Implications
Intramuscular fibro-adipogenic progenitors reside in the interstitium of muscle fibers, and primary muscle bundles are the major source of intramuscular adipocytes where marbling fat deposits. Expanding intramuscular fibro-adipogenic progenitor pool and enhancing their commitment to adipogenic fate, in addition to their differentiation into lipid-laden adipocytes, provide attractive targets to facilitate marbling fat deposition thus improve palatability of meat.
Introduction
In farm animals, adipocytes are mainly clustered inside subcutaneous, intermuscular, visceral and mesenteric connective tissues, and some are scattered between and within muscle bundles. Fat pads located at subcutaneous, visceral and mesenteric depots have low commercial value and thus are generally considered as a waste for meat production. On the other hand, the quantity and distribution of intramuscular fat, or referred to as marbling fat, is highly desirable for enhancing meat flavor and palatability (Du et al., Reference Du, Huang, Das, Yang, Duarte, Dodson and Zhu2013; Hausman et al., Reference Hausman, Basu, Du, Fernyhough-Culver and Dodson2014; Ngapo et al., Reference Ngapo, Braña Varela and Rubio Lozano2017 and Reference Ngapo, Rubio Lozano and Braña Varela2018). Therefore, there are intensive efforts for increasing marbling fat deposition while reducing the overall fatness of animals.
The contents of intramuscular fat (IMF) are mainly determined by the number and size of intramuscular adipocytes, of which the formation of intramuscular adipocytes is especially important because they provide sites for later marbling fat deposition. Most intramuscular adipocytes are deposited between primary and secondary muscle bundles in the perimysium of beef cattle (Harper and Pethick, Reference Harper and Pethick2004) and pigs (Chen et al., Reference Chen, Wang, Tang, Liu, Cai, Gao, Sun, Yang and Pang2019), while some marbling adipocytes can also be found within muscle bundles in the high-quality grade Japanese Black cattle (Hoshino et al., Reference Hoshino, Suzuki, Yamaguchi and Ohwada1990). There are also intramuscular adipocytes detected in humans (Agley et al., Reference Agley, Rowlerson, Velloso, Lazarus and Harridge2013) and rodents (Bagchi et al., Reference Bagchi, Forss, Mandrup and MacDougald2018).
Recent studies show that adipocytes are derived from a pool of progenitor cells with dual potentials of adipogenic and fibrogenic differentiation, named fibro-adipogenic progenitors (FAPs) (Uezumi et al., Reference Uezumi, Fukada, Yamamoto, Ikemoto-Uezumi, Nakatani, Morita, Yamaguchi, Yamada, Nishino, Hamada and Tsuchida2014). Here, we overviewed the origin and physiological behaviors of FAPs, factors controlling their proliferation and adipogenesis and potential strategies to enhance lipid accumulation in newly formed muscular adipocytes in order to increase marbling fat.
Molecular markers and heterogeneity of fibro-adipogenic progenitors
The origins of muscular fibro-adipogenic progenitors
The muscle stromal vascular fractions (SVFs), containing a mixture of mesenchymal stem/stromal cells (MSCs), fibroblasts, immune cells and endothelial cells (Perruchot et al., Reference Perruchot, Lefaucheur, Barreau, Casteilla and Louveau2013; Wosczyna et al., Reference Wosczyna, Konishi, Perez Carbajal, Wang, Walsh, Gan, Wagner and Rando2019), have been widely used for in vitro studies of intramuscular adipogenesis (Zhou et al., Reference Zhou, Wang, Wang, Zhu, Shu, Liao, Yu, Gao, Xi, Wang, Zhang, Yuan and Jiang2010; Jiang et al., Reference Jiang, Wei, Song, Yang, Peng and Jiang2013; Zhang et al., Reference Zhang, Lu, Chen, Zhao, Guo, Yang and Zang2014; Sun et al., Reference Sun, Qin, Liu, Cai, Chen, Wang and Pang2017 and Reference Sun, Chen, Qin, Liu, Zhao, Yu, Chu, Yang and Pang2018; Chen et al., Reference Chen, Wang, Tang, Liu, Cai, Gao, Sun, Yang and Pang2019); however, the origins of intramuscular adipocytes had remained largely undefined until fairly recently.
In 2010, a subpopulation of non-myogenic mesenchymal stem cells, distinct from myogenic satellite cells, was identified to be the major source of intramuscular adipocytes in mice during muscle regeneration (Uezumi et al., Reference Uezumi, Fukada, Yamamoto, Takeda and Tsuchida2010 and Reference Uezumi, Ito, Morikawa, Shimizu, Yoneda, Segawa, Yamaguchi, Ogawa, Matev, Miyagoe-Suzuki, Takeda, Tsujikawa, Tsuchida, Yamamoto and Fukada2011) and later in humans (Uezumi et al., Reference Uezumi, Fukada, Yamamoto, Ikemoto-Uezumi, Nakatani, Morita, Yamaguchi, Yamada, Nishino, Hamada and Tsuchida2014), and these results are further confirmed by other independent studies (Agley et al., Reference Agley, Rowlerson, Velloso, Lazarus and Harridge2013; Arrighi et al., Reference Arrighi, Moratal, Clément, Giorgetti-Peraldi, Peraldi, Loubat, Kurzenne, Dani, Chopard and Dechesne2015). This subgroup of progenitor cells is platelet derived growth factor receptor α (also named CD140a)-positive (PDGFRA + ), and displays bipotency of differentiation into both lipid-laden adipocytes and collagen I-expressing fibroblasts, thus defined as FAPs (fibro/adipogenic progenitors). Bipotent PDGFRA+ fibroblasts, unlike muscle satellite cells that locate beneath the basal lamina, are located in the interstitial space between muscle fibers and bundles of mice (Uezumi et al., Reference Uezumi, Fukada, Yamamoto, Takeda and Tsuchida2010) and humans (Uezumi et al., Reference Uezumi, Fukada, Yamamoto, Ikemoto-Uezumi, Nakatani, Morita, Yamaguchi, Yamada, Nishino, Hamada and Tsuchida2014), where intramuscular fat accumulates (Duarte et al., Reference Duarte, Paulino, Das, Wei, Serão, Fu, Harris, Dodson and Du2013). Further studies showed that a large portion of intramuscular FAPs is generated from the embryonic Odd skipped-related 1 (Osr1)-positive fibroblasts (Vallecillo-García et al., Reference Vallecillo-García, Orgeur, Vom Hofe-Schneider, Stumm, Kappert, Ibrahim, Börno, Hayashi, Relaix, Hildebrandt, Sengle, Koch, Timmermann, Marazzi, Sassoon, Duprez and Stricker2017), and after birth, the levels of Osr1 in quiescent intramuscular FAPs become quite low (Stumm et al., Reference Stumm, Vallecillo-García, Vom Hofe-Schneider, Ollitrault, Schrewe, Economides, Marazzi, Sassoon and Stricker2018). New postnatal FAPs mainly arise from pre-existing muscle-resident PDGFRA+ cells, not or rarely from PDGFRA– cells (Uezumi et al., Reference Uezumi, Ito, Morikawa, Shimizu, Yoneda, Segawa, Yamaguchi, Ogawa, Matev, Miyagoe-Suzuki, Takeda, Tsujikawa, Tsuchida, Yamamoto and Fukada2011).
