INTRODUCTION
Dogs and cats are popularly kept for companionship in industrialized countries. In The Netherlands (population: 16·5 million), there were about 1·5 million dogs and 3 million cats in 2010, with 21% and 34% of households owning at least one dog or cat, respectively [1].
Ownership of dogs and cats (hereafter referred to as pets) is beneficial to the owner's psychological and physical health, promoting a less sedentary lifestyle, emotional protection and social interaction [Reference McNicholas2]. However, pets frequently enjoy great freedom in their owner's home, which may include access to their owner's bed [Reference Chomel and Sun3]. A recent survey in The Netherlands indicated that in 159 households with pets, 45% of dogs and 62% of cats were allowed on the bed [Reference Overgaauw4]. Half of the owners allowed the dogs to lick their face and 45% allowed the cats to jump onto the kitchen sink. This close interaction between pets and humans raises concerns regarding the potential zoonotic risks.
Campylobacteriosis, a frequently occurring foodborne infection in The Netherlands [Reference Havelaar5], is potentially transmissible between pets and humans via the faecal–oral route [Reference Marks6]. Owning a pet, especially a puppy, has been identified as a risk factor for Campylobacter jejuni and C. coli infection [Reference Doorduyn7]. Campylobacter may cause both symptomatic and asymptomatic infections in pets [Reference Marks6], with similar isolation rates in both diarrhoeic and healthy animals [Reference Rossi8, Reference Sandberg9]. Symptomatic infections mainly occur in young animals and are often caused by C. jejuni [Reference Marks6].
Although infection in pets and humans from a common source is possible, there is evidence for transmission between pets and humans from molecular studies of C. jejuni using amplified fragment length polymorphism [Reference Wolfs10], and pulsed-field gel electrophoresis [Reference Damborg11].
Multilocus sequence typing (MLST) [Reference Dingle12] is widely used for the purposes of source attribution of human Campylobacter infections [Reference McCarthy13–Reference Strachan17], but has not yet been used to investigate the genetic relatedness of strains from pets and their owners. In this study, we investigated MLST profiles of C. jejuni and C. coli strains isolated from pets and their owners. We also estimated the probability that these human strains originated from pets or other putative reservoirs by conducting a source-attribution analysis. Finally, we combined case-control and source-attribution data to explore risk factors at the point of exposure for human campylobacteriosis of probable pet origin.
METHODS
Epidemiological data
Data from the CaSa study, a large case-control study on risk factors for human campylobacteriosis and salmonellosis conducted in The Netherlands between April 2002 and April 2003, formed the basis of this study. A detailed description of the CaSa study is available elsewhere [Reference Doorduyn7, Reference Doorduyn18].
A total of 2858 C. jejuni and 257 C. coli laboratory-confirmed human cases were identified by the Dutch Regional Public Health Laboratories (RPHL) through passive surveillance of diarrhoeic patients seeking medical attention. Isolates were sent to the Central Veterinary Institute (CVI) in Lelystad, The Netherlands, for C. jejuni and C. coli species determination using molecular methods [Reference Fermér and Engvall19, Reference Marshall20]. Cases that did not return or successfully complete the questionnaire used to collect epidemiological information (1679 cases, 54%) and/or had a recent or unknown history of travel and/or lived abroad (338 cases, 11%), were excluded, leaving 1019 C. jejuni and 79 C. coli cases (35%) enrolled in the study. Isolates from 737 (67%) enrolled cases were typed using MLST [Reference Dingle12].
Controls were randomly selected from population registries within the RPHL service areas by frequency matching (aiming at two per case) according to the expected number of Campylobacter/Salmonella cases (based on historic surveillance data) by age (0–4, 5–17, 18–29, 30–44, 45–59, ⩾60 years), gender, degree of urbanization (urban >2500, urbanized 500–2500, rural <500 addresses/km2), and season (April–June 2002, July–September 2002, October–December 2002, January–March 2003). A total of 10 250 controls were approached and 3409 (33%) returned the questionnaire. After exclusion of controls that travelled abroad and/or did not successfully complete the questionnaire, 3119 (91%) controls were enrolled.
Cases and controls were asked to complete a questionnaire regarding food consumption, kitchen hygiene, contact with animals, occupation, recreational activity, medication use, history of chronic diseases, and contact with people with gastroenteritis. Questions covered the 7 days prior to symptom onset (cases) or questionnaire completion (controls). Parents completed the questionnaire for their children. Missing values were handled using multiple imputation [Reference Rubin21].
