INTRODUCTION
In recent years the Cape south coast ichnology project has focused on the description of tracks and traces on paleosurfaces in Late Pleistocene aeolianite and cemented foreshore deposits, representing the remains of dune, beach, and lagoon environments. The majority of tracks represent large mammals, birds, and reptiles, although the two former groups also include traces of smaller animals, inferred to represent mongoose and small avians (Helm et al., Reference Helm, Cawthra, Cowling, De Vynck, Marean, McCrea and Rust2018, Reference Helm, Cawthra, Cowling, De Vynck, Lockley, Marean, Thesen, Venter, Cleghorn, Potts and Cawthra2020a, Reference Helm, Lockley, Cawthra, De Vynck, Helm and Thesenb). Golden mole burrows were recently reported (Lockley et al., Reference Lockley, Helm, Cawthra, De Vynck and Perrin2021), and coprolites (including those of a small carnivore) offer further avenues of study to be reported elsewhere. Invertebrate traces are commonplace, but have not been formally described, and no inventory of invertebrate sites has yet been compiled.
While this trace fossil record does not suffer from the biases inherent in the regional body fossil record (which represents mostly hunted and scavenged prey in caves and rock shelters, by agents such as hominins, hyenas, porcupines, and owls), it has its own set of biases. Larger, heavier animals create larger, deeper tracks, which are easier to identify on Pleistocene paleosurfaces upon re-exposure, even when these surfaces exhibit substantial erosion (e.g., Helm et al., Reference Helm, Lockley, Moolman, Cawthra, De Vynck, Dixon, Stear and Thesen2021a). In addition, dune and beach sands do not provide ideal substrates for track registration and preservation, compared with finer-grained and more cohesive sediments.
Haubold (Reference Haubold1996, p. 23) introduced the term ‘phantom taxa’ to refer to ichnotaxa based on “fragmentary tracks, incomplete trackways and other preservational variations of optimal track morphology and trackway pattern.” Lucas (Reference Lucas2001) also reminded us that “the concept of extramorphological variation [was] introduced by Peabody (Reference Peabody1948) who noted that extramorphologic characters include characters arising from the type of recording material.” Thus, Lucas coined the term “taphotaxon” to encompass what Peabody termed extramorphological characters. This allows us to distinguish suboptimal preservation of footprint ichnotaxa under the concept of taphotaxa (see Haubold, Reference Haubold1997, and Lockley, Reference Lockley2000, for review of relevant concepts).
Recently, ichnologists have expressed concerns about the “morphological quality of preservation” of tracks and how it may affect ichnotaxonomy and ichnological interpretation more generally (Belvedere and Farlow, Reference Belvedere, Farlow, Falkingham, Marty and Richter2016; Gatesy and Falkingham, Reference Gatesy and Falkingham2017; Marchetti et al., Reference Marchetti, Belvedere, Voigt, Klein, Castanra, Díaz-Martínez and Marty2019a). In this regard, tracks on Pleistocene paleosurfaces on the Cape south coast seldom rise above level 2 on the four-point (0-1-2-3) quality-of-preservation scale developed by Belvedere and Farlow (Reference Belvedere, Farlow, Falkingham, Marty and Richter2016). Factors that lead to sub-optimal track preservation include sediment consolidation, moisture and microbe content, substrate grain size, and the effects of erosion and weathering in a coastal setting, which inevitably lead to biases against the identification of smaller, lighter trackmakers.
Nonetheless, under favorable circumstances, Pleistocene paleosurfaces on the Cape south coast have the capacity to preserve relatively fine detail, and thorough examination under optimal lighting conditions allows the identification of tracks and traces of smaller creatures. Two such examples are described here—one, a small-mammal trackway (with associated burrows), and the other, an invertebrate traceway. The tracksites were identified in 2019, and were situated ~140 m apart on loose aeolianite slabs on a remote stretch of coastline east of Still Bay.
GEOLOGICAL CONTEXT
Aeolianites, or cemented paleodune deposits, are distributed globally, generally in mid-latitude regions between 20° and 40° (Fairbridge and Johnson, Reference Fairbridge, Johnson, Fairbridge and Bourgeois1978; Brooke, Reference Brooke2001). Late Pleistocene examples of this rock form are well preserved along the Cape south coast of South Africa, and form the Waenhuiskrans Formation (Malan, Reference Malan1989). Late Pleistocene shoreface, foreshore, and lagoonal deposits of the Klein Brak Formation (Malan, Reference Malan1991) are also preserved along this coastline, but have not been encountered in the immediate area described herein. These two formations form part of the Bredasdorp Group.
Through the ongoing, multidisciplinary Cape south coast ichnology project (initiated in 2007), >300 tracksites have been identified in the study area between the town of Arniston in the west and the Robberg peninsula in the east, a distance of 350 km (Fig. 1). Numerous sites within this area have been dated through Optically Stimulated Luminescence, with the majority being from Marine Isotope Stage (MIS) 5 and MIS 6 (Roberts et al., Reference Roberts, Bateman, Murray-Wallace, Carr and Holmes2008; Carr et al., Reference Carr, Bateman, Roberts, Murray-Wallace, Jacobs and Holmes2010; Bateman et al., Reference Bateman, Carr, Dunajko, Holmes, Roberts, McLaren, Bryant, Marker and Murray-Wallace2011; Cawthra et al., Reference Cawthra, Jacobs, Compton, Fisher, Karkanas and Marean2018). MIS 11 deposits (Roberts et al., Reference Roberts, Karkanas, Jacobs, Marean and Roberts2012) and MIS 3 deposits (Carr et al., Reference Carr, Bateman, Cawthra and Sealy2019) are less common. The tracksite frequency has been explained by Roberts and Cole (Reference Roberts and Cole2003) as a result of four processes: (1) moist sand provided a cohesive molding agent; (2) high sedimentation rates enabled rapid track burial; (3) rapid lithification followed via partial solution and re-precipitation of bioclasts; and (4) shoreline erosion has re-exposed the track-bearing surfaces. In addition, we infer some binding of sand by microbial activity.

Figure 1. Locality map showing Bredasdorp Group sediments on the Cape south coast, and, in the bottom inset, the study site east of Still Bay.
East of Still Bay, a remote, rugged section of coastline, 6 km in length, comprises continuous cliffs as much as 70 m in height. Here, Pleistocene dune cordons are overlain by active Holocene dunefields of the Strandveld Formation. Planar cross-bedded units ranging in thickness from 0.4–18 m form the dominant primary sedimentary structure (Roberts et al., Reference Roberts, Bateman, Murray-Wallace, Carr and Holmes2008). The large-scale units are laterally persistent for tens of meters. Foreset dips of up to 37°, the angle of repose of wind-blown sand, are present. Foreset progradation of large-scale dunes has led to sedimentary facies that are dominated by large-scale planar cross-stratification. The foreset orientation indicates a dominance of westerly winds, broadly similar to present-day wind conditions (Roberts et al., Reference Roberts, Bateman, Murray-Wallace, Carr and Holmes2008).
Storm surges and high spring tides contribute to cliff collapse. Loose slabs and blocks are dislodged, and tumble down to lie at the base of the cliffs, at or below the high tide mark, where they are subjected to further wave and wind erosion. Over time, many of these loose blocks slump into the sea.