Bovine PDGFRA+ cells within muscular SVFs are initially purified by our group (Huang et al., Reference Huang, Das, Yang, Zhu and Du2012a). PDGFRA+ fibroblasts isolated from bovine skeletal muscles (Huang et al., Reference Huang, Das, Yang, Zhu and Du2012a; Guan et al., Reference Guan, Hu, Liu, Xing, Zhou, Liang, Yang, Jin, Bao, Gao, Du, Li and Zhang2017) can be induced to differentiate into adipocytes by the traditional adipogenic induction cocktail, despite at low efficiency (Ma et al., Reference Ma, Wang, Wang, Gomez, Zhu and Du2018b). Besides, the abundance of PDGFRA in finishing Angus is correlated with its higher IMF contents when compared with Nellore (Martins et al., Reference Martins, Sanglard, Silva, Chizzotti, Rennó, Serão, Silva, Guimarães, Ladeira, Dodson, Du and Duarte2015). Thus PDGFRA is a marker of intramuscular adipocyte progenitors in beef cattle (Huang et al., Reference Huang, Das, Yang, Zhu and Du2012a; Miao et al., Reference Miao, Zhang, Fu, Yang, Zhu, Dodson and Du2016). In pigs, PDGFRA+ cells are located in the gaps of myofibers in longissimus dorsi muscle, and more PDGFRA+ cells are detected in longissimus dorsi muscle of fat-type pigs compared with that of lean-type pigs at 180 days of age (Sun et al., Reference Sun, Qin, Liu, Cai, Chen, Wang and Pang2017), albeit no difference was observed in the abundance of PDGFRA+ cells or PDGFRA expression between longissimus thoracis (with higher IMF contents) and semitendinosus muscle (with lower IMF) in 180-day-old pigs (Chen et al., Reference Chen, Wang, Tang, Liu, Cai, Gao, Sun, Yang and Pang2019).
Mouse muscular PDGFRA+ cells also express other mesenchymal markers, such as mesenchymal intermediate filament, Vimentin, together with the well-discussed adipogenesis repressor, delta like non-canonical Notch ligand 1 (Dlk1, also known as preadipocyte factor 1 or Pref1) (Uezumi et al., Reference Uezumi, Fukada, Yamamoto, Takeda and Tsuchida2010). Of note, Sca1 (Stem cells antigen 1)-positive cells overlap with over 85% of PDGFRA+ cells in undamaged mouse muscle and up to 98% in injured muscles (Joe et al., Reference Joe, Yi, Natarajan, Le Grand, So, Wang, Rudnicki and Rossi2010), and Sca1 is also commonly used to sort mouse FAPs (Fiore et al., Reference Fiore, Judson, Low, Lee, Zhang, Hopkins, Xu, Lenzi, Rossi and Lemos2016; Judson et al., Reference Judson, Low, Eisner, Rossi and Ryall2017). However, a new study uncovered a subset of intramuscular Sca1+/PDGFRA– cells within endothelial cells which derived from Myf5+ progenitors in mice (Huang et al., Reference Huang, Schulz, Beauvais, Tseng and Gussoni2014). The CD15 and PDGFRA label the similar subpopulation of cells within human skeletal muscle (Arrighi et al., Reference Arrighi, Moratal, Clément, Giorgetti-Peraldi, Peraldi, Loubat, Kurzenne, Dani, Chopard and Dechesne2015).
The heterogeneity of intramuscular fibro-adipogenic progenitors
Intramuscular FAPs, a subgroup of mesenchymal stromal cells (Dominici et al., Reference Dominici, Le Blanc, Mueller, Slaper-Cortenbach, Marini, Krause, Deans, Keating, Prockop and Horwitz2006), constitute a heterogeneous pool of cells with divergent lineage dynamics. For instance, a sub-fraction of mouse intramuscular FAPs can differentiate as committed adipogenic progenitors, while the others express fibroblast markers even in the pro-adipogenic medium (Joe et al., Reference Joe, Yi, Natarajan, Le Grand, So, Wang, Rudnicki and Rossi2010). More than 90% of FAPs derived from mouse muscle exhibit adipogenic potential, and the frequency reduces to ~60% in injured muscle (Uezumi et al., Reference Uezumi, Ito, Morikawa, Shimizu, Yoneda, Segawa, Yamaguchi, Ogawa, Matev, Miyagoe-Suzuki, Takeda, Tsujikawa, Tsuchida, Yamamoto and Fukada2011), while in another study, the frequency is ~35% (Joe et al., Reference Joe, Yi, Natarajan, Le Grand, So, Wang, Rudnicki and Rossi2010). The ratio of FAPs with adipogenic capacity is reported to be ~30% in unperturbed skeletal muscles of human (Uezumi et al., Reference Uezumi, Fukada, Yamamoto, Ikemoto-Uezumi, Nakatani, Morita, Yamaguchi, Yamada, Nishino, Hamada and Tsuchida2014). Accordingly, PDGFRA-sorted cells from bovine SVF display different in vitro adipogenic capabilities with distinct gene expression patterns (Huang et al., Reference Huang, Das, Yang, Zhu and Du2012a).