Cases owning a pet were asked to submit a faecal sample of their pets to be tested for Campylobacter spp. and typed with MLST if they were positive for C. jejuni or C. coli using the same methods as for human cases. Pet faecal samples were submitted by mail: an envelope was sent to the owners including a container without transport medium together with instructions for collecting and returning the material. A minimum amount of faeces was requested in order to minimize the die-off due to dry conditions. Samples were transported overnight and processed the following day. A total of 687 pet faecal samples were submitted. The sample origin (dog or cat) could be determined for only 424 (62%) samples (315 from dogs and 109 from cats) because this information was not always indicated by the submitting owner, nor could it be inferred from the patient's questionnaire because of cohabitation of dogs and cats in the same household. A median of 32 days [interquartile range (IQR) = 13, min–max 10–71] separated human and pet faecal sampling. Bionumerics software v. 5.10 was used to analyse the sequence data. The expected probability (P) of finding an identical sequence type (ST) x in a human case and a pet living in the same household randomly was calculated as:
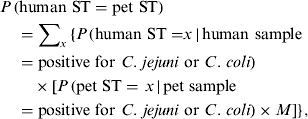
where ∑x is the summation over all STs found in both pets and humans and M is the overall prevalence of C. jejuni and C. coli found in our pet sample.
The proportional similarity index (PSI) [Reference Mullner15] was used to measure the similarity between ST frequency distribution of pets, pet owners, and non-pet owners. PSI values range between 1 (identical frequency distribution) and 0 (distributions with no common ST).
Attribution analysis
MLST data from Campylobacter strains obtained from chicken, cattle, sheep, pigs, pets, and the environment (water, sand, wild birds) were supplied by the CVI and supplemented with other data from the UK [Reference Dingle12], Scotland [Reference Strachan17], and Switzerland [Reference Korczak22] to provide a representative dataset for each reservoir (Table 1). As environmental strains have rarely been found in the other reservoirs [Reference Mullner15], they were treated as proxy for other unidentified reservoirs, putatively of wildlife origin [Reference Mullner15].
Table 1. Reference Campylobacter strains used to feed the asymmetric island model for source attribution

* Include isolates from dogs and cats.
† Provided by the Central Veterinary Institute (CVI) in Lelystad, The Netherlands.
The Asymmetric Island (AI) model, a Bayesian population genetics algorithm for modelling Campylobacter evolution and transmission [Reference Wilson14], was used to estimate the posterior probability (Pr) for each human ST to originate from the considered reservoirs. Two separate AI models were developed, one including and one excluding pets as reservoir. The overall proportion of human infections attributed to a given reservoir was calculated as the sum of its Pr over cases divided by the total number of cases.
Differences in Pr for pets (Pr p) were tested for the variables age, sex, degree of urbanization, season, and pet ownership using the Kruskal–Wallis or Mann–Whitney tests, as appropriate (α = 0·05).
Risk factor analysis
Risk factor analysis was restricted to human campylobacteriosis caused by STs of probable pet origin and to human campylobacteriosis as a whole (all 737 typed cases, non-specific to probable pet origin). Risk factors for campylobacteriosis as a whole and for campylobacteriosis caused by STs attributable to reservoirs other than pets have been reported previously [Reference Gras23].
STs were assigned to pets based on their Pr p. This was done in the same way as in previous studies [Reference Gras23–Reference Mullner25]: the Pr p distribution was assessed and a cut-off was determined to optimize the number of cases assigned to pets and the confidence as to their correct assignment derived by the highest possible Pr p. A logistic regression analysis was then conducted to investigate risk factors for human campylobacteriosis caused by STs with at least 60% probability (cut-off Pr p⩾0·60) of originating from pets. This cut-off Pr p resulted in the selection of 76 cases with a median Pr p of 0·62 (mean Pr p = 0·65, IQR = 0·08, min–max 0·60–0·74) and represented the best trade-off between the increasing Pr p (i.e. increase in pet specificity) and the decreasing number of cases eligible for inclusion in the analysis (i.e. decrease in statistical power and failure of the model to converge).
For preliminary significance testing, we assessed the association of 131 risk factors using a single-variable logistic regression analysis: variables with P⩽0·10 were selected for inclusion in a multivariable logistic regression model. A backward stepwise selection procedure was applied and variables with P < 0·05 were retained in the final model. The frequency-matched variables and level of education [Reference Doorduyn18] were always included as covariates.
