Introduction
Billions of birds undertake annual migrations between breeding and nonbreeding grounds (Dokter et al. Reference Dokter, Farnsworth, Fink, Ruiz-Gutierrez, Hochachka and La Sorte2018; Hahn et al. Reference Hahn, Bauer and Liechti2009; Van Doren and Horton Reference Van Doren and Horton2018). Aside from its crucial importance in the life cycles of migratory species, avian migration also plays an essential role in nutrient and energy transportation at a global scale, and in mediating community dynamics and ecological interactions (Bauer and Hoye Reference Bauer and Hoye2014; Hahn et al. Reference Hahn, Bauer and Liechti2009). Population declines of migratory birds may thus compromise ecosystem integrity, functions, and services (Şekercioğlu et al. Reference Şekercioğlu, Daily and Ehrlich2004), in addition to the negative impacts sustained by the species themselves. Unfortunately, accumulating evidence indicates that migratory birds are experiencing widespread population declines, highlighting the urgency of effective conservation actions aimed at these species (Bairlein Reference Bairlein2016; Rosenberg et al. Reference Rosenberg, Dokter, Blancher, Sauer, Smith and Smith2019; Vickery et al. Reference Vickery, Ewing, Smith, Pain, Bairlein and Škorpilová2014). At the centre of devising such actions is long-term population monitoring of migratory birds, especially during migration – the phase of their life cycles when the highest mortality occurs (Klaassen et al. Reference Klaassen, Hake, Strandberg, Koks, Trierweiler and Exo2014; Newton Reference Newton2006).
Population monitoring during migration poses considerable technical and logistical challenges, particularly for migratory landbirds. Unlike shorebirds, most migratory landbirds do not congregate in large numbers at relatively well-defined stopover sites amenable to targeted surveys (Buler and Dawson Reference Buler and Dawson2014). They also tend to migrate at night (Dokter et al. Reference Dokter, Farnsworth, Fink, Ruiz-Gutierrez, Hochachka and La Sorte2018; Gillings and Scott Reference Gillings and Scott2021), defying simple visual observation outside of rare cases. In addition, most of them are too small to support even the most advanced and miniaturised tag technology. Although large-scale field observations supported by citizen science have offered an invaluable opportunity to characterise the distribution and abundance of migratory landbirds diurnally (Fink et al. Reference Fink, Auer, Johnston, Ruiz-Gutierrez, Hochachka and Kelling2020, Reference Fink, Johnston, Strimas-Mackey, Auer, Hochachka and Ligocki2023; Walker and Taylor Reference Walker and Taylor2017), the underlying migratory processes, including the dynamics of migratory timing, location, and magnitude, remain latent or disconnected from such monitoring.
An emerging method to potentially overcome such challenges is bioacoustic monitoring of nocturnal flight calls (NFCs) (Van Doren et al. Reference Van Doren, Lostanlen, Cramer, Salamon, Dokter and Kelling2023). While NFC monitoring started as early as the late nineteenth/early twentieth centuries (Ball Reference Ball1952; Libby Reference Libby1899), it was after the mid-twentieth century when nascent recording technology advanced significantly that the potential of such monitoring expanded dramatically (e.g. Graber and Cochran Reference Graber and Cochran1959). The first large-scale opportunities to monitor the NFCs of migrating birds arose only in the late twentieth century (e.g. Evans and Rosenberg Reference Evans, Rosenberg, Bonney, Pashley and Cooper2000). NFC monitoring is enabled by the fact that many birds utter species-specific calls during nocturnal migratory flights that allow species identification (e.g. Farnsworth Reference Farnsworth2005), information that is often difficult to obtain by other means of remote sensing such as radar imagery (Guo et al. Reference Guo, Buler, Smolinsky and Wilcove2023; Shi et al. Reference Shi, Hu, Soderholm, Chapman, Mao and Cui2023). Whilst NFC monitoring has been increasingly used in North America and Europe over the last few years (Gillings and Scott Reference Gillings and Scott2021; Heiss Reference Heiss2018; Horton et al. Reference Horton, Shriver and Buler2015a; Van Doren et al. Reference Van Doren, Lostanlen, Cramer, Salamon, Dokter and Kelling2023; Watson et al. Reference Watson, Wilson and Mennill2016; Winger et al. Reference Winger, Weeks, Farnsworth, Jones, Hennen and Willard2019), its application in other regions remains relatively limited. The opportunity for its application, however, is straightforward in these other regions, with many guides and databases having been developed to help the identification of NFCs (e.g. Bergmann et al. Reference Bergmann, Chappuis and Dingler2014; Cornell Lab of Ornithology 2022; Farnsworth Reference Farnsworth2005; Planqué et al. Reference Planqué, Vellinga, Pieterse and Jongsma J. de By2005; Robb et al. Reference Robb and Pelikan2021).
The East Asian–Australasian Flyway (EAAF), one of nine major migration systems in the world, supports a diverse and abundant migratory bird community, with many species poorly studied and some highly threatened (Li et al. Reference Li, Hughes and Dudgeon2019; Yong et al. Reference Yong, Liu, Low, Española, Choi and Kawakami2015, Reference Yong, Heim, Chowdhury, Choi, Ktitorov and Kulikova2021). Assessments of population status and trends are lacking for much of the migratory bird community on the EAAF, especially for landbirds, although many species are likely to have experienced declines given the alarming declines documented for shorebirds (Studds et al. Reference Studds, Kendall, Murray, Wilson, Rogers and Clemens2017; Szabo et al. Reference Szabo, Choi, Clemens and Hansen2016), waterbirds (Harris and Mirande Reference Harris and Mirande2013; Jia et al. Reference Jia, Koyama, Choi, Kim, Cao and Gao2016; Wang et al. Reference Wang, Kuang, Tan and Ma2018), and some select landbird species (Choi et al. Reference Choi, Nam, Kim, Park and Park2020a; Edenius et al. Reference Edenius, Choi, Heim, Jaakkonen, De Jong and Ozaki2017; Kamp et al. Reference Kamp, Oppel, Ananin, Durnev, Gashev and Hölzel2015) in the region. The growth of citizen science on the EAAF is accumulating invaluable insights on the region’s migratory birds (Choi et al. Reference C.-Y, Nam, Park and Bing2020b; Heim et al. Reference Heim, Heim, Beermann, Burkovskiy, Gerasimov and Ktitorov2020; Studds et al. Reference Studds, Kendall, Murray, Wilson, Rogers and Clemens2017; Yamaura et al. Reference Yamaura, Schmaljohann, Lisovski, Senzaki, Kawamura and Fujimaki2017), but this avifauna remains poorly studied, particularly in terms of consistent population monitoring and assessment. NFC monitoring, thus far rarely adopted in this region, promises an important complement to ongoing citizen science observation efforts as a standardisable, efficient, and at-scale approach for monitoring EAAF’s migratory birds and landbirds in particular.