Furthermore, some, but not all of, FAPs are ciliated in mouse (Kopinke et al., Reference Kopinke, Roberson and Reiter2017) and human skeletal muscles (Arrighi et al., Reference Arrighi, Lypovetska, Moratal, Giorgetti-Peraldi, Dechesne, Dani and Peraldi2017). Primary cilia are likely to mediate extracellular signals, such as transforming growth factor (TGF) β (Clement et al., Reference Clement, Ajbro, Koefoed, Vestergaard, Veland, de Jesus, Pedersen, Benmerah, Andersen, Larsen and Christensen2013) and insulin-like growth factor (IGF) 1 (Dalbay et al., Reference Dalbay, Thorpe, Connelly, Chapple and Knight2015) in human cell lines or Hedgehogs (Hh) in mouse (Kopinke et al., Reference Kopinke, Roberson and Reiter2017), which regulate the lineage commitment and adipogenic differentiation of FAPs. The primary cilium shortens but maintains a signaling activity when human adipose and muscular FAPs morphs into fibroblasts ex vivo (Arrighi et al., Reference Arrighi, Lypovetska, Moratal, Giorgetti-Peraldi, Dechesne, Dani and Peraldi2017). On the other hand, the primary cilium of mouse muscular FAPs elongates at the early stage of adipogenic differentiation and then disappears in the mature adipocytes (Kopinke et al., Reference Kopinke, Roberson and Reiter2017).
Moreover, PDGFRA+ cells in mouse subcutaneous and visceral white adipose pads can be classified according to CD9 expression levels, and CD9low/PDGFRA+ progenitors can differentiate into adipocytes with dietary high-fat challenge, and CD9high/PDGFRA+ cells are fibrotic (Marcelin et al., Reference Marcelin, Ferreira, Liu, Atlan, Aron-Wisnewsky, Pelloux, Botbol, Ambrosini, Fradet, Rouault, Hénégar, Hulot, Poitou, Torcivia, Nail-Barthelemy, Bichet, Gautier and Clément2017), supporting the heterogeneity of FAPs.
Proliferation of fibro-adipogenic progenitors
The importance of the proliferation of fibro-adipogenic progenitors on marbling
Interestingly, discernable intramuscular adipocytes in domestic animals appear later than adipocytes located in other fat depots (Du et al., Reference Du, Huang, Das, Yang, Duarte, Dodson and Zhu2013 and Reference Du, Wang, Fu, Yang and Zhu2015). In beef cattle, adipocyte hyperplasia in visceral and subcutaneous fat greatly slows down after birth, but there are apparent cell hyperplasia observed in IMF depots in calves between 11 and 15 months of age (Cianzio et al., Reference Cianzio, Topel, Whitehurst, Beitz and Self1985). Of note, the later-formed intramuscular fat flecks tend to be smaller and rounder (Albrecht et al., Reference Albrecht, Teuscher, Ender and Wegner2006), thus benefiting marbling scores (Cheng et al., Reference Cheng, Cheng, Sun and Pu2015). Consistently, in Asian cattle breeds with high marbling, intramuscular adipose tissue tends to be small and evenly distributed (Motoyama et al., Reference Motoyama, Sasaki and Watanabe2016). Previous studies found that Wagyu FAPs are proliferated faster than Angus FAPs (May et al., Reference May, Savell, Lunt, Wilson, Laurenz and Smith1994; Wei et al., Reference Wei, Fu, Liang, Zhu, Jiang, Parish, Dodson, Zan and Du2015), which correlated with higher FAP density in Wagyu muscle (Duarte et al., Reference Duarte, Paulino, Das, Wei, Serão, Fu, Harris, Dodson and Du2013). Collectively, these studies suggest the higher proliferation capacity of FAPs contributes to the high marbling fat deposition (Albrecht et al., Reference Albrecht, Teuscher, Ender and Wegner2006; Kern et al., Reference Kern, Pritchard, Blair, Scramlin and Underwood2014; Wang et al., Reference Wang, Yang, Harris, Nelson, Busboom, Zhu and Du2016; Kruk et al., Reference Kruk, Bottema, Reyes-Veliz, Forder, Pitchford and Bottema2018).
Factors regulating proliferation of intramuscular fibro-adipogenic progenitors
The mechanism underlying the proliferation of muscular FAP has been extensively studied using mice as models. As described earlier, FAPs acutely expand in injured muscles, and the activation of FAPs is necessary for muscle repair (Joe et al., Reference Joe, Yi, Natarajan, Le Grand, So, Wang, Rudnicki and Rossi2010; Fiore et al., Reference Fiore, Judson, Low, Lee, Zhang, Hopkins, Xu, Lenzi, Rossi and Lemos2016). During muscle regeneration, activated FAPs obtain transient expression of Osr1. Odd skipped-related 1-positive FAPs either undergo apoptosis or return to the resident FAP pool after regeneration. These cells can also become adipocytes in fatty degeneration (Stumm et al., Reference Stumm, Vallecillo-García, Vom Hofe-Schneider, Ollitrault, Schrewe, Economides, Marazzi, Sassoon and Stricker2018). During muscle injury, intramuscular FAPs’ accumulation is preceded by the appearance of inflammatory infiltration (Tidball, Reference Tidball2017), indicating that immune cells might regulate the activation of FAPs. Besides, age-related decline of dermal PDGFRA+ is in parallel with the loss of immune cells (Zhang et al., Reference Zhang, Chen, Guerrero-Juarez, Li, Tong, Liang, Liggins, Chen, Chen, Li, Hata, Zheng, Plikus and Gallo2019a). In chronic muscle damage, TGFβ1 (transforming factor β1) production in macrophages is elevated, which inhibits apoptosis (Lemos et al., Reference Lemos, Babaeijandaghi, Low, Chang, Lee, Fiore, Zhang, Natarajan, Nedospasov and Rossi2015) and promotes proliferation of PDGFRA+ cells in mouse muscle (Uezumi et al., Reference Uezumi, Ito, Morikawa, Shimizu, Yoneda, Segawa, Yamaguchi, Ogawa, Matev, Miyagoe-Suzuki, Takeda, Tsujikawa, Tsuchida, Yamamoto and Fukada2011). In acutely damaged muscle, the expression of tumor necrosis factor α (TNFα) in macrophages is elevated, and TNFα directly induces apoptosis of FAPs (Lemos et al., Reference Lemos, Babaeijandaghi, Low, Chang, Lee, Fiore, Zhang, Natarajan, Nedospasov and Rossi2015). Upon injection of cardiotoxin, interleukin (IL)-4 released by eosinophils enforces the proliferation of intramuscular FAPs (Heredia et al., Reference Heredia, Mukundan, Chen, Mueller, Deo, Locksley, Rando and Chawla2013). Because inflammatory reaction is heavily dependent on tissue environment and the time elapsed since injury, it is difficult to clearly decipher the responses of FAPs to inflammatory cells.
