Dairy production has been a crucial part of agricultural and food systems around the world for centuries. There is an increasing need to better understand and quantify the impact of worldwide dairy systems on climate change as dairy production emits greenhouse gases, such as methane (CH4) and nitrous oxide (N2O) (FAO and GDP, 2018). Enteric CH4 production accounts for nearly 60% of GHG emissions from dairy cattle (FAO and GDP, 2018), and greenhouse gas emissions represent a large inefficiency in dairy production because these gases represent a loss of energy and nitrogen (a fundamental component of protein) from the dairy cattle, which otherwise can be used for production gains (Eckard and Clark, Reference Eckard and Clark2020). Hence, quantifying and managing GHG emissions from dairy cattle is essential to support the sustainable development of dairying. Moreover, accurate quantification will guide the setting of future national and international policies to meet the goals set in the Paris Agreement 2015 by 195 countries (FAO and GDP, 2018).
Dairy sectors in developing countries are dominated by smallholder dairy production systems. In these systems, farming households tend to keep 2–5 animals per herd (Staal et al., Reference Staal, Ahuja, Hemme and Sharma2016) and in the majority of cases are an integrated crop-livestock farming system, where animal feeding is made up of crop residues in combination with a cut and carry system for fodders (in more resource rich areas) through to free ranging systems (in resource poor areas). These systems tend to rely on family labour and produce milk for both household consumption and, where possible, sale into the market. In many cases, milk in developing countries comes from these smallholder systems. For instance, 85% of total Kenya milk production comes from smallholders (Gerosa and Skoet, Reference Gerosa, Skoet, Muehlhoff, Bennett and McMahon2013) and in Pakistan 95% of milk consumed is from local and unpackaged milk sources produced by smallholder farmers (Zia et al., Reference Zia, Mahmood and Ali2011). Intensification of the dairy system is common in developing countries as a response to increased demand for milk. However, traditional smallholder systems still remain dominant (Staal et al., Reference Staal, Ahuja, Hemme and Sharma2016). Developing countries contribute to a large proportion of global milk production. For instance, Asia, which is primarily made up of developing countries, accounts for 42% (360 million tonnes) of the world's milk production (FAO, 2019). Many of the developing countries' dairy research programmes and projects have little capacity for quantifying GHG emissions due to resource and expertise limitations. Thus, the main goal of this Research Reflection was to explore challenges and opportunities for quantifying GHG through dairy research in developing countries.
Importance of dairy production for food security, livelihood and economy in developing countries
Global demand for food is expected to more than double by 2050 as a result of human population growth and an increase in living standards, particularly in developing countries (Rojas-Downing et al., Reference Rojas-Downing, Nejadhashemi, Harrigan and Woznicki2017). Demand for milk is expected to rise by 48% from 2005 to 2050 (Alexandratos and Bruinsma, Reference Alexandratos and Bruinsma2012), with 14% of the world's population benefiting from income generated from dairy production (Hemme and Otte, Reference Hemme and Otte2010). This proportion increases dramatically in developing country dairy sectors, where more than 50% of the world's milk-producing cattle are located (FAOSTAT, 2018). Developing countries are increasingly demanding dairy products, while developed countries are traditionally high in dairy product consumption (Gerber et al., Reference Gerber, Steinfeld, Henderson, Mottet, Opio, Dijkman, Falcucci and Tempio2013).
Dairy production is often more than just income generation for rural farming families. It contributes to livelihoods, food security and ultimately helps to achieve a number of the United Nations Sustainable Development Goals in the developing countries (Reisinger and Clark, Reference Reisinger and Clark2018). Since dairying plays an important role in economic and social wellbeing in developing countries, maintaining sustainable dairy production is crucial. However, expansion of these dairy production systems may negatively impact their environmental sustainability, largely due to GHG emissions from dairy production systems. Therefore, quantifying and mitigating GHG emissions from dairy cattle is an essential component to support the sustainable development of dairying in developing countries.