Here, we present the findings of a nascent NFC monitoring project in Beijing, set up as a proof-of-concept case for wider, long-term monitoring efforts in Beijing and elsewhere on the EAAF. Using data collected from a recording station in north-central Beijing over four migration seasons between 2021 and 2023, we report the total numbers of NFCs and species recorded for each season, and the habitat association and migration phenology of each species. Importantly, we present the above findings in the context of citizen science observation data from Beijing during the same seasons over a 20-year period to assess the extent to which NFC monitoring can complement citizen science in obtaining information on migratory birds. To our knowledge, this study is the first formal investigation of bird migration on the EAAF using NFC monitoring.
Methods
Study site and recording set-up
We conducted our study in Beijing Municipality, located in the north of the North China Plain. With the Bohai Sea to the east and the Gobi Desert to the west, both large ecological barriers to many migratory birds, this region likely serves as an important corridor for migratory birds on the EAAF (Holt et al. Reference Holt, Catley and Tipling1995; Hu et al. Reference Hu, Gu, Luo, Lu, Wei and Zhong2020). At least 508 bird species have been recorded in Beijing, the majority of which are migratory (Zhao and Zhu Reference Zhao and Zhu2021).
We set up a Wildlife Acoustics Song Meter Mini recorder (Wildlife Acoustics Inc. 2023) on the roof of the Asian Infrastructure Investment Bank headquarters, an 80-m high building located in north central Beijing (40.01´N, 116.39´E, 45 m a.s.l.) (Figure 1). The height of this building and its location on the edge of the Olympic Forest Park, a large greenspace of 680 ha, ensured minimal air traffic and ground noise around the recorder, and thus a relatively quiet recording environment despite its location in the centre of this metropolis. The recorder is programmable, has a single built-in omni-directional microphone, and saves recordings in one-hour WAV-format audio files. We programmed it to record at a default sampling rate of 24 kHz and maximum gain for better detection of NFCs. We considered the 24-kHz sampling rate as striking the best balance between battery life (Wildlife Acoustics Inc. 2023) and the coverage of bird calls in the study region (to our knowledge, only the high-frequency part of flight calls of some buntings Emberiza spp. can reach 11–12 kHz).
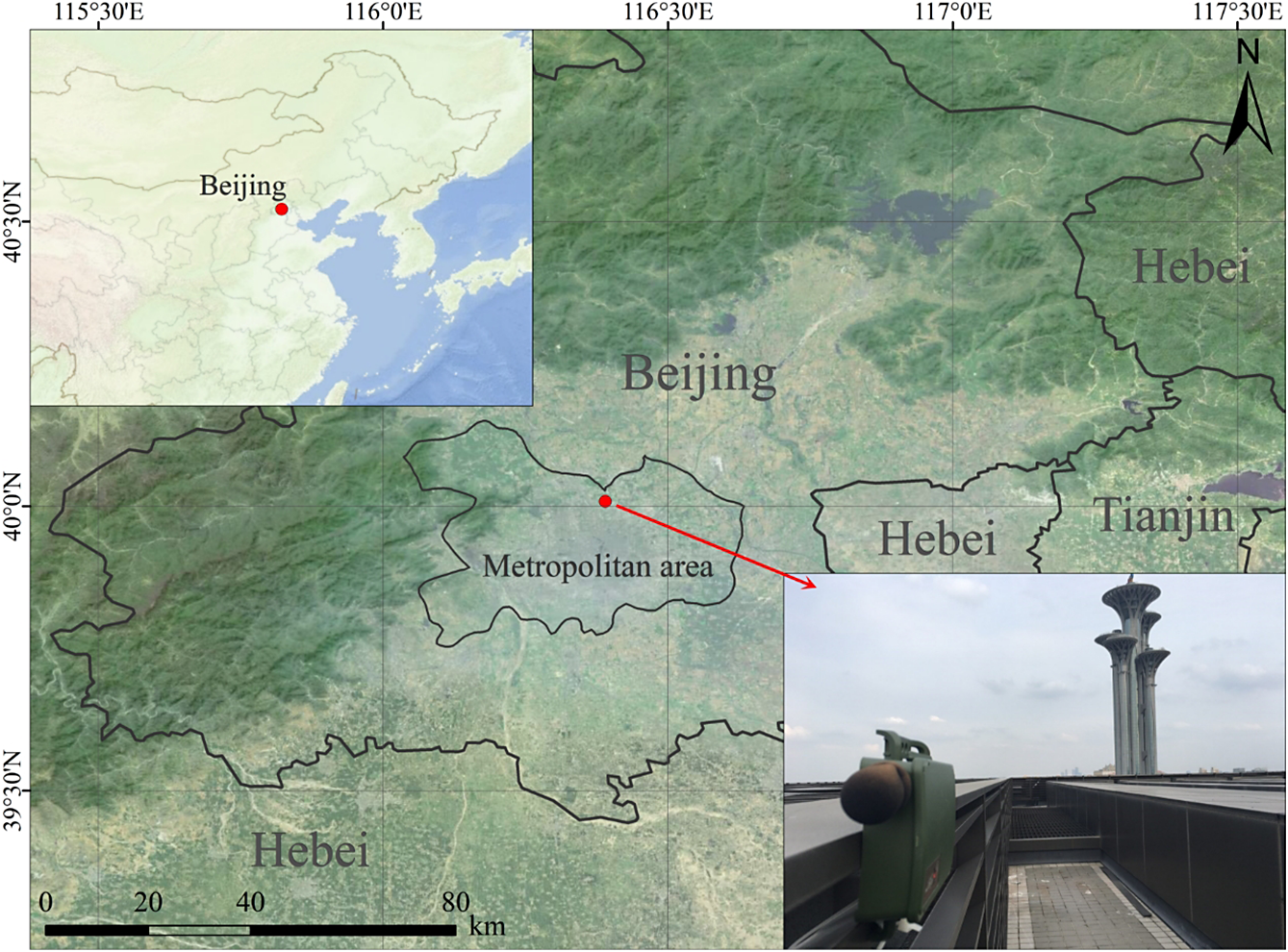
Figure 1. The location and setup of our recorder. The building is situated immediately south of the Olympic Forest Park and opposite the Beijing Olympic Tower.