Multivariable odds ratios (ORs) and corresponding 95% confidence intervals (CI) of risk factors for pet-associated human campylobacteriosis were presented together with those of a multivariable model built in the same fashion including all 737 typed cases that had been reported previously [Reference Gras23] and is presented here in abbreviated format to facilitate comparison.
The effect of the assignment cut-off Pr p value was checked by sensitivity analysis by repeating the analysis for a range of cut-off Pr p values, looking for significant changes in the risk factors in the reduced model. The low number of cases did not allow for the construction of models based on cut-off Pr p > 0·60. Overall model significance and goodness-of-fit were verified by likelihood ratio χ 2 and Hosmer–Lemeshow tests, respectively. Statistical analysis was performed using Stata v. 11.2 (StataCorp., USA).
RESULTS
Multilocus sequence types
Isolates from the 737 typed human cases were assigned to 154 STs (Fig. 1). Of the 687 pet faecal samples examined, 132 (19%) tested positive for Campylobacter spp. Campylobacter prevalence was 18% in samples specified of either dog or cat origin and 23% in the 263 samples of unspecified origin. A total of 499 isolates were recovered from the 132 positive samples (3·8 isolates per sample). Only 248 isolates had their origin specified (205 from dogs, 43 from cats).
Fig. 1. Sequence types identified in 737 Campylobacter jejuni and C. coli strains from human cases, subdivided by pet ownership. The category ‘others’ includes sequence types that occurred fewer than five times.
Overall, 320 (64%) isolates were C. upsaliensis and C. helveticus, 100 (20%) C. jejuni, 33 (7%) C. hyointestinalis and C. fetus, 10 (2%) C. lari, four (1%) C. coli, and 32 (6%) were unidentified. The 205 isolates from the dogs were: 158 (77%) C. upsaliensis and C. helveticus, 19 (9%) C. jejuni, 25 (12%) C. hyointestinalis and C. fetus, and three (2%) unidentified. The 43 strains from the cats were: 21 (49%) C. upsaliensis and C. helveticus, 21 (49%) C. jejuni, and one (2%) unidentified. The 104 C. jejuni and C. coli isolates from pets belonged to 49 STs, with ST45, ST403, and ST22 the most prevalent (>25%, Fig. 2); ST403 was the most prevalent in dogs (4/19 strains) and ST45 in cats (4/21 strains).
Fig. 2. Sequence types identified in 104 Campylobacter jejuni and C. coli strains from dogs and cats owned by people with C. jejuni and C. coli infections. The category ‘others’ includes sequence types that occurred once.
The PSI for STs of pet owners and non-pet owners (Fig. 1) was 0·69 (95% CI 0·50–0·88). PSI for STs of pets and pet owners was 0·39 (95% CI 0·27–0·51) and that for pets and non-pet owners was 0·33 (0·20–0·45). PSI for pets and dog owners was 0·43 (95% CI 0·26–0·60) and that for pets and puppy owners was 0·51 (95% CI 0·30–0·64).
In 68 cases, isolates were typed from both pets and patients living in the same household. Of these, two owners (2·94%) were infected with the same ST found in their pets. These were a 44-year-old man and his dog infected with ST45 and a 47-year-old man and his pet infected with ST658. Given the ST distribution in humans and pets and the overall prevalence of C. jejuni and C. coli in pets, the expected probability of finding an identical ST in humans and pets in a one-to-one relationship purely by chance was 0·198%, which corresponds to an expected 0·134 cases out of 68. The difference between the observed (n = 2) and expected («1) co-isolation of identical STs in humans and pets was statistically significant (binomial probability test, P = 0·008).
Attribution of human infections
The AI model without pets (Fig. 3) estimated that 77% (95% CI 75–79) of human infections originated from chicken, followed by cattle 18% (95% CI 16–19), the environment 2% (95% CI 1–3), sheep 2% (95% CI 1–3), and pigs 1% (95% CI 0·1–1). When pets were also included (Fig. 3), cases were attributed as follows: chicken 63% (95% CI 61–65), pets 25% (95% CI 23–26), cattle 11% (95% CI 9–11), pigs 0·5% (95% CI 0·1–0·8), sheep 0·4% (95% CI 0·3–0·5), and the environment 0·1% (95% CI 0·1–0·2).
Fig. 3. Assignment source probability (%) estimated by the Asymmetric Island model with and without pets represented as a matrix plot. Each human case is a vertical column with level of shading according to the probability that it came from each considered source. To aid visualization, cases are ordered horizontally according to the probability attributed to chicken.