Roberts et al. (Reference Roberts, Bateman, Murray-Wallace, Carr and Holmes2008) first drew attention to this area, describing fossil elephant trackways and tracks in the Waenhuiskrans Formation. Roberts et al. (Reference Roberts, Bateman, Murray-Wallace, Carr and Holmes2008) presented OSL dates for the main elephant tracksite, which is situated ~750 m east of the two sites described here: a result of 140 ± 8.3 ka was obtained for the oldest dated unit, and a result of 91 ± 4.6 ka was obtained for the youngest dated unit. This currently remains the only reliably dated sequence on this stretch of coast. Although paleosols at the dated sequence are laterally persistent, permitting stratigraphic correlation to one nearby tracksite (Helm et al., Reference Helm, Cawthra, Cowling, De Vynck, Marean, McCrea and Rust2018), they do not extend west as far as the sites described here. Nonetheless, the expectation is that these cliffs represent an age range of deposits from MIS 6 to MIS 5b.
This stretch of coastal cliffs forms a zone of concentration of tracksites and, to date, 80 vertebrate tracksites have been identified here. Some of these have led to important paleoenvironmental inferences (Helm et al., Reference Helm, Cawthra, Cowling, De Vynck, Marean, McCrea and Rust2018, Reference Helm, Cawthra, De Vynck, Lockley, McCrea and Venter2019a; Lockley et al., Reference Lockley, Cawthra, De Vynck, Helm, McCrea and Nel2019, Reference Lockley2021) and paleoanthropological implications (Helm et al., Reference Helm, Cawthra, De Vynck, Helm, Rust and Stear2019b, Reference Helm, Cawthra, De Vynck, Helm, Rust and Stear2021b). The two sites described here would have been situated at the edge of the Palaeo-Agulhas Plain, most of which is currently submerged. Substantial changes in Pleistocene climates led to glacio-eustatic sea-level oscillations, with maximum regressions exposing the entire Plain (Marean et al., Reference Marean, Cowling and Franklin2020).
METHODS
Global Positioning System readings were taken, using a hand-held device. Locality data was reposited with the African Centre for Coastal Palaeoscience at Nelson Mandela University, South Africa, to be made available to bona fide researchers upon request. Tracksites were interpreted in the field through correlation to dated deposits and examination of cliff sections above the sites. Access to the tracksites was only possible during spring low tides.
Measurements of the trackways and burrows included length, width, and depth, and, where plausible, pace length. Thicknesses of foresets were measured. Results were recorded in centimeters. Tracings were made on clear acetate film. In addition to photographs that were taken of the tracks, traces, and and burrows described here, photographs were also taken of a Cape gerbil (Gerbilliscus afra) making tracks after release from a Sherman trap, and of bounding gait patterns in snow in British Columbia, Canada.
RESULTS
Small-mammal trackway and burrows
The small-mammal trackway is preserved in epirelief on a loose, north-facing aeolianite slab at the high tide mark. The precise origin in the cliffs above cannot be reliably determined. The mid-portion of the trackway contains the best-preserved track impressions, whereas the surface appears more eroded, with loss of track detail at either end. The trackway is interpreted as comprising 12 sets of relatively evenly spaced tracks, most of them in pairs (Fig. 2).
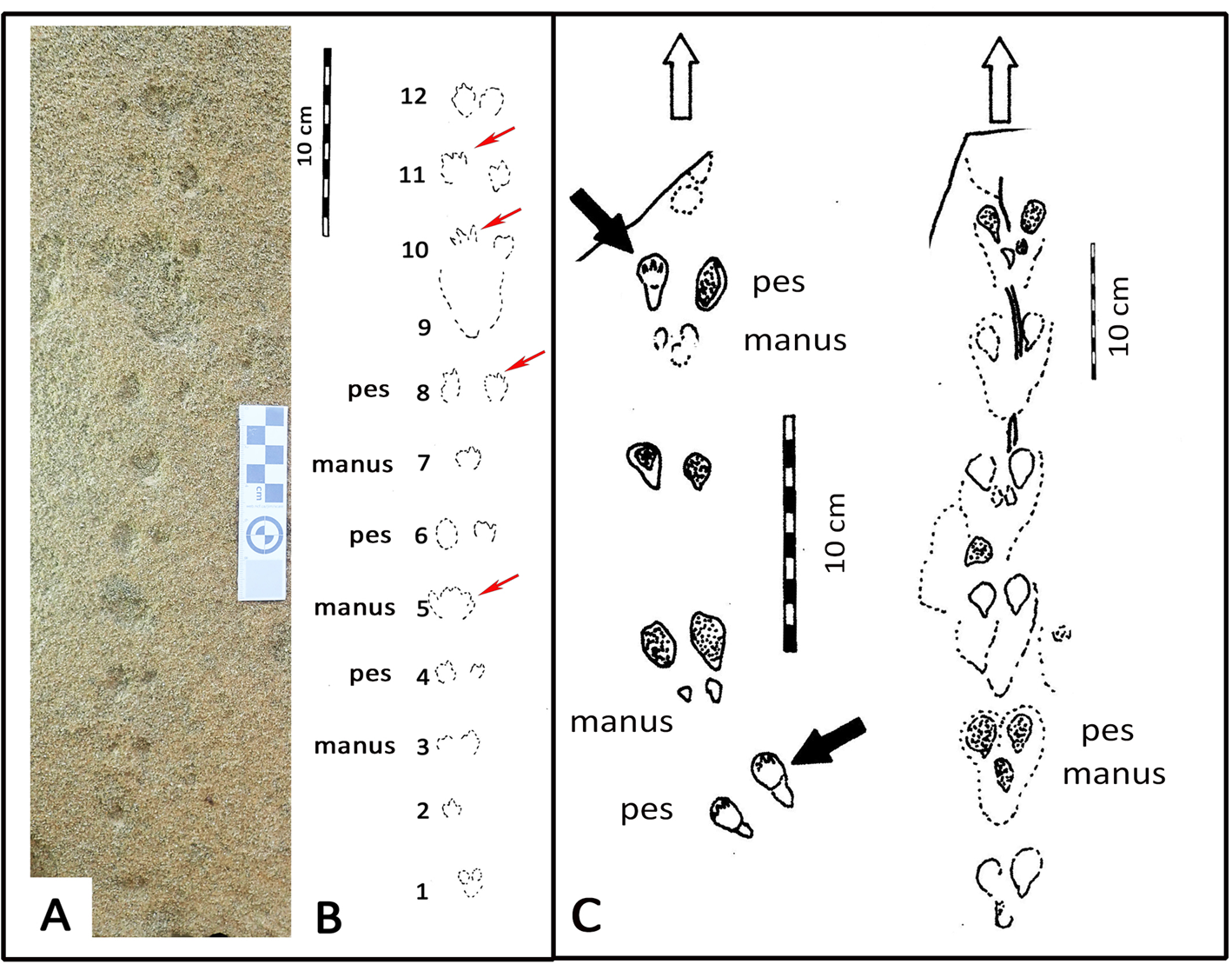
Figure 2. Photograph (A) and line drawing (B) of small-mammal trackway from the Waenhuiskrans Formation, Cape south coast, showing alternating manus and pes sets. Red arrows point to features inferred to represent faint digit tip traces that help orient the trackway. (C) Two trackways of Musaltipes from the Miocene Browns Park Formation, Colorado, also showing manus-pes sets (Lockley et al., Reference Lockley, Culver and Wegweisser2007; Lockley and Milner, Reference Lockley and Milner2014). Heavy black arrows indicate pes tracks with toe traces. White arrows show direction of progression.