We conducted recording each night during the spring and autumn migration seasons of 2021, 2022, and 2023, with the following date ranges and recording times: (1) 25 August to 2 November 2021, from sunset to sunrise; (2) 16 March to 7 June 2022, from one hour after sunset to one hour before sunrise; (3) 18 July to 10 November 2022, from sunset to sunrise; (4) 15 March to 8 June 2023, from sunset to sunrise. We selected these date ranges because, based on a local bird field guide (Friends of Nature 2014), citizen science data (eBird and Birdreport, see below), and our field experience, they encompass the migration seasons of most bird species in Beijing. We extended the autumn date range of 2022 compared with 2021 to cover more completely the autumn migration season. We experimented with shortening the recording time in spring 2022 to include only “true” nocturnal calls. However, because data filtering can easily be carried out during data processing, we did not do so in the following seasons.
Acoustic data processing
We manually processed audio files saved by the recorder using Audacity 3.0.2 (Audacity Team 2021). For each file, we successively displayed the spectrogram (0–10,000 Hz) of each 15-second clip full-screen to scan for bird vocalisations, using the linear scale, grey-scale palette, and Hamming window type. We extracted each individual NFC, identifying it to species or species group (for closely resembling species such as flycatchers and buntings) wherever possible. For sounds that were clearly bird vocalisations but could not be identified with high confidence to the order level at the time of data extraction, we labelled them as “unidentified”. To minimise data extraction bias introduced by assessor identity, one single assessor (T.T., who possesses over 10 years of birdwatching experience in Beijing) conducted all data extractions. Species identifications were based on the assessor’s field experiences, as well as consultation with several experienced wildlife sound recordists from Asia and Europe and the public sound database xeno-canto: https://xeno-canto.org/ (Planqué et al. Reference Planqué, Vellinga, Pieterse and Jongsma J. de By2005). Taxonomy and nomenclature followed the Clements Checklist 2022 (Clements et al. Reference Clements, Schulenberg, Iliff, Fredericks, Gerbracht and Lepage2022). With experience accumulation, some NFCs that were initially labelled as unidentified were later identifiable to more specific taxa. However, due to the workload involved, we did not go back to previous data entries to re-identify these NFCs. For example, we were not able to count the number of NFCs from flycatchers in autumn 2021 and spring 2022 because we labelled them as “unidentified”, but we were able to do so in autumn 2022 and spring 2023 after learning their NFCs.
We analysed and visualised all data using programme R (version 4.2.2; R Core Team 2022) and package ggplot2 (version 3.4.1; Wickham Reference Wickham2016). We first assigned each call to a given night, defined as the period between the sunset of the previous day and the sunrise of the given day. For each species or species group during a given season, we then tallied the total number of calls, differentiating among the various phases of the night time as demarcated by civil dusk and civil dawn (solar elevation angle = -6°). This differentiation is in light of the increasing evidence from North America and Europe that, compared with sunset and sunrise, civil dusk and civil dawn better demarcate the temporal span of nocturnal migration for most species (Cooper et al. Reference Cooper, Dossman, Berrigan, Brown, Brunner and Chmura2023; Heiss Reference Heiss2018; Gillings et al. Reference Gillings, Moran, Robb, van Bruggen and Troost2018). Yet because of the lack of information from EAAF, it would be risky to discard data from the evening and morning civil twilight hours (i.e. from sunset to civil dusk, and from civil dawn to sunrise) outright, so we broadly regarded all the bird calls we recorded as NFCs. We used package suncalc (version 0.5.1; Thieurmel and Elmarhraoui Reference Thieurmel and Elmarhraoui2022) to calculate the time of local night phases, and for each species, we tallied the total number of NFCs for each phase: evening civil twilight, morning civil twilight, and bona fide night (i.e. from civil dusk to civil dawn). To depict species’ habitat association, a single assessor (S.L.) classified all species or species groups into one of five habitat types, i.e. woodland, shrubland, grassland, wetland, and reedbed, based on descriptions of passage habitat (or non-breeding habitat, if no distinction between passage and wintering habitats was made) in the Birds of the World (Billerman et al. Reference Billerman, Keeney, Rodewald and Schulenberg2022).
Although our target species were migratory birds passing through Beijing, NFCs captured by our recordings came from a mixture of migratory species, local breeders/nonbreeders, and resident species. We excluded five resident species from our data set (i.e. Oriental Magpie Pica serica, Light-vented Bulbul Pycnonotus sinensis, Chinese Blackbird Turdus mandarinus, Scaly-breasted Munia Lonchura punctulata, and Little Owl Athene noctua), but we retained other species that were possibly migratory but had local resident or breeding/nonbreeding populations, noting that data extracted from our recordings may have been from non-migratory individuals.
Additionally, we assessed the variations in recording conditions between seasons, including weather features and background noise. We obtained data on dew point temperature, wind speed, air temperature, and sea-level pressure for Beijing from the Integrated Surface Dataset (NOAA National Centers for Environmental Information 2001), at three-hour intervals from 18h00 to 06h00 over the same date ranges as our recording data. This data set has a quality indicator for each observation value, and we included only data that passed all quality control checks (i.e. quality indicator = 1). We also quantified the mean loudness (measured in sones) of five one-minute samples randomly selected from every one-hour recording, using the package soundgen in programme R (version 2.6.0; Anikin Reference Anikin2019). We then assessed whether the four weather parameters and the mean loudness of sampled sound clips differed significantly among recording seasons, using analysis of variance (ANOVA) and multiple comparisons with Bonferroni correction.
Comparison with citizen science observation data
We compared data on migratory timing and counts obtained from the NFC recordings with those from citizen science observations for species with ≥150 NFCs recorded in at least one season. We excluded the Siberian Rubythroat Calliope calliope, for which we did not have good knowledge of its full NFC variations; we also excluded common local breeders (e.g. Red-rumped Swallow Cecropis daurica) because most of their calls captured would be from local populations. Given the different nature and format of the two data types, we standardised them to a seasonal range of NFC or observation numbers. For each species or species group, we rescaled its nightly number of NFCs to between 0 and 1 using the following formula:
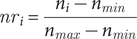
where nri and ni are the rescaled and original number of NFCs on night i for the species concerned, respectively, and nmin and nmax the minimum and maximum number of nightly NFC totals for the species concerned during the season concerned.