STs predominantly associated with pets included in the risk factor analysis (Pr p ⩾ 0·60) were: ST403 (Pr p = 0·76), ST508 (0·74), ST586 (0·74), ST1326 (0·74), ST878 (0·73), ST3130 (0·72), ST1911 (0·70), ST2088 (0·67), ST47 (0·67), ST657 (0·63), ST2151 (0·62), ST2130 (0·61), ST122 (0·61), ST22 (0·61), and ST677 (0·60).
The average Pr p was significantly higher (P = 0·028) in dog owners (median Pr p = 0·25, IQR = 0·3, n = 221) compared to non-dog owners (median Pr p = 0·18, IQR = 0·23, n = 516), and this difference became more evident when considering those owning a puppy (median Pr p = 0·31, IQR = 0·43, n = 35, P = 0·001) or those owning both puppies and adult dogs (median Pr p = 0·49, IQR = 0·34, n = 12, P = 0·003).
Risk factors for pet-associated human campylobacteriosis
Several factors were significantly associated with C. jejuni and C. coli infection in the overall model (Table 2), including factors concerning contact with pets, such as ownership of several adult dogs and at least one puppy (OR 2·5, 95% CI 1·1–5·8), ownership of one or more cats (OR 1·4, 95% CI 1·2–1·8), and contact with dogs outside the household (OR 0·6, 95% CI 0·5–0·8). However, only three risk factors (two of which related to contact with pets) were significant in the model for campylobacteriosis of probable pet origin (Table 2). These were: (1) ownership of a puppy (OR 3·7, 95% CI 1·4–10·0), (2) ownership of several adult dogs and at least one puppy (OR 9·2, 95% CI 2·7–32·0), and (3) recent use of proton-pump inhibitors (OR 11·1, 95% CI 5·4–22·9). The first risk factor was unidentified in the overall model. No significant interactions of these risk factors with age, gender, degree of urbanization, season, or level of education were found.
Table 2. Risk factors for human Campylobacter jejuni/coli infection in general (overall model) and for infection caused by C. jejuni/coli strains of pet origin (pet model) as assigned by the Asymmetric Island model for source attribution
OR, Odds ratio; CI, confidence intervals; n.s., not significant (P > 0·05).
Bold values indicate the risk factors; protective factors are in normal font.
* % of imputed missing values in the whole dataset.
† Adjusted for age, sex, degree of urbanization, season, and level of education.
‡ n = 3856 (737 cases and 3119 controls).
§ n = 3195 (76 cases and 3119 controls).
DISCUSSION
This study shows that there is a high degree of overlap between human and pet C. jejuni and C. coli STs and that dog owners, especially puppy owners, are at higher risk for infection with STs associated with pets than controls and non-dog owners. Furthermore, there were 2/68 cases where a patient and a pet living in the same household were infected with an identical ST. Although this may seem a minor proportion, it is considerable if we reflect on both the adopted sampling scheme (particularly the relatively lengthy time lag between human and pet sampling) and the low occurrence of severe, symptomatic campylobacteriosis usually detectable by passive surveillance. Moreover, C. jejuni carriage in pets is infrequent and of short term [Reference Hald26]. Accordingly, the expected occurrence of identical STs in a one-to-one relationship was significantly lower than that observed. Furthermore, although the 95% CIs of the PSI were largely overlapping, the point estimates showed a trend towards similarity of pet and human ST frequency distribution according to pet ownership. Taken together these results suggest that dog ownership significantly increases the risk of acquiring C. jejuni and C. coli infection caused by STs originating from pets and that co-isolation of identical strains in humans and their pets occurs significantly more often than expected by chance.
There are four possible scenarios arising from our findings: (1) humans and pets become infected from the same source, (2) humans and pets become infected from different sources that incidentally carry the same strain, (3) humans become infected from dogs, (4) dogs become infected from humans. While the second scenario seems unlikely because of the large variety of existing STs, there may be many common sources of infection for pets and humans [Reference Parsons27]. This is mainly because pet foods and treats contain ingredients of animal origin. Moreover, pets are increasingly regarded as real family members and tend to be fed with the same foods as their owners [Reference Schlesinger and Joffe28]. Feeding of a homemade diet or table and kitchen food scraps, especially raw meats, offal, and bones, is a risk factor for Campylobacter carriage in pets [Reference Wieland29–Reference Leonard31], and pets carrying Campylobacter may eventually act as reservoirs for transmission to humans either directly or by contaminating the household and the immediate environment [Reference Parsons27, Reference Schlesinger and Joffe28]. As the sampling design was non-directional in the transmission of infection, our results support evidence for genetic association of C. jejuni and C. coli strains between humans and their pets but do not prove that transmission of such strains occurs from pets to humans or vice versa.