Importance of understanding and quantifying greenhouse gas emissions from dairy production through research in developing countries
According to Tubiello (Reference Tubiello2011), three-quarters of global agricultural GHG emissions are produced by developing countries. The livestock industry is a key part of global agriculture production and it contributes to about 15% of global anthropogenic emissions. From this, dairy cattle production accounts for approximately 30% of the livestock sector's emissions globally (Gerber et al., Reference Gerber, Steinfeld, Henderson, Mottet, Opio, Dijkman, Falcucci and Tempio2013). In 2015, dairy production added 1,711.8 million tonnes of carbon dioxide equivalent (CO₂e) GHG to the atmosphere (FAO and GDP, 2018).
Emission can be expressed as both an absolute emission (per hectare, per cow) and emission intensity (emission per unit of product) term (Reisinger and Ledgard, Reference Reisinger and Ledgard2013). The least productive countries may have the highest emission intensities (per unit of milk). However, there may be a low emission per hectare depending on the stocking rate and relatively low GHG emission per cow. The trade-off between these metrics can be complicated. For example, Eckard et al. (Reference Eckard, Grainger and De Klein2010) modelled and compared the pasture-based dairy systems in terms of low-quality and high-quality diets by adjusting the stocking rates using the DairyMod, a mechanistic whole-farm systems model. Their analysis showed that feeding high-quality pasture reduced emission intensity (kg CO2e per litre milk) by 19%, however, emission per hectare increased by 26% due to the higher stocking rates compared to low quality pasture feeding. This result shows the importance of having different metrics to quantifying emissions as it gives a different dimension of the issue. Further, these metrics are important for different policy contexts. For example, absolute emission can be used for determining the contribution of a country to gross emission, while emission intensity is more useful in benchmarking production efficiency between systems, regions and countries (Reisinger and Ledgard, Reference Reisinger and Ledgard2013).
It is interesting to note that North American and South Asian dairy cattle produced 116.6 kg CH4 and 9867 kg milk per head per year and 62.1 kg CH4 and 1388 kg milk per head per year, respectively (FAO and GDP, 2018). These values indicate a major difference in their GHG emission rates and milk production performances due to production system differences. Similarly, these values highlight the considerable differences in GHG emission intensities (emission per unit of product) between developed (North American) and developing countries (South Asian). Further, when it comes to emission intensity measures, a recent study showed that developed country dairy production had a lower emission intensity (averaged 1.4 kg CO2e per kg fat-and protein- corrected milk (FPCM)) compared with developing country dairy production (averaged 5.4 CO2e per kg FPCM) (FAO and GDP, 2018).
An important consideration in the developing country context is that dairy cattle in these systems play multiple functions on the smallholder farms. In these systems, dairy cattle are not just a source of milk, but they are also a source of draught power, store of capital, insurance for crop failure and provide nutrient cycling for cropping (Tricarico et al., Reference Tricarico, Kebreab and Wattiaux2020). This multifunctional nature of the contribution needs to be taken into account in determining their actual emissions contribution. For instance, a case-study of mixed smallholder milk production systems conducted in Kenya emphasized that including multiple functions of dairy cattle has a strong impact on estimation outcomes of emission intensity of dairying (Weiler et al., Reference Weiler, Udo, Viets, Crane and De Boer2014).
Increasing productivity has a risk of reducing some of the non-food use of dairying, for instance, draught power. However, the benefit as a store of capital, insurance for crop failure and nutrients for cropping will still apply. Increasing productivity could bring more revenue for the farmers and will increase the benefit of insurance for crop failure, as it will provide more economic opportunities to ensure the improved livelihood of farmers. Further, milk will bring more benefits than the non-food uses for farm families and the rural communities, as it fulfils the human nutrition requirement by providing high-quality protein and other micronutrients. For example, the deficit for milk in Ethiopia is approximately 4.5 billion litres per year (Tricarico et al., Reference Tricarico, Kebreab and Wattiaux2020). This can be addressed by increasing the productivity of the systems, which will ultimately reduce the GHG emission intensity of the system as well.