We obtained citizen science observation data for Beijing in the form of public birdwatching records from two sources: eBird (Sullivan et al. Reference Sullivan, Wood, Iliff, Bonney, Fink and Kelling2009; https://ebird.org/) and the Chinese citizen science database, Birdreport (http://www.birdreport.cn/). We did not include a larger geographical region to obtain more citizen science data, because the neighbouring Hebei Province is a data-poor region. To describe the temporal pattern of migration intensity for each species under consideration, we tallied, for each day, the number of checklists (including incomplete checklists) that contained the species over the date ranges of 15 March to 8 June and 18 July to 10 November between 2002 and 2022 (i.e. the same date ranges as our recording data for the past 20 years). For species groups under consideration that were not identifiable to species, we used the most commonly recorded candidate species as substitutes, and we conducted daily checklist tallies for each substitute species (e.g. we used four substitute species for the flycatcher group Muscicapidae spp.: Dark-sided Flycatcher Muscicapa sibirica, Asian Brown Flycatcher Muscicapa dauurica, Taiga Flycatcher Ficedula albicilla, and Yellow-rumped Flycatcher Ficedula zanthopygia). We used the number of checklists rather than individuals as the proxy for migration intensity because many checklists from Birdreport did not provide individual counts. We used pooled data from the past 20 years because of the relatively small number of checklists during our study years, which may produce a biased representation of the temporal pattern of migration intensity. We selected a 20-year period because the first checklist with an accurate date at Birdreport was from 2002, and eBird checklists from Beijing were few in number before 2002. For each species, we applied the same rescaling formula as in Equation 1 to standardise daily checklist tallies.
Results
In total, we obtained 3,490 recording files (~3,350 hours; the last file of each day was less than one hour in length) over the four migration seasons, from which we extracted 84,135 NFCs. Not considering the approximately 4% of NFCs (3,638) we were unable to identify to the order level, these NFCs involved 111 migratory or partially migratory bird species or species groups (see Supplementary material Table S1), approximately 60% (66) of which were typical landbirds, including songbirds, cuckoos, kingfishers, swifts, nightjars, and raptors (Yong et al. Reference Yong, Heim, Chowdhury, Choi, Ktitorov and Kulikova2021). Even if we excluded the civil twilight hours, there were still 70,254 NFCs involving 99 bird species or species groups (Figures 2 and 3, Table S1). The full set of NFCs (i.e. not excluding civil twilight hours) included 34,654 NFCs involving 61 identified species or species groups in autumn 2021, 4,402 NFCs involving 58 identified species or species groups in spring 2022, 34,440 NFCs involving 63 identified species or species groups in autumn 2022, and 10,679 NFCs involving 63 identified species or species groups in spring 2023 (Table S1). Peak nights occurred in September for autumn and May for spring (Figure 2). The busiest night was 27–28 September 2021 with 2,703 NFCs, and the busiest hour was 05h02–06h02 on 29 September 2021 with 1,012 NFCs.
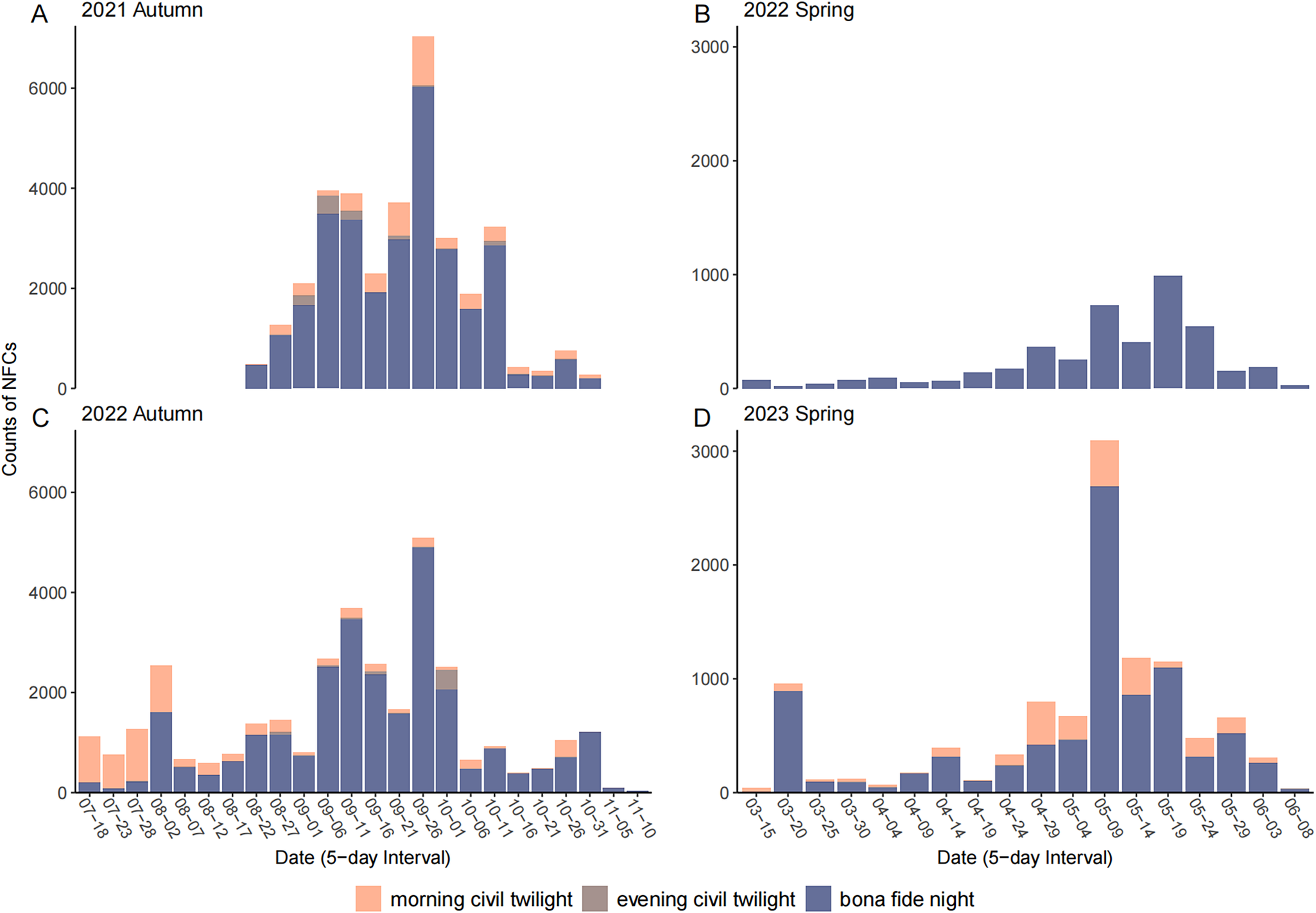
Figure 2. Counts of nocturnal flight calls (NFCs) for each five-day period in (A) autumn 2021, (B) spring 2022, (C) autumn 2022, and (D) spring 2023. The various night phases are shown in different colours: evening civil twilight (i.e. from sunset to civil dusk); morning civil twilight (i.e. from civil dawn to sunrise); bona fide night (i.e. from civil dusk to civil dawn).