The total trackway length is 43 cm, and is symmetrical about the mid line (i.e., the tracks occur as pairs indicating registration of a hopping or bounding gait rather than a series of alternating left and right footprints). The maximum straddle (measured from the most lateral margins of the tracks) is ~4.0 cm, whereas the average straddle is less (~3.5 cm). Dimensions for the best-preserved tracks that occur in non-overlapping pairs are ~1.5 cm long, and ~1.0 cm wide (i.e., the long axis is oriented parallel to the trackway mid-line). The individual tracks exhibit an oval configuration, and bilateral symmetry. Some tracks appear to have poorly preserved anteriorly situated toe traces. In some cases, in the mid-portion of the trackway, a single larger impression is apparent in the midline, rather than two separate impressions. No tail-drag impressions are apparent.
If the 12 pairs of tracks were interpreted as a series of 11 hind foot hops, then the mean length of each hop would be ~3.9 cm. However, if the discernable pattern of alternating widely and closely spaced tracks represents alternating manus and pes traces, as we infer (see Discussion), then the closely spaced pairs (e.g., 5, 7, and 9 in Fig. 2B) represent manus traces that are not discernably separated under the quality of preservation observed here, and the mean distance between corresponding points of these larger impressions is ~7.5 cm. Therefore, the trackway configuration appears to reflect the characteristic reduced inter-track spacing between the manus impressions of a bounding gait compared with the wider spacing of pes tracks. Post-registration erosion of the tracks may contribute to the overlapping appearance of individual tracks. The present state of preservation may reflect the influence of both gait and erosion factors (see Discussion). By 2021, detail on the track-bearing surface had deteriorated significantly due to erosion.
An adjacent large block, with poorly defined bedding, contains a variety of infaunal burrows, ~5 cm in diameter and as much as 50 cm in length (Fig. 3). Two loose slabs lie beside each other close to the high-tide mark, 310 m farther to the east of the trackway site. Branching burrows, ~4 cm in diameter, with maximum length of 80 cm, form a complex pattern on the surface of the western slab. Several of these traces lead to the base of an unusual, raised feature that measured ~25 × 20 cm, with a height of ~12 cm (Fig. 4). Within months of its identification, this raised feature had partly disintegrated. On the eastern slab, similar burrows are exposed on the same layer. Part of this slab contains overlying layers exhibiting parallel bedding, and the burrow-containing bedding plane can be followed around the side of the slab, where burrows are evident in profile, to where another raised feature can be viewed in profile.
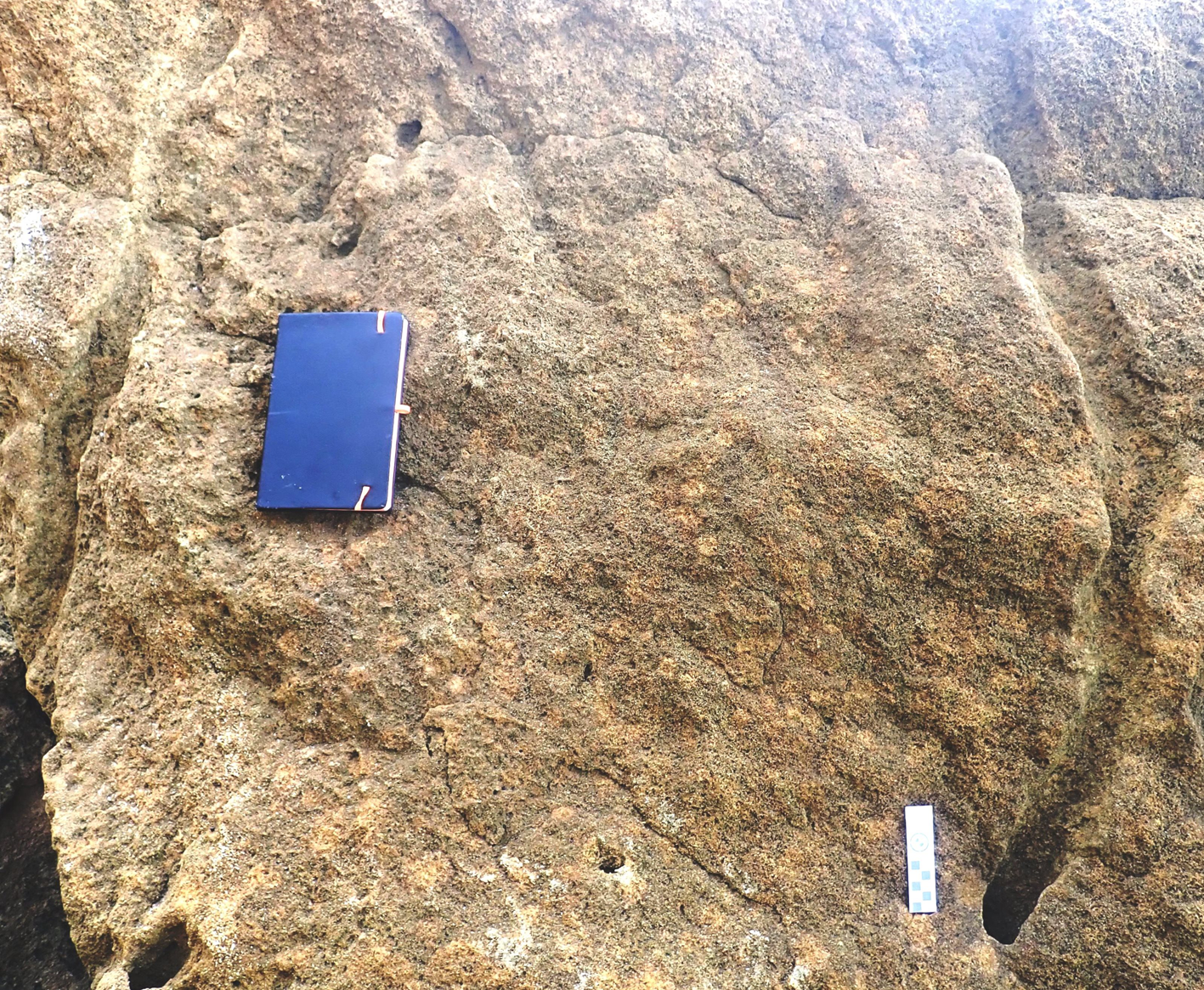
Figure 3. Burrows on a rock surface adjacent to the small-mammal tracksite.

Figure 4. Branching burrow traces on a surface 310 m to the east of the small-mammal tracksite, leading to an unusual raised feature that is interpreted here as a debris mound at the entrance to Cape gerbil burrows.
Arthropod traceway
The term ‘arthropod traceway’ is used here to describe a single, surface traceway, ~25 cm long and between ~3.0 cm and ~4.5 cm wide (Fig. 5). The traceway has a morphological configuration that invites comparison with the bilaterally asymmetric arachnid (spider) ichnogenus Octopodichnus, which originally was described from Permian sand-dune facies by Gilmore (Reference Gilmore1927). This configuration includes a repetition of a distinctive quadripartite set of four regularly spaced traces (footprints) on each side (right and left) of the traceway. Each set of four traces in the case of the traceway described here is between 5.5 cm and 5.8 cm long, parallel to the traceway axis, and the spacing between corresponding points in consecutive sets (strides) is ~9.0 cm. The traceway described here shows this regular configuration of quadripartite sets more clearly on one side than the other. The individual traces are round to oval and ~1.0 cm in diameter.

Figure 5. Photo (A) and line drawing (B) of presumed arachnid (spider) traceway from Pleistocene dune facies of the Cape south coast. Repeat pattern of quadripartite sets (red boxes) suggest a spider and identification of the traceway as Octopodichnus. Probable direction of progression was from left to right. See text for details.