Currently, many developing countries use default Intergovernmental Panel on Climate Change (IPCC) Tier 1 method to estimate the GHG emissions, which adopts a fixed emission value/factor per animal as there is no local available data to practice Tier 2 calculations. This leads to incorrect estimations of emissions, as Tier 1 method does not account for local livestock factors such as milk production, feed quality and environmental conditions. A recent study on CH4 emissions from selected African cattle production showed approximately 40% overestimation of emission when IPCC Tier 1 method was used than using method considered dairy cattle intake (Goopy et al., Reference Goopy, Onyango, Dickhoefer and Butterbach-Bahl2018). This demonstrates clearly the need to more accurately measure and/or estimate GHG emissions from dairy cattle in developing countries. Further, lack of activity data (e.g. animal number and information about different dairy production systems in developing countries) is another major problem associated with the estimation of GHG emissions. Therefore, developing countries use other generalized data sources, for instance, FAOSTAT. This will lead to over/underestimation of GHG emissions. Therefore, more specific data sources are required to fill these activity data gaps to support adopting higher inventories in developing countries.
Overall, accurately quantifying GHG emissions from dairy cattle through more research in developing countries, across a range of relevant production systems, is important for identifying GHG emissions by size and source. This in turn; (1) allows scientists to explore locally relevant GHG emissions management and mitigation strategies, (2) provides evidence for producers or the dairy industry to trade in carbon credits on voluntary markets, providing the potential for income diversification and (3) supports the transparent process of reaching and reporting nationally determined contribution obligations set in the Paris Agreement (IIED, 2019).
There are a number of management practices which can be implemented for mitigating GHG emission and/or GHG emissions intensity. These include nutritional intervention, herd size, manure management, housing systems and energy efficiency (including renewables) (Grossi et al., Reference Grossi, Goglio, Vitali and Williams2019). Mitigation potential of GHG emissions using these strategies is highly diverse among farms and regions depending on different factors. For example, diversity of the production systems, environmental conditions, availability of the technology, economic incentives and institutional frameworks influence the degree of mitigation possible (FAO, Reference Gerber, Vellinga, Opio, Henderson and Steinfeld2010). However, while dairy systems in developing countries have potential to improve production and efficiency, it is not as simple as adopting those technologies and practices used in developed countries, where evidence is mounting that these more intensive systems may not be environmentally sustainable (Weiler et al., Reference Weiler, Udo, Viets, Crane and De Boer2014). Therefore, greater investment in dairy research in developing countries is required to address these complex situations and build more sustainable systems.
Challenges for quantifying greenhouse gas emissions through dairy research in developing countries
During the last few decades, quantifying and managing environmental impacts has been recognized as an important part of dairy research (Cheng, Reference Cheng2020). However, the amount of research of this sort that is funded in developing countries is limited compared to that within developed countries. Such differences are likely a reflection of food security being a major pressing priority in many of the developing countries, but also that government funds for research in developing countries are generally at a lower level than in developed countries (Gaffney et al., Reference Gaffney, Challender, Califf and Harden2019). Further, lack of expertise and facilities are two major constraints associated with quantifying GHG emissions through dairy research in developing countries.
There are a number of methods developed for quantifying GHG emissions from dairy cattle under research conditions. For example, enteric CH4 emissions from dairy cattle can be measured by respiration chamber, sulphur hexafluoride tracer technique, ventilated hood /face mask and using the Greenfeed system (Hammond et al., Reference Hammond, Crompton, Bannink, Dijkstra, Yáñez-Ruiz, O'Kiely, Kebreab, Eugène, Yu, Shingfield and Schwarm2016; Zhao et al., Reference Zhao, Nan, Yang, Zheng, Jiang and Xiong2020). However, all these methods require substantial facilities and expertise to accurately quantify CH4 emissions. While respiration chambers are regarded as a gold standard for CH4 measurements, the system is costly to build and operate and as a result has historically only been used in developed countries (e.g. New Zealand, Australia, Belgium, Denmark and Germany: Pinares-Patiño and Waghorn, Reference Pinares-Patiño and Waghorn2018), although chambers are now available in Brazil, China and Kenya.
The lack of relevant expertise and facilities in developing countries is also evident in a lack of publications in this area. The six countries with the largest dairy populations are India, Brazil, Sudan, Pakistan, China and Ethiopia. These countries account for nearly 40% of the total world dairy cattle population (AHDB Dairy, 2019). Using these individual country names together with methane and dairy, a search of the PubMed database returned only 4 publications between the year 2010 and 2020. These studies were conducted in China (2), Brazil (1) and India (1). In contrast, during the same period, scientists from Australia produced 9 publications, Canada published 13 publications and a similar case was found from other major developed countries. This simple comparison is an indicator that these developing countries are not quantifying dairy cattle GHG emissions often, which is a combination of lack of research funding, relevant facilities, expertise and environmental impacts being a lower research priority.