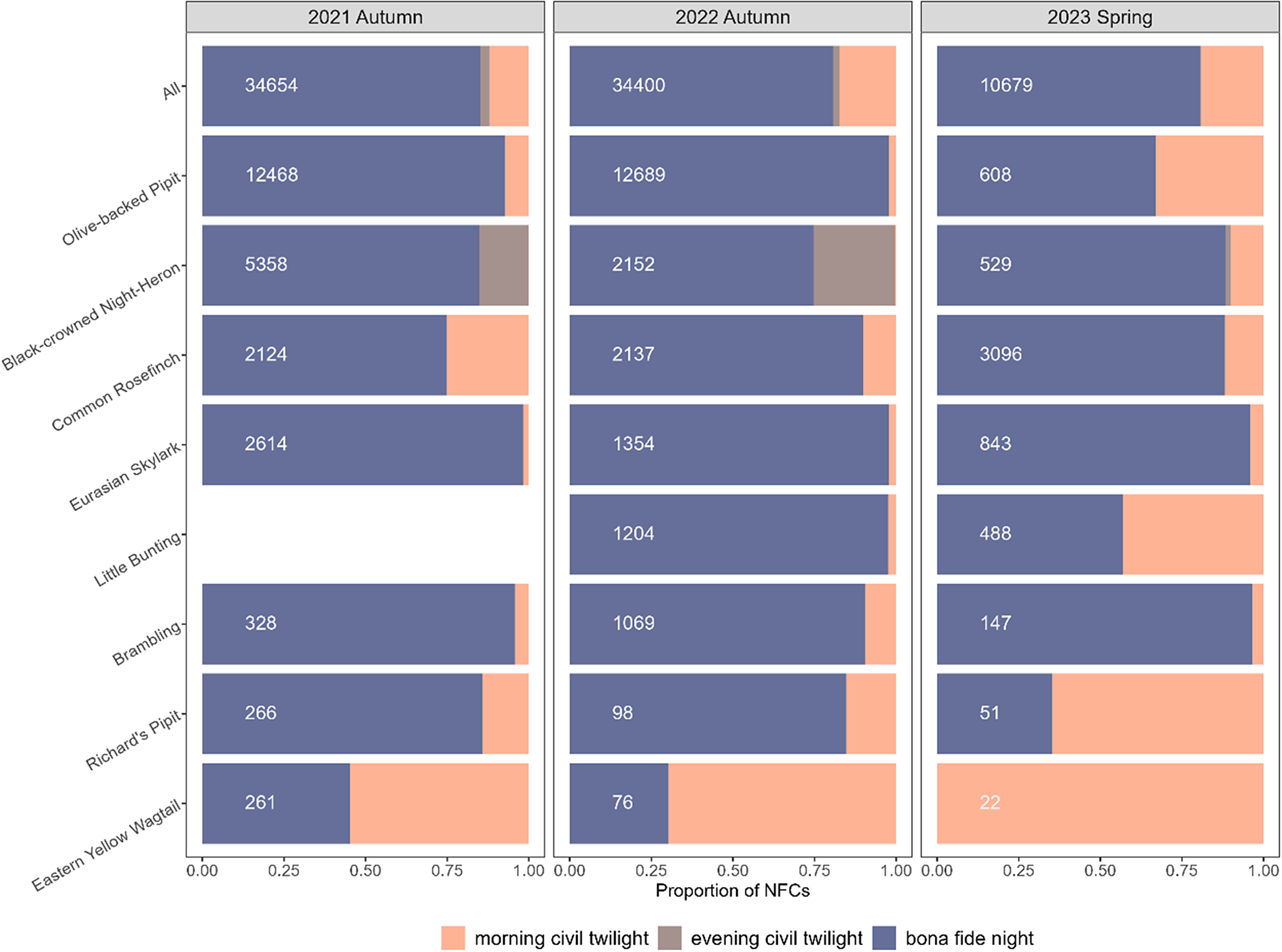
Figure 3. The proportion of nocturnal flight calls (NFCs) during various night phases for each migration season, displayed for all species combined and select species, including five most frequently recorded species (top) and three species commonly considered as diurnal migrants (bottom). The night phases distinguished include evening civil twilight (i.e. from sunset to civil dusk), morning civil twilight (i.e. from civil dawn to sunrise), and bona fide night (i.e. from civil dusk to civil dawn). The white number for each species indicates its total absolute number of NFCs during a given migration season. This figure does not include spring 2022, because recordings from this year did not include civil twilight hours. For Little Bunting Emberiza pusilla, we did not count the number of their calls in autumn 2021 and spring 2022 (they were labelled as Emberiza sp.); we thus had data only for autumn 2022 and spring 2023.
Olive-backed Pipit Anthus hodgsoni (26,158 NFCs), Black-crowned Night-heron Nycticorax nycticorax (8,464 NFCs), and Common Rosefinch Carpodacus erythrinus (7,843 NFCs) were the most commonly recorded species among identifiable NFCs (Figure 3, Table S1). This NFC abundance pattern persisted even if we excluded the civil twilight hours (Figure 3, Table S1). Other notable species included a single Grey-tailed Tattler Tringa brevipes and at least two Little Whimbrels Numenius minutus, both of which are rare passage migrants in Beijing. In addition, several threatened species were recorded, including a “Critically Endangered” Yellow-breasted Bunting Emberiza aureola, a “Vulnerable” Relict Gull Ichthyaetus relictus, several “Vulnerable” Black-capped Kingfishers Halcyon pileata, and several “Vulnerable” Rustic Buntings Emberiza rustica (IUCN 2023). The migratory birds recorded exhibited diverse habitat associations, with some species relying exclusively on a single habitat type (Figure S1).