The contribution of pet ownership to human infections, as derived from case-control studies, appears to not exceed 10% [Reference Doorduyn7]; thus, the pet attribution found in this study (25%) seems to be overestimated. Presumably, this is an artefact of the attribution process, as pets are not the main reservoir of STs found in pets and humans. The AI model could therefore have attributed many cases to the pets themselves instead of the common reservoirs. When including pets in the AI model, cases attributable to chicken decreased measurably (–14%), followed by cattle (–7%), sheep (–2%), and the environment (–2%). The extent of this decrease is suggestive of the reservoirs from which pets may acquire infection in parallel with humans. Overall, figures provided by the AI model without pets agree with those of similar studies [Reference McCarthy13–Reference Strachan17].
We found that ownership of dogs, particularly puppies and several adult dogs, became a predominant risk factor when considering the cases with a high Pr p. Campylobacter prevalence is higher in puppies and adult dogs housed in groups, possibly due to low levels of acquired immunity and dog-to-dog transmission, respectively [Reference Marks6, Reference Acke32]. Moreover, while puppies are usually housed indoors and have closer contacts with their owners, adult dogs living in groups are likely to have (unsupervised) outdoor access and act as vectors for environmental strains [Reference Gras23], especially if they have access to fields grazed by livestock or wildlife [Reference Parsons27]. Eventually, owners walking their dogs may be particularly exposed to such environmental strains. While dog ownership increases the risk for pet-associated infections, contact with dogs outside the household appears to be protective. A possible explanation is that contact with dogs other than their own encourages individuals to undertake protective actions such as hand washing [Reference Gras23].
In this study, cat ownership, unlike dog ownership, was not a significant risk factor for the most pet-associated STs. This is in accordance with canine behaviour that generally results in frequent soil and water contact, whereas cats usually hide and bury their faeces and lick their fur intensively, making it easier to remove any possible Campylobacter-contaminated matter [Reference Overgaauw4].
It has been shown that individuals acquiring C. jejuni and C. coli infection from different reservoirs have different associated risk factors [Reference Gras23, Reference Bessell24]. Modelling of MLST data is useful for determining where infection is likely to be acquired from. However, most cases cannot be attributed to a single reservoir; only those cases infected with rare STs, i.e. occurring 1–5 times on average [Reference Gras23], usually show a high Pr (i.e. ⩾0·5) for a given reservoir and possess unique risk factors associated with that reservoir. Also in this study, ST reservoir specificity and rareness appear to correlate, as the Pr p-based selection led to 76 cases belonging to 15 different STs (five cases per ST). Common STs are difficult to disentangle and assign to specific reservoirs because they could potentially originate from several reservoirs simultaneously. Moreover, the high Pr for a given reservoir may change over time: new STs may emerge at low frequencies in specific reservoirs (i.e. high Pr for a reservoir) and then either disappear or become increasingly widespread (i.e. an almost equal Pr for different reservoirs).
ST45 and ST658 were co-isolated in pets and their owners. Pets corresponded to the first and second most likely reservoir for ST658 (Pr p = 0·59) and ST45 (0·31), respectively. The globally widespread ST45 usually predominates in chicken [Reference Dingle12, Reference Mullner15, Reference Strachan17, Reference Kärenlampi33–Reference Schouls35], as also indicated by our AI model with pets (Pr for chicken = 0·62). However, in our study and in another from the UK [Reference Parsons27], ST45 was the most frequent ST in pets and was associated with contact with pets in Finland [Reference Kärenlampi33], suggesting that its circulation in pets is extensive. Furthermore, as ST45 was overrepresented in surface water [Reference Sopwith36], and open drains/pools have been associated with Campylobacter carriage in dogs [Reference Wieland29], it has been hypothesized that ST45 is an environmentally adapted ST that mainly infects humans through transmission pathways other than food, including contact with pets [Reference Parsons30, Reference Sopwith36].