The track-bearing dune facies surface was identified on loose slabs in an unstable rockfall area. By 2021, the surface could no longer be identified, presumably due to slumping of the slabs into the ocean.
DISCUSSION
Clearly the studied material, consisting of only one small-mammal trackway with probable associated burrows, and one spider traceway, is limited. However, it is significant from both ichnotaxonomic and facies perspectives. There is ample precedent in the paleoichnological literature for comparative analysis of similar mammal and spider trackways. The record of spider traceways goes back to the Paleozoic (Sadler Reference Sadler1993; Lockley and Hunt, Reference Lockley and Hunt1995), whereas that of hopping mammaliform trackmakers only goes back to the mid Mesozoic (Casamiquela, Reference Casamiquela1961; de Valais, Reference de Valais2009). In the sections that follow, we briefly review the fossil track record of both groups, before discussing the ichnology, behavior, and facies preferences of extant representatives of these groups.
Fossil trackways of small mammals
The track record of small mammaliforms extends back to the Jurassic and includes a few trackways that indicate hopping or bounding behavior. The most celebrated examples come from Argentina where the oldest “true mammal” trackways were first reported from the Middle Jurassic La Matilde Formation as Ameghinichnus patagonicus (Casamiquela, Reference Casamiquela1961) and A. manantialensis (de Valais, Reference de Valais2009). These exceptionally well-preserved tracks, showing all digit traces in fine detail, reveal evidence of both bounding or hopping behavior, as well as alternating gaits. As noted by de Valais (Reference de Valais2009), typical Ameghinichnus tracks have average footprint lengths and widths of 9.0 mm and 13.0 mm, respectively (L/W 0.7). Thus, they are similar in size to the tracks described here, but are wider.
Although there are reports of isolated mammal tracks from the Cretaceous, the only unambiguous trackway segment is that reported by Kim et al. (Reference Kim, Lim, Lockley, Xing and Choi2017) from the Lower Cretaceous Jinju Formation of Korea. This trackway, named Koreasaltipes jinjuensis, reveals clear evidence of a small hopping or bounding animal that registered a trackway, symmetrical about the mid-line, with nine consecutive pairs of pes prints. The mean trackway width is 21.3 mm (range 19.0–24.0 mm). The mean hop distance was given as ~4–5 times the pes length (= ~4.0 cm), and pes tracks had a mean width (W) and length (L) of 4.76 mm and 8.06 mm, respectively, and thus, a mean L/W of 0.59 (Kim et al., Reference Kim, Lim, Lockley, Xing and Choi2017). Most tracks revealed anteriorly directed toe traces, some with extended toe-drag traces registered in a fine, cohesive mud. The Korean trackway is similar to the Cape south coast trackway with respect to the ‘hopping’ trackway configuration, but the individual tracks are smaller, relatively wider, and were registered in a quite different sedimentological substrate.
It is beyond the scope of this discussion to describe other isolated mammal tracks from the Cretaceous, such as Schadipes crypticus from the Maastrichtian of Colorado (Lockley and Foster, Reference Lockley and Foster2003), as well as unnamed tracks from the Lower Cretaceous of Maryland (Stanford et al., Reference Stanford, Lockley and Weems2007), Tunisia (Contessi, Reference Contessi2013), and Angola (Marzola et al., Reference Marzola, Mateus, Schulp, Jacobs, Polcyn and Pervov2014, Reference Marzola, Mateus, Schulp, Jacobs, Polcyn, Pervov, Goncalves and Morais2015), the latter revealing isolated mammal tracks named Catocapes angolanus (Mateus et al., Reference Mateus, Marzola, Schulp, Jacobs, Polcyn, Pervov, Gonçalves and Morais2017). None of these tracks is comparable in size, individual track morphology, or trackway configuration, although the short, incomplete Schadipes crypticus segment shows ambiguous evidence of a hopping gait.
Turning to the Cenozoic record of trackways of small mammals, we again find very few examples of clearly defined trackways of hopping, rodent-sized animals. The formally defined ichnotaxa are named as Musaltipes occidens and M. longidigitus from the Cenozoic (Miocene) of Colorado and Utah, respectively (Lockley and Milner, Reference Lockley and Milner2014). The former ichnospecies occurs in an aeolian sandstone and is represented by symmetrical trackways revealing multiple pes pairs as ‘hop’ traces occurring in association with well-preserved arthropod trackways. Both ichnospecies reveal sub-optimal preservation, with individual tracks showing only indistinct digit traces. Musaltipes occidens is ~2.5 cm long and 1.25 cm wide (L/W ~2.0), and M. longidigitus is about half this size (L 1.2 cm, W 0.8 cm: L/W ~0.67), with hop lengths between ~6.0 cm and 8.0 cm. In many respects, as discussed below, ichnogenus Musaltipes, named to connote a hopping mouse, is the only fossil trackway similar to the Cape south coast example with respect to facies association, quality of preservation, size, age, and trackway configuration.
Few other small-mammal tracks are of significance with respect to the present study because they are not preserved as trackways and, given the poor preservation in many cases, offer few useful points of comparison. Lockley et al. (Reference Lockley, Helm, Cawthra, De Vynck and Perrin2021) recently described Musvestigium minutus from the Cenozoic of Colorado, which was named on the basis of a short trackway segment indicating an alternating gait. These authors also reviewed some of the small-mammal ichnotaxa reported from elsewhere in the Cenozoic (e.g., by Sarjeant and Langston, Reference Sarjeant and Langston1994). They also cited reports of unnamed Miocene tracks of hopping mammals from Miocene volcaniclastic sequences in Washington State (Kaler, 1998). No other Cenozoic tracks of small mammals invite comparison with the Cape south coast trackway.
Thus, in summary, there are only two Mesozoic trackways of small hopping mammals (Ameghinichnus and Koreasaltipes) that are comparable to the South African trackway. Likewise, there are only two Cenozoic trackways (Musalipes occidens and M. longidigitus) that are comparable and would tentatively allow us to label the South African trackway as cf. Musaltipes. All other aforementioned ichnotaxa and track morphotypes are based on isolated tracks or small trackway segments with different individual track morphologies and trackway configurations.
Fossil traceways of arachnids
As discussed below, fossil traceways and other traces of arthropods have a long geological range, and are too numerous to mention (Buatois and Mángano, Reference Buatois and Mángano2018). However, limiting our discussion to traceways attributed to large arachnids, particularly spiders, constrains comparative analysis to a much smaller set of traces, reviewed here, especially if we limit our focus to Octopodichnus and similar traces. A review of the available literature informs us that almost all occurrences are associated with dune facies, ranging in age from Permian to the present. In the following brief sections, we outline the stratigraphic distribution of Octopodichnus and compare traceway morphology with that of the Cape south coast traceway.
