemented at remote sites with radiology support from larger partner sites with access to conventional MRI. Future work should focus on the integration of ultra-low-field MRI with current standard-of-care systems.
Acknowledgments
The authors thank Dr David Beach for his assistance with overseeing onsite study logistics.
Author contributions
The authors confirm their contributions to the paper as follows: study conception and design: CD, OI, EI; acquisition, analysis and/or interpretation of data: OI, DT, JOJ, IS, BYMK; manuscript preparation: CD, OI; revising manuscript: DT, JOJ, IS, BYMK. All authors reviewed the results and approved the final version of the manuscript.
Funding statement
This work was supported by the William H. Boyd Memorial Fund for Neurological Research, Faculty of Health Sciences, Queen’s University, and the Academic Fund, Department of Diagnostic Radiology, Faculty of Health Sciences, Queen’s University. Hyperfine Inc. has supplied the portable MRI, training and associated equipment.
Competing interests
There is no conflict of interest with any of the authors.