Besides, growth factors such as platelet derived growth factor (PDGF) can provoke the proliferation of murine PDGFRA+ FAP cells through activating PI3K (phosphatidylinositol 3- kinase) - AKT (protein kinase B) and MEK2 (mitogen activated protein kinase 2) signaling (Uezumi et al., Reference Uezumi, Fukada, Yamamoto, Ikemoto-Uezumi, Nakatani, Morita, Yamaguchi, Yamada, Nishino, Hamada and Tsuchida2014). Genetic knockout of vascular endothelial growth factor receptor 2 (VEGFR2) in mouse PDGFRA+ cells blocks the pro-proliferation effects of retinoic acid on FAPs (Wang et al., Reference Wang, Fu, Liang, Wang, Yang, Zou, Nie, Zhao, Gao, Zhu, de Avila, Maricelli, Rodgers and Du2017), indicating that vascular endothelial growth factor (VEGF) signaling promotes the proliferation of FAPs.
Until now, our knowledge on intramuscular FAP hyperplasia in livestock animals remains rudimentary. The SVF cells containing FAPs derived from Wagyu muscle possess higher proliferative ability than those from Angus (May et al., Reference May, Savell, Lunt, Wilson, Laurenz and Smith1994; Wei et al., Reference Wei, Fu, Liang, Zhu, Jiang, Parish, Dodson, Zan and Du2015). Also, SVF cells proliferate faster in fat-type Bamei than lean-type Landrace (Zhang et al., Reference Zhang, Lu, Chen, Zhao, Guo, Yang and Zang2014). Certain nutrients, like conjugated linoleic acid (CLA) (Meadus et al., Reference Meadus, MacInnis and Dugan2002) and vitamin A (Harris et al., Reference Harris, Wang, Deavila, Busboom, Maquivar, Parish, McCann, Nelson and Du2018; Kruk et al., Reference Kruk, Bottema, Reyes-Veliz, Forder, Pitchford and Bottema2018), can augment marbling via increasing the number of intramuscular adipocyte precursors, although detailed mechanisms remain elusive.
Adipogenic differentiation of fibro-adipogenic progenitors
Regulation of the adipogenic differentiation of fibro-adipogenic progenitors
Fibro-adipogenic progenitors possess both fibrogenic and adipogenic potentials, and fibrogenesis of intramuscular FAPs has been depicted in our previous review (Miao et al., Reference Miao, Zhang, Fu, Yang, Zhu, Dodson and Du2016). Because both adipocytes and fibroblasts are derived from FAPs, it had been postulated that enhancing adipogenic differentiation may correspondingly reduce fibrogenesis. Though this notion was supported by several studies (Huang et al., Reference Huang, Das, Yang, Zhu and Du2012a; Marcelin et al., Reference Marcelin, Ferreira, Liu, Atlan, Aron-Wisnewsky, Pelloux, Botbol, Ambrosini, Fradet, Rouault, Hénégar, Hulot, Poitou, Torcivia, Nail-Barthelemy, Bichet, Gautier and Clément2017), enhanced adipogenesis and fibrogenesis are both detected in Wagyu skeletal muscles (Duarte et al., Reference Duarte, Paulino, Das, Wei, Serão, Fu, Harris, Dodson and Du2013), which could be due to the enhanced proliferation of FAPs resulting in the elevation of both fibrogenesis and adipogenesis. In agreement, enhanced adipogenesis and fibrogenesis were also observed in beef cattle offspring subjected to maternal overnutrition (Duarte et al., Reference Duarte, Gionbelli, Paulino, Serão, Nascimento, Botelho, Martins, Filho, Dodson, Guimarães and Du2014). Besides, comparable fibrogenesis and collagen contents are found in Angus and Nellore cattle yet differing in IMF contents (Martins et al., Reference Martins, Sanglard, Silva, Chizzotti, Rennó, Serão, Silva, Guimarães, Ladeira, Dodson, Du and Duarte2015). These data suggest that enhanced intramuscular adipogenesis is not necessarily correlated with compromised fibrogenesis, and FAP proliferation has a major role in determining both processes.
The Zinc finger protein (Zfp) 423, a multi-zinc finger transcription factor, stands out as a key player in the commitment of progenitor cells to adipogenic lineage, which induces peroxisome proliferator activated receptor γ (PPARγ) expression, which commits FAPs to preadipocytes, as well as converts preadipocytes to mature adipocytes (Gupta et al., Reference Gupta, Mepani, Kleiner, Lo, Khandekar, Cohen, Frontini, Bhowmick, Ye, Cinti and Spiegelman2012). Zfp423 is abundant in bovine SVF colonies with higher adipogenic capability, and enforced expression of Zfp423 propels adipogenic differentiation in low adipogenic cells and vice versa (Huang et al., Reference Huang, Das, Yang, Zhu and Du2012a). In agreement, bta-miR-23a blocks adipogenic genes expression and lipid accumulation in bovine intramuscular FAPs via directly targeting Zfp423 (Guan et al., Reference Guan, Hu, Liu, Xing, Zhou, Liang, Yang, Jin, Bao, Gao, Du, Li and Zhang2017).