Paleozoic arachnid traceways have been known at least since the 1920s, and are widespread and appear typical of the dune facies (Fig. 6). The type specimen of Octopodichnius didactylus originates from the Coconino Sandstone of Arizona (Gilmore, Reference Gilmore1927; Brady, Reference Brady1947; Sadler, Reference Sadler1993), and the ichnogenus is also known from other Permian dune deposits, including the De Chelly Sandstone of Arizona (Sadler, Reference Sadler1993), the Lyons Sandstone of Colorado (Lockley and Hunt, Reference Lockley and Hunt1995), and the Weber Sandstone of Utah (Chure et al., Reference Chure, Engelmann, Good, Haymes, Hansen, Lockley and Lucas2014a). In the three examples shown in Figure 6, the diagnostic Octopodichnus configuration is shown, where the traceway is asymmetric about the mid-line, with alternating quadripartite sets of four tracks in an ‘L’-shaped configuration. Note also that the traceways are quite wide: ~8.0 cm in type O. didactylus and 6.0–9.0 cm in the examples shown in Figure 6. In well-preserved examples, the individual tracks show tarsal spine traces that bifurcate in the direction of anterior progression (hence the ichnospecies name O. didactylus). This reveals that typically the longer portion of the ‘L’ configuration, with two tracks situated anterior of the L's inflection point, is oriented antero-medially, whereas the shorter portion is marked by one postero-medially situated track. This configuration helps to identify the direction of progression when tarsal spine traces are not preserved. However, it should be noted that this L-shaped configuration may vary depending on the progression of the tracemaker, as in the case of Octopodichnus raymondi named by Sadler (Reference Sadler1993, fig. 7).

Figure 6. (A–C) Permian Octopodichnus from University of Colorado Museum (UCM) collections. (A) and (B) (UCM 139.93 and 139.83, respectively) from the Coconino Sandstone of Arizona. (C) Octopodichnus (UCM 139.72) from the De Chelly Sandstone of Arizona (from Lockley et al., Reference Lockley, Culver and Wegweisser2007). Note ‘L’-shaped quadripartite track sets (red boxes). Note also that some individual tracks show tarsal spine traces that bifurcate in the direction of forward progression. Thus, traceway 139.93 indicates progression to the right and 139.72 to the left. See text for details.
Mesozoic Octopodichnus have mostly been reported from the Lower Jurassic Navajo, Nugget, and Aztec sandstones of the western USA (Faul and Roberts, Reference Faul and Roberts1951; Rainforth and Lockley Reference Rainforth, Lockley and Morales1996a, Reference Rainforth, Lockley and Moralesb; Lockley et al., Reference Lockley2011; Chure et al., Reference Chure, Good, Engelmann, Lockley and Lucas2014b; Rowland et al., Reference Rowland, Breithaupt, Stoller, Matthews and Saines2014), and from the Jurassic–Cretaceous Botucatu Formation of Brazil (Buck et al., Reference Buck, Ghilardi, Fernandes and Fernandes2017). As shown in Figure 7, in some cases, Octopodichnus traceways indicate that both left and right sets of footprints failed to register completely. This appears to have been the case on the Cape south coast traceway (Fig. 5), which may reflect progression across a slope (e.g., in contour-parallel direction where left and right footprints might exert differential pressures on the substrate).

Figure 7. Four examples of partial Octopodichnus from the Nugget Sandstone of Idaho, showing characteristic quadripartite track sets (red boxes). UCM 179.116–118 are replicas, UCM 184.46 is an original specimen. Note that in all cases, only one side of the traceway is preserved. Compare with Figure 5 and Lockley et al. (2011, figs. 7, 8). See text for details.
Lockley et al. (Reference Lockley, Culver and Wegweisser2007) reported that the aforementioned trackways of small hopping mammals from the Miocene of Colorado (Lockley and Milner, Reference Lockley and Milner2014) were commonly associated with arthropod surface traceways, including at least two identified as cf. Octopodichus (Lockley et al., Reference Lockley, Culver and Wegweisser2007, fig. 8). This observation means that Octopodichnus has been reported in association with mammal or mammaloid tracks in dune facies ichnocoenoses from the Paleozoic (Permian), Mesozoic (Jurassic and Cretaceous), and Cenozoic (Miocene), as well as from the Pleistocene sites reported here (see discussion below).
Trackway and burrow interpretation
In a bounding gait, the hind legs provide the propulsion for pushing the trackmaker off the ground and forwards (Van den Heever et al., Reference Van den Heever, Mhlongo and Benadie2017). The trackmaker lands on the two front feet, which are usually smaller than the hind feet, and the manus tracks are thus situated closer to each other and close to the midline of the trackway. The hind legs then follow, over and around the front feet, landing ahead of them, farther apart from each other than in the case of the front feet, and farther from the midline. The push-off phase by the hind feet then initiates another bounding cycle. Such configurations, as detailed by Liebenberg (Reference Liebenberg2000), Stuart and Stuart (Reference Stuart and Stuart2019), and Van den Heever et al. (Reference Van den Heever, Mhlongo and Benadie2017), are consistent with the features present in the trackway described here, and suggest the front feet landing close enough to each other that the impressions they created coalesced into a single larger depression on three occasions, in ‘pairs’ 5, 7, and 9 in Figure 2. Such a configuration is clearly seen in the Miocene trackway (M. occidens) from Colorado. The alternative (less parsimonious) interpretation is of a hopping gait in which the distance between hindfoot track pairs happened to vary.
Extant small mammals capable of registering tracks on Cape south coast dunes of the size described here, with a bounding gait pattern, include gerbils and mice. Both may walk with an alternating gait, run, or employ a hopping or bounding gait. Identifying small-rodent tracks to trackmaker species in the field is difficult. However, gerbils, as a rule, indulge much more frequently in bounding, and are considered the most likely trackmaker group for the trackway described here (e.g., the extant and common Cape gerbil, Gerbilliscus afra). The most commonly encountered mouse in the region is the four-striped grass mouse (Rhabdomys pumilio), and is a plausible, albeit less likely candidate trackmaker species. Figure 8A illustrates G. afra in the process of registering a bounding gait pattern in sand, and Figure 8B depicts a bounding gait pattern of an extant small mammal from the Cape south coast.

Figure 8. (A) Cape gerbil (Gerbilliscus afra) registering a bounding-gait trackway, which includes short tail-drag impressions; scale bar = 30 cm. (B) Bounding gait pattern of an extant small mammal on the Cape south coast, in which the front-foot impressions have coalesced into single depressions, behind the paired hind-foot impressions; scale is in cm and mm.
The measured length of 7.5 cm between corresponding points on tracks pairs 5, 7, and 9 in Figure 2 is short for a pace length in a bounding gait pattern. Pace length in bounding gaits increases with trackmaker velocity, and sets of four tracks become spread farther apart at higher velocities (two manus tracks posteriorly, and two pes tracks ahead of them). Figure 9A illustrates the typical pattern in snow, and corresponds closely to the pattern reported for M. occidens trackways from the Miocene of Colorado (Fig. 2C) (Lockley et al., Reference Lockley, Culver and Wegweisser2007; Lockley and Milner, Reference Lockley and Milner2014). The even spacing noted in the described trackway may represent a slow bounding gait. Alternatively, it may conceivably represent the superimposition of one trackway on another, which fortuitously (but less parsimoniously) has resulted in even spacing of the tracks—a possibility that we consider unlikely. Small mammals regularly re-use their paths, and such a phenomenon may be encountered in extant small-mammal trackways that exhibit bounding gaits.

Figure 9. (A) Bounding gait pattern of a small mammal, registered in snow in British Columbia; (length of red base of ski pole = 5 cm; mean pace length = 20 cm). (B) Small-mammal tracks made by Tamiasciurus hudsonicus in snow in British Columbia, illustrating the level of detail that can be preserved in a fine-grained substrate; scale bar = 10 cm.
The capacity for track detail to be registered is greater in finer-grained substrates. High-quality small-mammal tracks are therefore more likely to be encountered in substrates of silt, mud, or snow. Figure 9B illustrates this phenomenon in snow (cf., Lockley and Milner, Reference Lockley and Milner2014, fig. 9). In the Cape south coast context, silty Pleistocene lagoonal deposits, reflecting depositional quiescence, have greater potential to record fine ichnological detail (i.e., better quality of preservation), but these are underrepresented on this coast compared to paleodune and paleobeach environments.