We found significant among-season differences in all four weather features assessed: compared with autumn, spring seasons were generally less humid, and had higher wind speeds, lower temperatures, and lower air pressure (ANOVA: P <0.001; see Figure S2 for results of the multiple comparison). While we found no significant difference in the loudness of background noise among recordings from autumn 2021, autumn 2022, and spring 2023, recordings from spring 2022 were slightly less noisy compared with the other seasons (ANOVA: P = 0.001; see Figure S3 for results of the multiple comparison).
For the 14 species or species groups for which we compared migratory intensity as informed by the NFC recordings versus citizen science observation data, the temporal patterns of migratory intensity were generally consistent between the two data sources (Figure 4, Figure S4; see Table S2 for the numbers of checklists from the eBird and Birdreport data sets). Nonetheless, we found some notable inconsistencies with regard to the date range of migration season and peak dates, particularly for the flycatcher group Muscicapidae spp. during the autumn migration: our NFC recordings revealed a migration intensity peak in late summer for this group that was absent from the citizen science observation data (Figure 4F). This inconsistency for the flycatcher group persisted even if we removed the civil twilight hours – in fact, only 3.4% (14) of flycatcher NFCs were uttered during the civil twilight hours (Table S1).
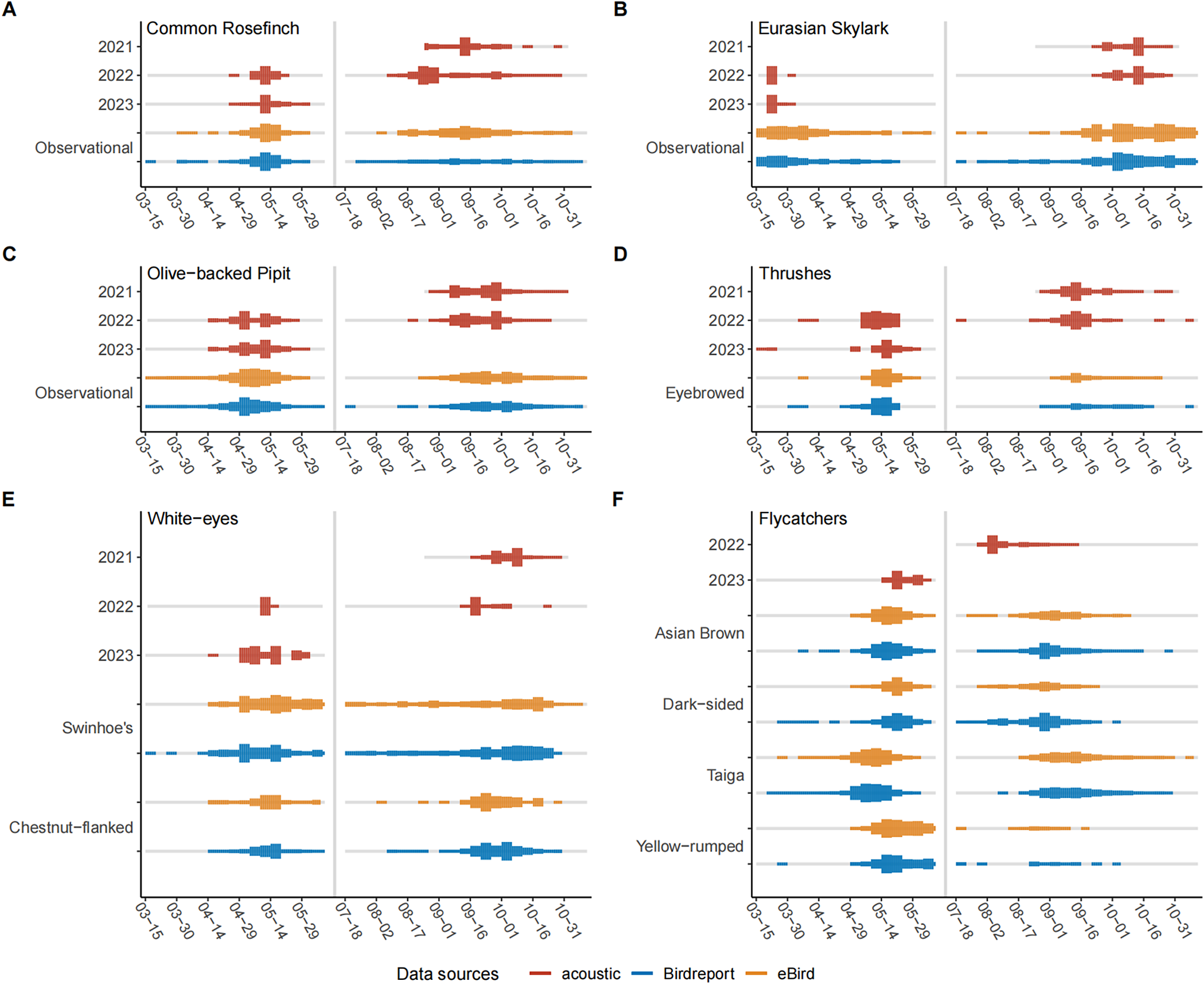
Figure 4. Temporal patterns of migratory phenology of select species or species groups that had ≥150 NFCs over at least one migration season, based on nocturnal flight call (NFC) monitoring and citizen science observations, including (A) Common Rosefinch Carpodacus erythrinus, (B) Eurasian Skylark Alauda arvensis, (C) Olive-backed Pipit Anthus hodgsoni, (D) Turdidae spp. (thrushes), (E) Zosterops spp. (white-eyes), and (F) Muscicapidae spp. (flycatchers). Line width indicates the rescaled number of calls or checklists, aggregated over five-day periods. Line colour indicates data resource, with grey colour representing zero counts. For Muscicapidae spp., we did not count the number of their calls in autumn 2021 and spring 2022; we thus had data only for autumn 2022 and spring 2023.
Discussion
Our study provided valuable insights into the nocturnal bird migration on the EAAF, highlighting the diversity and abundance of vocal, nocturnally migrating birds that pass through Beijing and their diverse habitat associations. This is the first comprehensive bioacoustic study of the flight calls of nocturnally migrating birds in China and in this migration system. Our findings also showed that NFCs provided additional information on species’ migration phenology in comparison with citizen science observation data, providing a valuable complement to the growing body of information provided by such observations.