Adipogenic differentiation of intramusclar compared to other fat depots
Generally, the lipid-storing capacity of intramuscular adipocytes is lower than subcutaneous adipocytes, consistent with the later development of intramuscular fat as compared to other fat depots. Indeed, the initial expression of genes related to lipogenesis and lipolysis in intramuscular preadipocytes is relatively slower when compared with subcutaneous preadipocytes (Wang et al., Reference Wang, Zhou, Shu, Wang, Zhu, Gao, Xi, Zhang, Yuan and Jiang2013), and gene expression and/or activities of lipogenic and lipolytic enzymes are much lower in intramuscular fat than subcutaneous adipocytes (Jiang et al., Reference Jiang, Wei, Song, Yang, Peng and Jiang2013). The sizes of adipocytes and lipid droplets are smaller in human intramuscular FAP-derived adipocytes than subcutaneous adipocytes (Arrighi et al., Reference Arrighi, Moratal, Clément, Giorgetti-Peraldi, Peraldi, Loubat, Kurzenne, Dani, Chopard and Dechesne2015). Important similarities are identified in livestock animals. The diameter of bovine intramuscular adipocytes is smaller than those in subcutaneous adipose depots (Smith and Crouse, Reference Smith and Crouse1984). Likewise, intramuscular fat contains less lipid than subcutaneous adipose tissue in pigs (Kouba and Bonneau, Reference Kouba and Bonneau2009). The lower lipogenic capacity of intramuscular adipocytes is further confirmed by proteomic analysis (Gondret et al., Reference Gondret, Guitton, Guillerm-Regost and Louveau2008), consistent with microarray analysis of gene expression in pigs (Zhou et al., Reference Zhou, Wang, Wang, Zhu, Shu, Liao, Yu, Gao, Xi, Wang, Zhang, Yuan and Jiang2010). In alignment, the expression of leptin, an adipokine primarily secreted by lipid-laden adipocytes, is lower in intramuscular compared to subcutaneous fat (Gardan et al., Reference Gardan, Gondret and Louveau2006). Recent RNA-seq study further confirmed the lower lipid metabolic capacity of intramuscular adipose tissue as compared to subcutaneous fat (Huang et al., Reference Huang, Zhang, Li and Miao2017).
The less maturity of intramuscular fat compared to other fat depots renders it less responsive to hormonal stimuli which alter lipid metabolism. Insulin-induced lipogenesis and catecholamine-induced lipolysis are lower in intramuscular fat compared with subcutaneous and perirenal adipocytes in growing pigs (Gardan et al., Reference Gardan, Gondret and Louveau2006). Intramuscular SVFs isolated from semitendinosus muscles in neonatal pigs are less sensitive to glucocorticoids than that from subcutaneous adipose (Hausman and Poulos, Reference Hausman and Poulos2004), correlated with their lower adipogenic activity (Chu et al., Reference Chu, Wei, Han, Gao, Liu, Tian, Jiang, Zhang and Chen2017). The responses of intramuscular SVFs to other pro-adipogenic components, such as thiazolidinedione, also differ from subcutaneous counterparts (Poulos and Hausman, Reference Poulos and Hausman2006).
The microenvironment of intramuscular fibro-adipogenic progenitors
The most significant difference between intramuscular FAPs and FAPs in other fat depots is the microenvironment. In an early study, purified intramuscular FAPs generate adipocytes only when transplanted to subcutaneous fat pads and glycerol-injected muscle, but not in healthy and intact muscle (Joe et al., Reference Joe, Yi, Natarajan, Le Grand, So, Wang, Rudnicki and Rossi2010). Besides, glycerol injection establishes a degenerative environment (more intramuscular adipocytes) and cardiotoxin injection generates a regenerative niche (less intramuscular adipocytes) (Mahdy et al., Reference Mahdy, Lei, Wakamatsu, Hosaka and Nishimura2015), and intramuscular FAPs reciprocally transplanted between degenerative and regenerative skeletal muscles can well adapt to different differentiation fates according to the new environment in mice (Uezumi et al., Reference Uezumi, Fukada, Yamamoto, Takeda and Tsuchida2010). These reports highlight the dominant regulatory effects of microenvironment on the differentiation from intramuscular FAPs into adipocytes. In addition to skeletal myofibers, infiltrating immune cells within skeletal muscles and others together build up a highly specialized niche environment for intramuscular FAPs (Figure 1).

Figure 1 A schematic sketch of microenvironment niche affecting the proliferation and adipogenic differentiation of FAPs in mouse, human or pigs. FAPs, fibro-adipogenic progenitors; FSP1, fibroblast-specific protein-1; IL-15, interleukin 15; IL-4, interleukin 4; PDGFRA, platelet derived growth factor receptor alpha; TGFβ1, transforming growth factor β 1; Sca1+, Stem cells antigen 1 positive; BMP, bone morphogenetic protein.
The influence of skeletal myofibers and satellite cells
Early experiment in mice has shown that the adipogenesis of intramuscular FAPs can be strongly inhibited by the co-culture with myogenic cells (Uezumi et al., Reference Uezumi, Fukada, Yamamoto, Takeda and Tsuchida2010), indicating a cross-talk between intramuscular FAPs and myofibers. Muscle conditioned medium (MCM) was recently used to mimic the in vivo paracrine effects of skeletal muscle, and MCM can restrain the proliferation and differentiation of porcine subcutaneous preadipocytes (Han et al., Reference Han, Wei, Chu, Liu, Tian, Jiang and Chen2017). Myostatin is one of cytokines secreted from skeletal muscle, named as ‘myokines’. Myostatin production in skeletal muscle is stimulated during chronic kidney disease in mouse model, and the increased myostatin promotes intramuscular FAP proliferation and conversion into fibrocytes (Dong et al., Reference Dong, Dong, Chen, Mitch and Zhang2017). Myostatin can repress porcine glucocorticoid receptor expression in intramuscular adipocytes via elevating DNA methylation levels in its promoter (Chu et al., Reference Chu, Wei, Han, Gao, Liu, Tian, Jiang, Zhang and Chen2017). Myostatin also reduces glucocorticoid receptor at post-transcription level by upregulating expression of miR-124-3p in mice (Liu et al., Reference Liu, Zhang, Wei, Liu, Tian, Han, Zhang, Wu and Chen2019). Interleukin 15, another muscle-derived cytokine (Quinn, Reference Quinn2008), stimulates the proliferation of intramuscular FAPs, and impedes intramuscular FAP differentiating into adipocytes with upregulated Hedgehog signaling in mice (Kang et al., Reference Kang, Yang, Shi, Xie, Zhu, Zheng, Zhang, Ge, Bian, Lv, Wang, Zhou and Tang2018). Besides, irisin, an exercise-induced myokine, reduces preadipocytes differentiation in humans (Huh et al., Reference Huh, Dincer, Mesfum and Mantzoros2014).