The dimensions (3–5 cm diameter) of the burrows (Fig. 3) on the loose block adjacent to the small-mammal tracksite are consistent with gerbil burrows or small golden mole burrows, among extant fossorial species (Stuart and Stuart, Reference Stuart and Stuart2019). In contrast, the two adjacent loose slabs at the burrow site 310 m to the east (Fig. 4) provide substantially more evidence of a gerbil origin—gerbils create mounds of debris adjacent to their tunnels (Fig. 10), which is consistent with the size of the raised features noted at this site (Stuart and Stuart, Reference Stuart and Stuart2019; Nigel Bennett and Chris Faulkes, pers. comm., 2021). These observations suggest that evidence of such mounds might be found in the fossil record, in addition to the Cape south coast example (Fig. 4). Indeed, in studies of Middle Jurassic burrows attributable to mammals, Loope (Reference Loope2006, fig. 7C; Reference Loope2008, fig. 7D) illustrated kangaroo rat burrows and mounds that are very similar to those illustrated here (Figs. 3, 4). As noted below, tetrapod burrows of mammal/mammaloid affinity have been reported from various Lower Jurassic aeolian deposits (Lucas, Reference Lucas, Gobetz, Odier, McCormick, Egan, Harris, Lucas, Spielmann, Lockley, Milner and Kirkland2006). The disintegration of the raised feature, interpreted here as a debris mound, serves as a reminder of the ephemeral nature of Cape south coast trace fossil sites, and the need for frequent coastal surveys, in particular after rockfall events and storm surges.
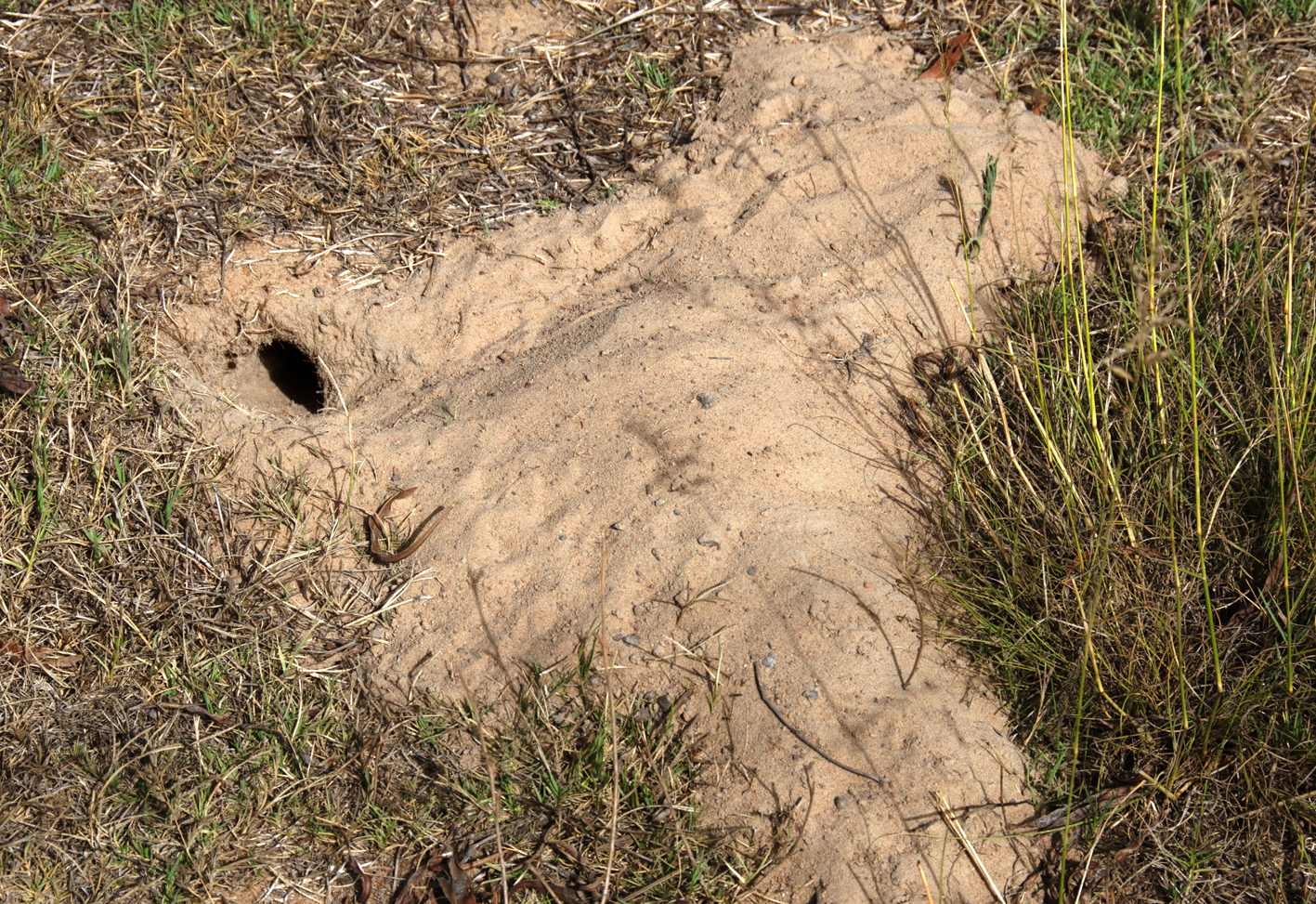
Figure 10. Debris mound beside the entrance to a Gerbilliscus afra burrow in a sandy, grass-covered setting (entrance hole is 5–7 cm in diameter); reproduced with permission from Chris and Mathilde Stuart.
In a study of micromammal remains from fossil and archaeological sites from the Cape south coast from MIS 9 through MIS 1, Matthews et al. (Reference Matthews, Marean and Cleghorn2020) reported remains of G. afra from all sites, and it remains a common denizen of the region in sandy soils. In combination, the various features identified at the tracksite and burrow sites therefore strongly suggest various forms of gerbil activity, and contribute to a sparse global record of such features.
Invertebrate trace fossils are common on Cape south coast Pleistocene paleosurfaces, but their analysis, description, and consideration of paleoenvironmental significance are in their infancy. The arthropod traceway described here illustrates the potential of this field for future study, especially as it relates to the interpretation of ichnofacies (Bautois and Mángano, Reference Buatois and Mángano2011).
The two large extant spider groups on the Cape south coast capable of registering traces of the size described here are the rain spiders (family Sparassidae, e.g., Palystes superciliosus) and the baboon spiders (family Theraphosidae). Stuart and Stuart (Reference Stuart and Stuart2019, p. 216) noted that “generally, you will find only the trails of large spiders, and then only in the finest of substrates,” and they also stated, in relation to the baboon spider, that “the spread of the legs… results in a wide straddle in the trail.” Likewise, Van den Heever et al. (2017, p. 298) noted that “spider footprints are represented by faint lines or dots which can be seen only in perfectly soft substrate.” Figure 11 depicts a traceway of a baboon spider registered in sand, and a cast of a rain spider from the Cape south coast.

Figure 11. (A) Baboon spider traceway from South Africa; reproduced with permission from Chris and Mathilde Stuart. (B) Cast of rain spider from the Cape south coast; scale bar = 10 cm.