It is worth noting that the total number of NFCs recorded in the spring migration season of 2022 was <13% of those recorded in the autumn of 2021 (<20% after adjusting for the slightly longer recording times of the latter), and the total number of NFCs recorded in the spring migration season of 2023 was around 31% of those recorded in the autumn of 2022, even though the number of species identified was only slightly smaller in the spring (Table S1). This difference is consistent with some similar studies from other flyways (e.g. Farnsworth Reference Farnsworth, Gauthreaux and van Blaricom2004; Heiss Reference Heiss2018; Horton et al. Reference Horton, Shriver and Buler2015a), and has four possible reasons. First, because of the many juveniles that undertake their first autumn migration but that may not survive that journey or their first winter (Latta and Faaborg Reference Latta and Faaborg2002; Marra and Holmes Reference Marra and Holmes2001), the number of migratory individuals in the spring tends to be much lower than in the autumn, as evidenced by the 20–40% lower spring biomass of migratory birds estimated by radar imagery in North America (Dokter et al. Reference Dokter, Farnsworth, Fink, Ruiz-Gutierrez, Hochachka and La Sorte2018). Second, juvenile individuals on the autumn migration likely vocalise more frequently than adults that make up the spring migration (Farnsworth Reference Farnsworth2005), possibly linked to their lack of migration experience and the need for increased communications with other individuals (e.g. a greater need for information in situations where disorientation may be occurring). Third, individuals on spring migration may exhibit higher flight altitudes than in autumn (Liechti et al. Reference Liechti, Bauer, Dhanjal-Adams, Emmenegger, Zehtindjiev and Hahn2018), rendering our recorder less capable of picking up their flight calls in the spring. Finally, the migration routes of migratory birds may not be the same between spring and autumn (e.g. North America: Stanley et al. Reference Stanley, MacPherson, Fraser, McKinnon and Stutchbury2012; Europe: Schmaljohann et al. Reference Schmaljohann, Buchmann, Fox and Bairlein2012; East Asia: Heim et al. Reference Heim, Pedersen, Heim, Kamp, Smirenski and Thomas2018). It is therefore possible that for some species, there are inherently (many) fewer individuals passing through Beijing in the spring than in the autumn.
In our study, only a small portion of the NFCs recorded were from the civil twilight hours. This is in contrast with previous findings that these hours contribute a major proportion of NFCs during migration seasons (Heiss Reference Heiss2018). This difference may be linked to three factors. First, the stopover-to-passage ratio of nocturnal migrants at our study site may be generally low. Therefore, relative to bona fide night and potentially strongly related to birds passing over, calls may be less concentrated in civil twilight hours that are potentially strongly related to bird departure or landing. Second, birds’ flight altitudes may be lower at our study site than in the case of Heiss (Reference Heiss2018), which would make night-time NFCs easier to capture at our study site. Third, there may be fewer diurnal migrants calling during civil twilight hours at our study site, related to possible differences in individual number, calling behaviour, and/or timing of departure/landing. Notably, the study site of Heiss (Reference Heiss2018) is a crucial migratory bottleneck adjacent to two ecological barriers (Besh Barmag in Azerbaijan), and it is conceivable that birds’ migration strategies may be considerably different from birds passing through our study site.
An added value of NFC monitoring is the documentation of species engaged in nocturnal migration. There is presently no comprehensive list of species that migrate at night on the EAAF, and with each recording of NFC comes the opportunity to document nocturnal migratory behaviours. Of particular interest is the large number of calls our study recorded of the Olive-backed Pipit. This species is a member of a diverse radiation of pipits and wagtails, in the family Motacillidae, many members of which have long been considered as diurnal migrants (e.g. Badyaev et al. Reference Badyaev, Kessel, Gibson, del Hoyo, Collar and Billerman2020; Hendricks and Verbeek Reference Hendricks, Verbeek and Billerman2020). However, our findings indicate that this is unlikely to be entirely true – with the caveat that our recording of NFCs could not differentiate migrating individuals from those making local movements or roosting nearby: large numbers of NFCs were recorded from species in this family, including notably the Olive-backed Pipit but also Richard’s Pipit Anthus richardi and Eastern Yellow Wagtail Motacilla tschutschensis (Figure 3, Table S1). Similarly, finches, like the Brambling Fringilla montifringilla (Clement and Arkhipov Reference Clement, Arkhipov, del Hoyo, Elliott, Sargatal, Christie and de Juana2020), as well as other families of migrants may be best known from diurnal movements but clearly have significant nocturnal components to their migratory ecology (Figure 3). To obtain more understanding of these aspects of their behavioural and migratory ecology, monitoring using NFCs provides an essential tool.
The large number and diverse habitat associations of migratory birds recorded to pass through Beijing at night underscores the conservation importance of managing habitat, urban lighting, and building design in and around Beijing for the safe stopover and passage of these birds. To be clear, our data do not themselves indicate stopover, but citizen science observation data from Beijing suggest that these and other birds do stop and refuel in a wide range of habitats, including woodlands but also wetlands, grasslands, shrublands, and reedbeds. Notably, several migratory landbird species from which we recorded NFCs have strong associations with non-woodland habitats (Figure S1), including the Critically Endangered Yellow-breasted Bunting that is mainly found in reedbeds and grasslands during migration (Copete and Sharpe Reference Copete, Sharpe, del Hoyo, Elliott, Sargatal, Christie and de Juana2020). Such diverse habitat needs mean that protecting and restoring these habitats is of direct relevance to supporting the migration of these birds, and that efforts narrowly targeted at tree cover would fail to benefit and may even harm many species.
Furthermore, numerous studies from other flyways have demonstrated the negative impacts of light pollution on migratory birds, including disorientation, improper habitat selection, and fatal collisions (Horton et al. Reference Horton, Buler, Anderson, Burt, Collins and Dokter2023; McLaren et al. Reference McLaren, Buler, Schreckengost, Smolinsky, Boone and van Loon2018; Van Doren et al. Reference Van Doren, Horton, Dokter, Klinck, Elbin and Farnsworth2017; Winger et al. Reference Winger, Weeks, Farnsworth, Jones, Hennen and Willard2019), the latter worsened by the high densities of buildings with glass exteriors in urban centres (Cusa et al. Reference Cusa, Jackson and Mesure2015; Loss et al. Reference Loss, Will, Loss and Marra2014). Although data are not yet widely available for the EAAF (Shi et al. Reference Shi, Liao, Zhu and Li2022), these impacts are likely applicable to birds on this flyway. Given existing knowledge and experience from other flyways (Loss et al. Reference Loss, Li, Horn, Mesure, Zhu and Brys2023; Van Doren et al. Reference Van Doren, Willard, Hennen, Horton, Stuber and Sheldon2021), and particularly given the fact that many major population centres lie on the EAAF, Beijing and other major cities should proactively reduce light pollution and building collisions, including by minimising unnecessary outdoor lighting, promoting lights-out initiatives during peak migration periods, and adopting bird-friendly building designs (Loss et al. Reference Loss, Li, Horn, Mesure, Zhu and Brys2023; Shi et al. Reference Shi, Liao, Zhu and Li2022).