The content of IMF varies among different muscles in the same animal (Sharma et al., Reference Sharma, Gandemer and Goutefongea1987; Font-i-Furnols et al., Reference Font-i-Furnols, Brun and Gispert2019, likely due to the difference in muscle fiber composition and locomotion (Picard et al., Reference Picard, Gagaoua, Al-Jammas, De Koning, Valais and Bonnet2018). The content of IMF is higher in the belly and lower in the ham (Kouba and Bonneau, Reference Kouba and Bonneau2009). For pigs, the most cranial part of loin presents the highest IMF content, as well as the Biceps femoris muscle of ham (Font-i-Furnols et al., Reference Font-i-Furnols, Brun and Gispert2019). As mentioned earlier, SVFs, which contain FAPs, derived from longissimus thoracis muscle present earlier and greater lipid accumulation than those from semitendinosus muscle, which is consistent with the higher IMF content in longissimus thoracis (Chen et al., Reference Chen, Wang, Tang, Liu, Cai, Gao, Sun, Yang and Pang2019).
Besides the regulatory effects of myofibers on the FAPs, it was recently reported that satellite cells are able to inhibit fibrogenesis of FAPs through secreting exosomes containing miR-206, a process believed to be critical for suppressing fibrogenesis induced by skeletal muscle hypertrophy (Fry et al., Reference Fry, Kirby, Kosmac, McCarthy and Peterson2017).
The influence of immune cells
Immune cells also regulate the fates of intramuscular FAPs. Fibro-adipogenic progenitors and macrophages (CD68 positive) are located in very close proximity in human degenerating skeletal muscles (Moratal et al., Reference Moratal, Raffort, Arrighi, Rekima, Schaub, Dechesne, Chinetti and Dani2018), suggesting a potential interaction between intramuscular FAPs and macrophages in vivo.
Macrophages are schematically classified into ‘proinflammatory’ M1 and ‘anti-inflammatory’ M2 subgroups (Murray et al., Reference Murray, Allen, Biswas, Fisher, Gilroy, Goerdt, Gordon, Hamilton, Ivashkiv, Lawrence, Locati, Mantovani, Martinez, Mege, Mosser, Natoli, Saeij, Schultze, Shirey, Sica, Suttles, Udalova, van Ginderachter, Vogel and Wynn2014). ‘Proinflammatory’ M1 macrophages are recruited at the initial stage of muscle regeneration, and ‘anti-inflammatory’ M2 macrophages are subsequently activated in a later regeneration phase (Chazaud, Reference Chazaud2016), although a new study found that both M1 and M2 macrophages are broadly activated at the early stage of acute skeletal muscle injury (Wang et al., Reference Wang, Zhao, Ransohoff and Zhou2018). The transition from a pro- to anti-inflammatory status in the niche profoundly affects intramuscular adipogenesis in mice (Dammone et al., Reference Dammone, Karaz, Lukjanenko, Winkler, Sizzano, Jacot, Migliavacca, Palini, Desvergne, Gilardi and Feige2018). Indirect co-culture with conditioned media showed that cytokines secreted by IL-1β-polarized macrophages drastically reduce intramuscular FAP adipogenic potential via stimulating SMAD2 (SMAD family member 2) signaling, and factors released by IL-4-polarized macrophages conversely enhance cellular lipid accumulation and expression of adipogenic markers, thus facilitating intramuscular FAPs adipogenesis (Moratal et al., Reference Moratal, Raffort, Arrighi, Rekima, Schaub, Dechesne, Chinetti and Dani2018).
Specifically, TGFβ1, secreted from macrophages during the regeneration phase in damaged muscle, is the most critical profibrogenic cytokine via stimulating the receptor-SMAD cascades (Miao et al., Reference Miao, Zhang, Fu, Yang, Zhu, Dodson and Du2016). Transforming growth factor β1 represses adipogenic differentiation of FAPs both in vivo and ex vivo (Lee, Reference Lee2018; Zhang et al., Reference Zhang, Chen, Guerrero-Juarez, Li, Tong, Liang, Liggins, Chen, Chen, Li, Hata, Zheng, Plikus and Gallo2019a). Knockdown of TGFβ1 receptor accelerates adipogenic differentiation in porcine preadipocytes (Zhang et al., Reference Zhang, Gao, Xu, Wang, Fu, Liu, Han, Jiang, Yuan and Zhang2019b) and murine 3T3-L3 cells (Zhang et al., Reference Zhang, Chang, Li, Gao, Wang, Ma, Li and Wang2015). Furthermore, the inhibitory effects of TGFβ1 on intramuscular adipogenesis are time-dependent. Co-injection of TGFβ1 with glycerol presents greater repressive effects than its administration 4 days following glycerol injection (Mahdy et al., Reference Mahdy, Warita and Hosaka2017). In aggregate, the differentiation fates of intramuscular FAPs are regulated by the intensive interaction with the surrounding macrophages and other immune cells.
However, studies discussed earlier were mainly conducted under pathological conditions, and there is currently lack of evidence about the contribution of immune cells in FAP differentiation and intramuscular marbling development in domestic animals under physiological conditions. A recent study discovers that genes related with T-cell activation are differentially expressed in porcine muscles divergent in feed efficiency and product quality (Horodyska et al., Reference Horodyska, Wimmers, Reyer, Trakooljul, Mullen, Lawlor and Hamill2018), indicating a possible interaction between immune cells and myofibers in skeletal muscle, likely with intramuscular FAPs under homeostatic conditions in farm animals.
The influence of fibroblasts and other cells
A latest study on mouse white fat suggests the critical contribution of fibroblasts to adipogenesis (Zhang et al., Reference Zhang, Gao, Zhao, Gao, Wu, Han, Qiao, Luo, Yang, Chen and Ge2018). Fibroblast-specific protein-1 (FSP1) is a marker for resident fibroblasts in some tissues including skeletal muscle. Fibroblast-specific protein-1+ fibroblasts in WAT are adjacent to the preadipocytes but devoid of adipogenic potential. Ablation of FSP1+ fibroblasts results in loss of adiposity, arguing that the FSP1+ fibroblasts function in providing an essential adipogenic niche for FAPs (Zhang et al., Reference Zhang, Gao, Zhao, Gao, Wu, Han, Qiao, Luo, Yang, Chen and Ge2018). In this regard, it is intriguing to speculate the interaction between non-adipogenic fibroblasts and intramuscular adipogenesis in livestock animals.