These neoichnological observations raise interesting questions about the preservation of spider and other arthropod traceways in dune sediments. Firstly, most dune deposits consist predominantly of fine-grained, well-sorted sand, as noted in previous citations to Paleozoic through Mesozoic and Cenozoic facies. Thus, as noted by Stuart and Stuart (Reference Stuart and Stuart2019), these substrates are suitable for registration and preservation of small tracks. However, given that dune deposits are often in dynamic, wind-induced motion and ostensibly dry, it is not obvious how well-preserved spider tracks, in some cases with tarsal spine traces (Fig. 6), might be preserved. Based on the work of Milàn and Bromley (Reference Milàn and Bromley2006), Milàn and Loope (Reference Milàn and Loope2007, p. 383) stated that “Experiments with track formation in different horizontal substrates, including dry sand, damp sand, and wet sand, demonstrate that totally dry sand is a bad medium to preserve true tracks.” One possibility is that the dunes were wet, dampened by dew or mist. How such moistening of coastal dunes facilitates registration of high-quality arthropod traces is vividly illustrated in documentaries, such as Creatures of the Namib Desert (National Geographic Society, Reference Saxon and Hughes1978). Once wetted, sand may quickly induce the growth of interstitial microbial (cyanobacterial) networks, which bind sand grains and enhance the preservation of traces. Seilacher (Reference Seilacher2008, p. 256) cited Octopodichnus from Permian dune faces of Arizona as a specific example of trackways in “moist dune surfaces … stabilized by the ‘bioglue’ of microbial grain envelopes.”
Ichnocoenoses and ichnofacies
There is a growing literature on terrestrial invertebrate and vertebrate (tetrapod) ichnocoenoses and ichnofacies (for a brief introduction to vertebrate/tetrapod ichnocoenoses and ichnofacies concepts, see Lockley et al., Reference Lockley, Hunt, Meyer and Donovan1994; Hunt and Lucas, Reference Hunt and Lucas2007, Reference Hunt and Lucas2016; and Buatois and Mángano, Reference Buatois and Mángano2011). These ichnocoenoses are defined, albeit somewhat loosely, as recurrent associations of trace fossils representative of once extant biological communities, which are usually facies related (given biological communities’ preferences for certain paleoenvironments, depositional systems, and or ecosystems). According to Hunt and Lucas (Reference Hunt and Lucas2007, Reference Hunt and Lucas2016), multiple tetrapod ichnocoenoses from similar terrestrial facies (e.g., the Paleozoic, Mesozoic, and Cenozoic dune facies examples given here) can be grouped into a single global or archetypal ichnofacies, as is the usual procedure in the study of marine ichnofacies. Thus, Hunt and Lucas (Reference Hunt and Lucas2007, Reference Hunt and Lucas2016) subsumed the Permian and Jurassic dune facies ichnocoenoses into the Chelichnus ichnofacies, which they described as “recurrent in dune faces of eolian environments,” but they stated that it only “extends from the early Permian to the Early Jurassic” (Hunt and Lucas, Reference Hunt and Lucas2016, p. 240). This is not disputed as a starting point for further discussion, but is elaborated below.
Lockley et al. (Reference Lockley2007, p. 59) had noted that the Miocene dune assemblages reveal “a distinctive facies-controlled small mammal and arthropod-dominated track assemblage representative of the Chelichnus ichnofacies […] also the first example of this ichnofacies reported from the Cenozoic.” The notion that this ichnofacies might be extended beyond the Early Jurassic was further discussed by Krapovickas et al. (Reference Krapovickas, Mángano, Buatois and Marsicano2016) in a comprehensive review of the ichnology of aeolian environments. In a recent report on a Late Jurassic dune tetrapod ichnocoenosis from the coastal dune facies of the Moab Member of the Curtis Formation in eastern Utah, the ichnocoenosis from that unit may not be attributable to the Chelichnus ichnofacies (see Lockley, Reference Lockley2021, for cautious use of the label ?Chelichnus ichnofacies). This uncertainty is in part due to the invertebrate and tetrapod components of the ichnocoenosis as well as the sedimentary facies, which lack both Octopodichnus and recognizable small-mammal tracks (Hunt and Lucas, Reference Hunt and Lucas2016; Lockley, Reference Lockley2021).
It is somewhat of an impediment to full understanding of terrestrial ichnofacies that invertebrate and vertebrate (tetrapod) ichnofacies have too often been studied and defined separately. In fact, there is debate as to whether their definition can or should follow the same guidelines (Hunt and Lucas, Reference Hunt and Lucas2007, Reference Hunt and Lucas2016; Lockley, Reference Lockley2007; Santi and Nicosia, Reference Santi and Nicosia2008), given that vertebrates are more mobile and evolved more rapidly. Fortunately, there is general agreement that there is close correspondence between invertebrate and vertebrate ichnofacies from dune settings. This was stated succinctly by Krapovickas et al. (2016, p. 63), who noted that “…numerous authors highlighted the concurrent characteristics of the eolian trace-fossil assemblages in the … contrasting Chelichnus, Octopodichnus, and Entradichnus ichnofacies and suggested the integration of these separate models (e.g. Buatois and Mángano, Reference Buatois and Mángano2011; Ekdale and Bromley, Reference Ekdale, Bromley, Knaust and Bromley2012; Krapovickas et al., Reference Krapovickas, Mancuso, Arcucci and Caselli2010, Reference Krapovickas, Mángano, Buatois and Marsicano2016). Originally, Hunt and Lucas (2007) emphasized the close correspondence between the Octopodichnus and Chelichnus ichnofacies (Hunt and Lucas, Reference Hunt and Lucas2007, p. 67). This same idea was already stated by Lockley et al. (Reference Lockley, Hunt, Meyer and Donovan1994) in the original definition of the Chelichnus ichnofacies where the authors indicated the common correspondence between Laoporus (=Chelichnus) and invertebrate trace fossils, such as Octopodichnus and Paleohelcura.”
Hunt and Lucas (Reference Hunt and Lucas2016, p. 238), “regard the ‘Entradaichnus ichnofacies’ as a synonym of the Scoyenia ichnofacies.” This implies it has no obvious relationship to the Octopodichnus ichnofacies. As reviewed by Lockley (Reference Lockley2021, p. 15), “a label like ‘Chelichnus-Octopodichnus ichnofacies [as] a natural combination (Lockley, Reference Lockley2004)… would allow naming of ichnofacies based on full integration of both vertebrate and invertebrate ichnofaunal evidence.” In other words, “the separate invertebrate (Octopodichnus) and vertebrate (Chelichnus) ichnofacies names for the same eolian or dune ichnofacies” had been proposed for ichnofaunas already recognized as “entirely co-extensive” (Lockley Reference Lockley2007, p. 51).
In short, the dune facies ichnofaunas are among those that most frequently show consistent similarities between their invertebrate and vertebrate traces from site to site, particularly with respect to the co-occurrence of mammaliform and arachnid surface trails. This is possibly due to the low diversity and ease of identification of many dune ichnofaunas. For this reason, it is tempting to simply conclude that dune ichnofaunas represent a single broadly defined ichnofacies ranging in age from Paleozoic to Recent. However, as the Late Jurassic Moab Member ichnocoenosis indicates, not all dune ichnocoenoses are the same (Lockley, Reference Lockley2021) (see, for example, Marchetti et al., Reference Marchetti, Voigt, Lucas, Francischini, Dentzien-Dias, Sacchi and Mangiacotti2019b, who identified a higher ichnofaunal diversity on some Permian dune substrates). Thus, too broad, if not oversimplified, categorizations mean it is difficult, or at least unsatisfactory, to define overarching or ‘archetypal’ ichnofacies in detail based only on invertebrate or vertebrate components, not least because they have generally been defined independently by those working separately on vertebrate or invertebrate ichnofaunas. Another complicating factor is that there is persuasive evidence that some infaunal burrows created by mammals, or mammaliform tracemakers, are attributable to the same groups (?species) as the makers of surface trackways, as noted above. Burrowing invertebrates, including spiders, also make surface traceways.