In this study, the overall proportion of Campylobacter-positive samples in pets (19%) was relatively low compared to previous figures from The Netherlands (77%) based upon 22 healthy and eight diarrhoeic household dogs aged between 3 months and 14 years [Reference Koene37]. In other studies [Reference Sandberg9, Reference Wieland29–Reference Leonard31], Campylobacter prevalence ranged between 15% and 76% for dogs, and 11% and 43% for cats, but can be as high as 87% and 75% in kennelled dogs and cats, respectively [Reference Acke32]. This variability is due to differences in age, sex, breed, diet, and housing of the examined animals, but also to the different management of faecal samples. The delivery of pet samples by mail could have affected the survival of the most fragile strains, possibly skewing our sample towards the most resistant ones.
We also found a relatively large proportion of C. upsaliensis and C. helveticus (64%). In a Danish longitudinal study in which 366 faecal samples from 26 household dogs were also sent by mail [Reference Hald26], 76% were Campylobacter-positive, 75% of which were C. upsaliensis and 19% C. jejuni. The Danish study included only puppies tested monthly up to age 2 years, a factor that may explain the higher overall isolation rate. However, the dog and cat Campylobacter species distribution we found is comparable with other reports [Reference Wieland29–Reference Leonard31, Reference Koene37], suggesting that receiving pet samples by mail did not bias the Campylobacter species distribution.
In conclusion, we compared C. jejuni and C. coli strains from pets and their owners using MLST and investigated risk factors for campylobacteriosis of probable pet origin by combining source-attribution and case-control data. Although pets and humans share many sources of infection and directionality of transmission between humans and pets could not be inferred, the combined analysis and the co-isolation of identical STs in pets and their owners suggest that dog, and particularly puppy, ownership is a risk factor for C. jejuni and C. coli infection caused by STs of probable pet origin and that co-isolation of identical strains in humans and their pets occurs more frequently than expected. This evidence could perhaps have been even stronger if the time lag between the two samples had been shorter.
Attributing human infections to pets may be deceptive when the goal is to identify the original reservoirs, as pets may artificially account for an abnormal amount of cases because they are, like humans, predominantly ‘final’ hosts for C. jejuni and C. coli. Conversely, the contribution of the other reservoirs will be underestimated and probably biased towards those reservoirs from which pets acquire infection.
It is unclear to what extent the increased risk of pet-associated campylobacteriosis in dog owners that we found is an indication of other unmeasured factors, such as owner's personality traits, lifestyle, income, disability or other health problems, which can plausibly influence the chance of becoming infected and the decision and manner of owning a pet. The zoonotic risk posed by pets should therefore be put into context, depending on factors such as level of Campylobacter carriage and intensity and type of contact between pets and humans.
Besides the previously identified risk factors for C. jejuni and C. coli infection [Reference Doorduyn7], there are different risk factors depending upon the attributable reservoir [Reference Gras23]. In this study, we explored risk factors for infection with STs attributable to pets, a poorly characterized reservoir of human campylobacteriosis. This analysis provided insight into reservoir-specific risk factors and transmission pathways for human campylobacteriosis, allowing for a better characterization of the zoonotic risk posed by pets. By enhancing our ability to characterize this zoonotic risk, public health initiatives can be better informed.
ACKNOWLEDGEMENTS
We thank the Dutch Ministry of Economic Affairs, Agriculture and Innovation, and the Dutch Ministry of Public Health, Welfare and Sport for research funding. We are grateful to M. A. S. de Wit and Y. Doorduyn for their contribution in designing the study, to the staff of the Veterinary Microbiological Diagnostic Laboratory of the Faculty of Veterinary Medicine of Utrecht University, for primary isolations of the pet samples, and to the laboratory staff of the Central Veterinary Institute, especially A. de Boer and E. Pothoven, for performing microbiological analyses and taking care of the strain collection. We also thank the participating Regional Public Health Laboratories for their contribution to the data collection, especially F. Vlaspolder, J. H. Sloos, J. Spaargaren, J. Peereboom, M. A. Schouten, R. W. Brimicombe, F. W. Sebens, H. Rothbarth, L. J. M. Sabbe, H. Mulder, D. Veenendaal, E. Ijzerman, J. H. T. Wagenvoort, J. H. van Zeijl, B. M. de Jongh, M. Tersmette, P. Voorn, A. M. Horrevorts, J. Buitenwerf, B. G. A. Hendrickx, M. Peeters and A. R. Jansz. Thanks are also extended to H. C. Boshuizen for help in multiple imputation method.
DECLARATION OF INTEREST
None.