Another recent study described the inhibitory role of Sca-1+/CD31+/PDGFRA– myo-endothelial progenitors on the adipogenic differentiation of FAPs (Huang et al., Reference Huang, Schulz, Beauvais, Tseng and Gussoni2014). Myo-endothelial progenitors are a group of newly identified subset of endothelial cells developmentally derived from Myf5 lineage and are located in the inter-myofiber spaces. Huang and colleagues identified that myo-endothelial progenitors inhibit intramuscular adipogenesis through bone morphogenetic protein (BMP) signaling. Deletion of BMP receptor 1a (Bmpr1a) in Myf5+ cells abolished the inhibitory effect of myo-endothelial progenitors on the adipogenic differentiation of FAPs, resulting in enhanced intramuscular adipogenesis in mice (Huang et al., Reference Huang, Schulz, Beauvais, Tseng and Gussoni2014).
Hedgehog signaling exerts a conserved and inhibitory role on fat formation (Suh et al., Reference Suh, Gao, McKay, McKay, Salo and Graff2006). In skeletal muscle, Hedgehog signals (e.g. Desert Hedgehog, Dhh) are primarily produced by Schwann cells. Desert Hedgehog expression in Schwann cells is induced by cardiotoxin injection, and the extracellular Dhh signal can transduce into intramuscular FAPs via the primary cilia on their cell surfaces and block adipogenesis of intramuscular FAPs (Kopinke et al., Reference Kopinke, Roberson and Reiter2017).
Nutritional strategies to improve marbling via targeting intramuscular fibro-adipogenic progenitors
As reviewed previously (Estany et al., Reference Estany, Ros-Freixedes, Tor and Pena2017; Park et al., Reference Park, Beak, Jung, Kim, Jeong, Piao, Kang, Fassah, Na, Yoo and Baik2018), genetic background or breeds, management (e.g. early weaning, castration and prolonged feeding) can effectively increase IMF percentage and marbling score. Here, we only focus on nutritional manipulations targeting intramuscular FAPs (Figure 2).
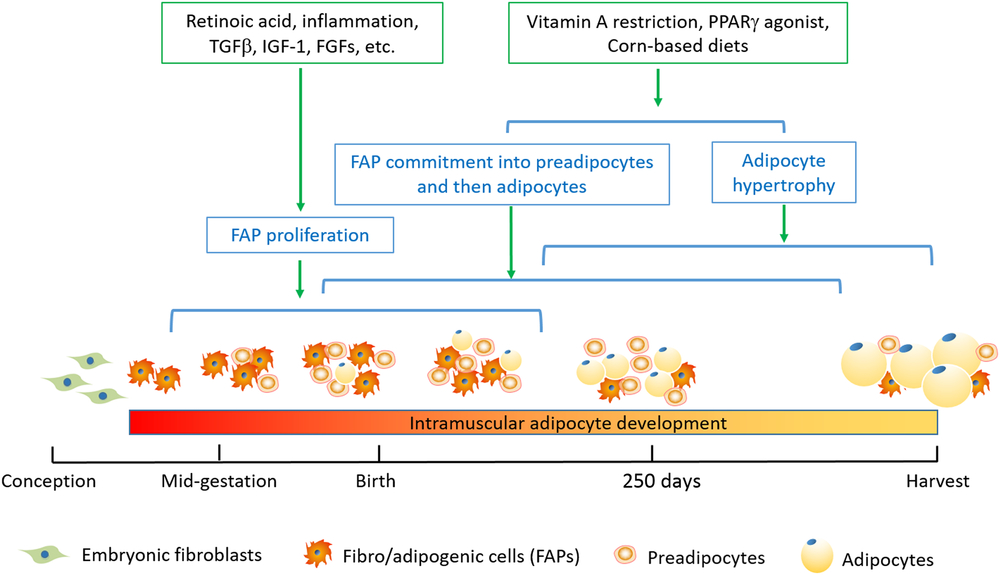
Figure 2 Timeline for nutritional strategies to enhance intramuscular FAPs and their differentiation into adipocytes in beef cattle. The stages including FAP proliferation, FAP commitment into preadipocytes and then adipocytes, and adipocyte hypertrophy are not mutually exclusive; instead, these processes represent progressive changes. Because of the conservativeness of adipose tissue development, it should be applicable to other species. However, due to the difference in maturity of animals at birth and at harvest, the timeline needs to be adjusted accordingly. FAPs, fibro-adipogenic progenitors; FGFs, fibroblast growth factors; IGF-1, insulin-like growth factor 1; PPARγ, peroxisome proliferator activated receptor γ; TGFβ, transforming growth factor β.
Prenatal nutrition
The fetal and neonatal stages are most effective in promoting FAP proliferation and intramuscular adipocyte formation (Du et al., Reference Du, Huang, Das, Yang, Duarte, Dodson and Zhu2013 and Reference Du, Wang, Fu, Yang and Zhu2015). Through binding to retinoic acid receptors, retinoid acids are required for adipogenesis. We recently found that maternal supplement of vitamin A or retinoid acid expands FAP population in mice (Wang et al., Reference Wang, Fu, Liang, Wang, Yang, Zou, Nie, Zhao, Gao, Zhu, de Avila, Maricelli, Rodgers and Du2017), and injection of vitamin A at birth and 1 month of age promotes intramuscular fat development in Angus beef cattle (Harris et al., Reference Harris, Wang, Deavila, Busboom, Maquivar, Parish, McCann, Nelson and Du2018). Because vitamin A deficiency in beef cattle occurs during the dry season when β-carotenoid content in forage becomes very limited, vitamin A supplementation at fetal and newborn stage provides a feasible strategy to increase intramuscular adipogenesis and marbling fat development in beef cattle (Kruk et al., Reference Kruk, Bottema, Reyes-Veliz, Forder, Pitchford and Bottema2018).