Presently, given that the Chelichnus ichnofacies sensu Hunt and Lucas (Reference Hunt and Lucas2007, Reference Hunt and Lucas2016) is defined on the basis of tetrapod tracks as limited to the Permian through Lower Jurassic, and the Octopodichnus ichnofacies, also defined by various authors (see Krapovickas et al., Reference Krapovickas, Mángano, Buatois and Marsicano2016) as ranging from Permian to Recent, we are left to ponder the relationships and appropriate ichnocoenoses and ichnofacies labels applicable to Cretaceous through Cenozoic dune ichnofaunas. For example, Peixoto et al. (Reference Peixoto, Mángano, Minter, dos Reis Fernandes and Fernande2020) noted that the occurrence of traceways like Octopodichus helps trace continuity between Paleozoic and post-Paleozoic ichnofaunas, at least in the sense of the eponymous Octopodichnus ichnofacies, when regarded as an ‘invertebrate’ ichnofacies recognized independently of tetrapod traces. The extent to which different ichnofaunas, including their tetrapod components, might be considered representative of different dune/desert paleoenvironments (e.g., with different precipitation regimes) is open to discussion, as noted by Krapovickas et al. (Reference Krapovickas, Mángano, Buatois and Marsicano2016), and naturally involves wide-ranging and complex consideration of paleoenvironmental and paleobiological evolution in space and time (e.g., Buatois and Mángano, Reference Buatois and Mángano2011). Moreover, the Cape south coast coastal dune ichnofaunas discussed here exemplify such complexity—more than one ichnocoenosis (or ichnofacies) may be represented and challenging to identify, as in the case of interpreting the small-mammal and spider surface trails on the one hand, and, on the other, their relationship ‘within’ coastal dune facies replete with very different large-animal traces and infaunal burrows. Moreover, there are obvious differences between coastal dune ichnofaunas and those associated with large ergs deposited in arid continental interior deserts.
It is outside the scope of this contribution to opine, definitively, as to how various invertebrate and vertebrate ichnoassemblages, ichnocoenoses, and ichnofacies and their distributions in space and time should be categorized and labeled. However, what can be confidently stated is that it is already recognized that dune facies are host to mammal/mammaloid and arachnid trackway assemblages, and variously labeled ichnocoenoses include the Permian Chelichnus and longer-ranging Octopodichnus ichnocoenoses, subsumed in the eponymous Chelichnus and Octopodichnus ichnofacies, respectively. At the time of writing, the Octopodichnus ichnofacies is ostensibly recognized from the Permian to Recent (Lockley et al., Reference Lockley, Hunt, Meyer and Donovan1994; Lockley, Reference Lockley2004; Hunt and Lucas, Reference Hunt and Lucas2007; Krapovickas et al., Reference Krapovickas, Mángano, Buatois and Marsicano2016; Peixoto et al., Reference Peixoto, Mángano, Minter, dos Reis Fernandes and Fernande2020), an inference consistent with the Waenhuiskrans Formation assemblage reported here (Fig. 12). However, to date, the Chelichnus ichnofacies, with its component Chelichnus and Brasilichnium ichnocoenoses, has been reported to range from Permian to Lower Jurassic (Hunt and Lucas, Reference Hunt and Lucas2016). Given the aforementioned co-occurrence of an assemblage of mammal (Musaltipes) and arthropod (including Octopodichnus) trackways, already identified as representative of the Chelichnus ichnofacies (Lockley et al., Reference Lockley, Culver and Wegweisser2007), as well as the present report of a very similar co-occurrence in the Pleistocene Waenhuiskrans Formation, a case can be made for inferring an extended temporal range for the Chelichnus ichnofacies to the Recent (Fig. 12). Also as noted, the obvious relationship between mammalian/mammaliform trackways and burrows adds a biological/ichnological signal that supports this interpretation.

Figure 12. Generalized stratigraphic column showing better-known dune deposits that have yielded mammal/mammaloid and arachnid track assemblages (or ichnocoenoses) attributed to or potentially attributable to the Chelichnus and Octopodichnus ichnofacies. Possible extended range of Chelichnus ichnofacies shown with red arrow. Superscript numbers 1–17 with formation names refer to original or secondary sources as follows 1: Chure et al. (Reference Chure, Engelmann, Good, Haymes, Hansen, Lockley and Lucas2014a); 2–6, 8, 9: Lockley and Hunt (Reference Lockley and Hunt1995) or Hunt and Lucas (Reference Hunt and Lucas2007, Reference Hunt and Lucas2016, and references therein); 7, 14, 15: Francischini et al. (Reference Francischini, Dentzien-Dias, Lucas and Schultz2018); 10: Lockley (Reference Lockley2021); 11: Buck et al. (Reference Buck, Ghilardi, Fernandes and Fernandes2017); 12: Lockley et al. (Reference Lockley, Culver and Wegweisser2007); 13: Haubold et al. (Reference Haubold, Lockley, Hunt, Lucas, Lucas and Heckert1995); 16: Raath and Yates (Reference Raath and Yates2005); 17: this study. The inference that the Curtis Formation dune facies ichnofauna represents the Chelichnus ichnofacies is questionable.
CONCLUSIONS
Large-vertebrate Pleistocene tracksites on the Cape south coast have led to significant paleoenvironmental inferences. Expanding this field of study to smaller-vertebrate and invertebrate trackmakers, such as the small-rodent trackway (and associated burrows) and arthropod (spider) traceway described here, adds to the diversity of trackmaking taxa represented. Such evidence has the dual value of (1) demonstrating that small-animal traces can be preserved and (2) opening a discussion of the paleoecology, which is highly convergent with the literature on both invertebrate and vertebrate ichnofacies. Thus, the presence of mammal tracks (cf., Musaltipes) made by bounding rodents, and spider tracks (Octopodichnus) similar to those found in Miocene dune deposits, suggests we can recognize, in the Pleistocene, both the Chelichnus and Octopodichnus ichnofacies, as defined by vertebrate and invertebrate ichnologists, respectively. The temporal range of the Chelichus ichnofacies, formerly identified in Permian through Early Jurassic dune deposits, is thereby a candidate for extension through the Cenozoic. While the presence of small-mammal tracks in dune facies is a characteristic signal of the Chelichnus ichnofacies, until now the presence of burrows (Paleozoic through Cenozoic) attributed to these same mammal and mammaliform trackmakers has not been explicitly cited as evidence in support of such ichnofacies schema. Such additional ichnnological evidence is pertinent to the description and definition of ichnocoeonoses and ichnofacies, and should be included.
Acknowledgments
We thank Nigel Bennett, Jacob Carrigan, Kirsty Casey, Chris Faulkes, Pieter-Jan Gräbe, Carina Helm, Thalassa Matthews, Chris Stuart, Mathilde Stuart, Peter Todd, and Alex Van den Heever for their assistance and support. Luis Buatois and Gabriela Mángano reviewed the manuscript prior to submission and made much-appreciated suggestions. We thank reviewers Eudald Mujal and Lida Xing and Associate Editor Tyler Faith for their thorough, thoughtful comments and suggestions, incorporation of which enhanced our manuscript. We thank Christopher Maples for thorough copy-editing of the manuscript.