The discrepancy in migration temporal patterns between our NFC recordings and citizen science observation data may reflect an inherent difference in the information captured by each data source. Whereas citizen science birdwatching is mostly related to individuals making stopovers on the ground and happens primarily during diurnal periods, NFC recording relates to those flying over at night in active migration. These two pools of individuals are not necessarily the same, and for the small number of individuals that were recorded in both data sets, the behaviours at the time of observation are likely distinctly different. In both cases, the discrepancy may reflect a potential bias and pitfall in the temporal coverage of citizen science observations: birdwatching activities in and around Beijing appear to be diminished in late summer, as evidenced by the relatively small number of checklists in August compared with the period between September and November (Figure S5). This diminished observation is likely linked to the hot weather and perceived low avian diversity during that time, and it can introduce unknown biases to describing the migratory bird community. In all, NFC recording can complement citizen science in obtaining insights on the intensity and phenology of migration (Van Doren et al. Reference Van Doren, Lostanlen, Cramer, Salamon, Dokter and Kelling2023).
In addition to the complementary relationship between acoustic and observational data, there is a primary opportunity to link up acoustic monitoring and radar surveillance of migrating birds. Recent publications have highlighted the value and importance of Chinese weather surveillance radar data for monitoring bird migration (Hu et al. Reference Hu, Cui, Wang, Long, Ma and Wu2019; Mao et al. Reference Mao, Hu, Wang, Cui, Wang and Kou2023; Shi et al. Reference Shi, Hu, Soderholm, Chapman, Mao and Cui2023); such monitoring is increasingly common and powerful in other parts of the world with existing radar networks (e.g. USA: Dokter et al. Reference Dokter, Farnsworth, Fink, Ruiz-Gutierrez, Hochachka and La Sorte2018; Gauthreaux et al. Reference Gauthreaux, Belser, van Blaricom, Berthold, Gwinner and Sonnenschein2003; Horton et al. Reference Horton, Van Doren, La Sorte, Cohen, Clipp and Buler2019; Europe: Nilsson et al. Reference Nilsson, Dokter, Verlinden, Shamoun-Baranes, Schmid and Desmet2019). Radar data can provide accurate estimates of migrant densities, while acoustic data can characterise nocturnal migration at the species level, and is well-suited for areas outside radar coverage, or where local-scale monitoring is of particular interest. Recent studies have illuminated the enormous potential for integrating radar, acoustic, and eBird data (Van Doren et al. Reference Van Doren, Lostanlen, Cramer, Salamon, Dokter and Kelling2023), as founded on earlier studies recognising the importance of documenting and understanding acoustic and radar relationships (e.g. Farnsworth et al. Reference Farnsworth, Gauthreaux and van Blaricom2004; Larkin et al. Reference Larkin, Evans and Diehl2002).
Two important caveats should be considered in interpreting findings from our study. First, the number of NFCs we recorded was likely confounded by weather conditions, which can influence call detectability in complex ways (Horton et al. Reference Horton, Stepanian, Wainwright and Tegeler2015b). The fact that weather conditions did differ among the four seasons our study covered (Figure S2) may be one reason for the seasonal differences in the total number of NFCs recorded, but this possibility is difficult to assess – even qualitatively – in the context of our study. Note that in contrast, background noise did not differ much among our study seasons (Figure S3), therefore was unlikely to have confounded our findings pertaining to NFC numbers. Second, we obtained all our recordings from a single site, rendering our findings potentially vulnerable to the influence of random events, especially busy hours related to weather conditions and/or the passage of large flocks. Such idiosyncrasy may have been involved to some extent in the seasonal and annual patterns we found of NFC numbers, as well as the difference in migratory phenology we detected between NFC recordings and citizen science observation data. More robust sampling designs involving multiple sites would be necessary to overcome the above caveats, and should be attempted in future studies.
Our study was, by design, a proof-of-concept based on available and accessible equipment and processing methods, but even with this single, simple deployment, we have demonstrated that it is feasible to identify species, count calls, and describe the migratory bird community and its phenology based on audio recordings for a specific location. Owing to the lack of an existing NFC library for the EAAF, we were not able to use automated methods for recording data extraction; however, as data accumulate, automated identification algorithms can be applied to these recordings to dramatically speed up data extraction (Gillings and Scott Reference Gillings and Scott2021; Van Doren et al. Reference Van Doren, Lostanlen, Cramer, Salamon, Dokter and Kelling2023, Reference Van Doren, Farnsworth, Stone, Osterhaus, Drucker and Van Horn2024), and potentially allow real-time monitoring. Given that, it would be highly feasible to apply this method on a much larger scale on the EAAF to aid the research, monitoring, and conservation of migratory birds.
Acknowledgements
The authors thank the Asian Infrastructure Investment Bank for support in setting up and maintaining the recorder, especially Alberto Ninio, Zeyu Li, Yaying Du, and Bo Yan, as well as those who helped with the attempt to set up more recorders elsewhere in Beijing. The authors would also like to express sincere gratitude to all the birders and wildlife recordists who have helped with call identifications, including Jonas Buddemeier, Geoff Carey, David Darrell-Lambert, James Eaton, Paul Holt, Juefei Li, James Lidster, Magnus Robb, Seán Ronayne, Joost van Bruggen, and Stanislas Wroza. The authors further thank members of the ConservationEE research group at Peking University and Tianhao Zhao from the University of Groningen for helpful discussions, Benjamin Van Doren from the Cornell Lab of Ornithology and University of Illinois Urbana-Champaign for valuable advice, as well as Simon Gillings and an anonymous reviewer for their thoughtful and constructive comments.
Supplementary material
The supplementary material for this article can be found at http://doi.org/10.1017/S0959270924000121.