It is widely accepted that maternal nutrition affects adipose tissue and skeletal muscle development in lamb (Zhu et al., Reference Zhu, Ford, Means, Hess, Nathanielsz and Du2006), beef cattle (Robinson et al., Reference Robinson, Cafe and Greenwood2013) and pigs (Oksbjerg et al., Reference Oksbjerg, Nissen, Therkildsen, Møller, Larsen, Andersen and Young2013). Maternal nutrient deficiency leads to overall increase in offspring fatness when fed with a high-energy diet (Dandrea et al., Reference Dandrea, Wilson, Gopalakrishnan, Heasman, Budge, Stephenson and Symonds2001; Zhu et al., Reference Zhu, Ford, Means, Hess, Nathanielsz and Du2006; Symonds et al., Reference Symonds, Pope, Sharkey and Budge2012), likely due to the adipocyte hypertrophy. On the other hand, maternal over-nutrition promotes intramuscular adipogenesis. Overfeeding ewes leads to a higher density of intramuscular adipocytes in fetal (Yan et al., Reference Yan, Zhu, Xu, Tong, Ford, Nathanielsz and Du2010) and adult lambs (Yan et al., Reference Yan, Huang, Zhao, Long, Uthlaut, Zhu, Ford, Nathanielsz and Du2011), accompanied with elevated collagen accumulation (Huang et al., Reference Huang, Zhao, Yan, Zhu, Long, McCormick, Ford, Nathanielsz and Du2012b), indicating that maternal over-nutrition might increase the number of intramuscular FAPs.
Postnatal nutrition
Intramuscular adipogenesis occurs at a later stage compared to other fat depots (Albrecht et al., Reference Albrecht, Kuzinski, Komolka, Gotoh and Maak2015). Postnatal hyperplasia of intramuscular preadipocytes also plays an important role in marbling formation (Albrecht et al., Reference Albrecht, Teuscher, Ender and Wegner2006), and individuals with a high capacity to create more preadipocytes within muscle are recommended in cattle breeding (Harper and Pethick, Reference Harper and Pethick2004). Supplementation of CLA during the fattening stage increases IMF accumulation while decreases subcutaneous deposition in pigs (Wiegand et al., Reference Wiegand, Sparks, Parrish and Zimmerman2002) and cattle (Zhang et al., Reference Zhang, Dong, Wang, Zhou, Peng, Zou, Xue and Wang2016). Possible interpretation is that CLAs promote the development of preadipocytes in intramuscular SVF cells, which contain FAPs (Meadus et al., Reference Meadus, MacInnis and Dugan2002), while limiting the adipogenic differentiation of subcutaneous SVF cells (Zhou et al., Reference Zhou, Li, Yin, Ni, Dong, Zhang and Du2007). Vitamin A restriction for 10 months during the finishing state of steers greatly increased IMF, possibly through enhanced proliferation of intramuscular preadipocytes, expanding the pool of intramuscular adipocytes; such effect was not observed in other fat in subcutaneous depots likely due to its inability for adipocyte expansion (Kruk et al., Reference Kruk, Bottema, Reyes-Veliz, Forder, Pitchford and Bottema2018).
Other promising strategies
Peroxisome proliferator activated receptor γ (PPARγ), a ligand-dependent transcription factor, is a master regulator of adipogenesis, and its agonists (rosiglitazone, thiazolidinedione, pioglitazone and others) are generally effective in treating metabolic dysfunction and diabetes (Ma et al., Reference Ma, Wang, Zhao and Xu2018a). Excitingly, PPARγ agonists promote IMF deposition while improving insulin sensitivity in type 2 diabetes (Mayerson et al., Reference Mayerson, Hundal, Dufour, Lebon, Befroy, Cline, Enocksson, Inzucchi, Shulman and Petersen2002). Consistent results are observed in mice (Muurling et al., Reference Muurling, Mensink, Pijl, Romijn, Havekes and Voshol2003) and rats (Lessard et al., Reference Lessard, Giudice, Lau, Reid, Turner, Febbraio, Hawley and Watt2004). Similarly, dietary supplementation of thiazolidinedione (Chen et al., Reference Chen, Feng, Yang, Shu, Jiang and Wang2013) or pioglitazone hydrochloride (Jin et al., Reference Jin, Gao, Wang, Zhang, Xu, Li, Yan and Wang2018) noticeably promotes IMF accumulation in finishing pigs, without affecting backfat thickness. Further work showed that the activation of PPARγ specially enhance adipogenesis of porcine muscular SVFs (Li, Reference Li2018), likely due to the enrichment of FAPs in intramuscular fat compared to other fat pads. Thus, it is promising to seek chemicals or supplements as PPARγ agonists to specifically increase marbling.
Previous works propose that intramuscular adipocytes prefer glucose for de novo fatty acid synthesis (Smith and Crouse, Reference Smith and Crouse1984; Rhoades et al., Reference Rhoades, Sawyer, Chung, Schell, Lunt and Smith2007; Wang et al., Reference Wang, Zhou, Shu, Wang, Zhu, Gao, Xi, Zhang, Yuan and Jiang2013); thus dietary glucose can be used as a lipogenic substrate. Compared with the hay-based diet, corn-based diet can increase glucose uptake in intramuscular adipocytes, thus increasing IMF contents in America Wagyu (Chung et al., Reference Chung, Lunt, Kawachi, Yano and Smith2007) and Barrosã bulls, but not Alentejana bulls under the same condition (Costa et al., Reference Costa, Costa, Bessa, Lemos, Simões, Santos-Silva, Fontes and Prates2013). Conversely, hay-based diet in the finishing stage generates lower marbling in Jersey steers (Arnett et al., Reference Arnett, Fluharty, Loerch, Zerby, Zinn and Kuber2012).
Conclusion
Skeletal muscular PDGFRA+ FAPs are the major sources of intramuscular adipocytes, which provide a key target to promote intramuscular adipocyte development and marbling fat deposition. Consistently, the proliferative and adipogenic potential of muscular FAPs correlates with marbling fat deposition, and the density of FAPs differs among breeds with different IMF contents and marbling scores. Growing efforts focusing on expanding intramuscular FAP pool, their commitment to preadipocytes and final differentiation into mature adipocytes, through nutritional and pharmaceutical manipulations, have yielded promising results. Moreover, as progenitor cells with dual potency of adipogenesis and fibrogenesis, the differentiation fate of intramuscular FAPs is largely dependent on its niche environment, which warrants further investigation in order to enhance a pro-adipogenic niche.
Acknowledgements
This work is partially supported by National Key Research and Development Program of China (2018YFD0500402), National Natural Science Foundation (31501925), and grants from Agriculture and Food Research Initiative Competitive Grants (no. 2015-67015-23219 and 2016-68006-24634) from the USDA National Institute of Food and Agriculture. A scholarship from the Chinese Scholarship Council was awarded to XL.
X. Li 0000-0002-6630-0503
Declaration of interest
All authors declare no conflicts of interest.
Ethics statement
None.
Software and data repository resources
No new software or database was generated as part of the outcomes of this work.