Opportunities for quantifying greenhouse gas emissions through dairy research in developing countries
Opportunities exist to allow scientists in developing countries to measure and estimate GHG emissions from dairy cattle. Firstly, open-access publications are increasingly available online to allow these scientists to learn about the latest dairy cattle GHG emissions research. Secondly, developed countries are offering support under the Paris Agreement for capacity building, transferring technology and financial support for minimizing GHG emissions in developing countries (FAO, 2016). The Australia Centre for International Agricultural Research (ACIAR) was established to support capability building in developing countries, including GHG emissions quantification and mitigation. Thirdly, there are many international organizations, individually and collaboratively, involved in supporting the development of skills and expertise of livestock GHG emissions management, including the Global Research Alliance on Agricultural Greenhouse Gases (GRA), the International Livestock Research Institute and Food and Agriculture Organization (FAO). These are good opportunities for dairy scientists in developing countries to leverage expertise and facilities from developed countries and enhance their understandings of GHG emissions and locally appropriate mitigation options.
Adopting existing greenhouse gas emission estimation models
There is a range of approaches that can be used to estimate GHG emissions from dairy cattle, ranging from the use of emissions factors based on animal numbers (analogous to IPCC Tier 1 methods; IPCC, 2006) to mechanistic models (Tier 3) that simulate the processes involved (Rotz, Reference Rotz2018). The simple emission factor approach requires minimal data collection but may not reflect the environmental conditions well. The more complex modelling approaches require more data (e.g. rumen function, physiological stage and rate of feed passage for enteric CH4 emission estimation using process-based mechanistic models) and may provide more accurate estimates of emissions (Rotz, Reference Rotz2018). Data requirements for Tier 2 methods are intermediate between the two approaches. The Tier 2 methods use detailed information on sub-groups of animals along with estimates of feed intake based on live weight, live weight gain, milk yield, feed digestibility and other factors that reflect the local management practices (Wilkes and van Dijk, Reference Wilkes and van Dijk2018).
While there is substantial potential to draw on the existing estimation models from developed countries to quantify GHG emissions in developing countries through dairy research, selection of the suitable modelling approaches requires careful consideration of the availability of local data and experimental conditions (dietary and animal characteristics). The production systems in developed countries and developing countries are different in numerous ways such as feeding practices, climatic conditions and animal characteristics (breeds, production capacity, heat tolerance). Dairy systems in developed countries are characterized normally by larger herd size, high yielding breeds and, often, confinement-based production systems either annually or seasonally. Further, these systems rely on stored forages, purchased grains and concentrates. However, there are some developed country production systems which are more grazing-based, for example, Australia, New Zealand and Ireland. In contrast, the smallholder systems of developing countries have lower-yielding, but more locally adapted dairy cattle breeds. Dairy systems of developing countries are mostly based on locally produced grasses and crop residues (e.g. corn stover, cereal straw). For instance, in India, dairy cattle diets are commonly based on approximately 61% crop residues and green fodders, 19% of by-products and 20% concentrates (FAO, IDF and IFCN, 2014). Therefore, direct application of GHG estimation models from developed countries may lead to over/underestimation of GHG emissions (FAO, IDF and IFCN, 2014). Local research to validate and/or adapt the approaches will be helpful to increase the estimation accuracy/precision. For instance, Ribeiro et al. (Reference Ribeiro, Rodrigues, Maurício, Borges, Reis, Berchielli, Valadares Filho, Machado, Campos, Ferreira and Júnior2020) evaluated the estimation precision and accuracy of the existing enteric CH4 estimation models from the literature for the tropical conditions and recommended the model proposed by Charmley et al. (Reference Charmley, Williams, Moate, Hegarty, Herd, Oddy, Reyenga, Staunton, Anderson and Hannah2016) be used for national inventories in tropical countries based on high concordance with local data (Table 1).
Table 1. Dairy cattle enteric methane production estimation models, that include a tropical dataset
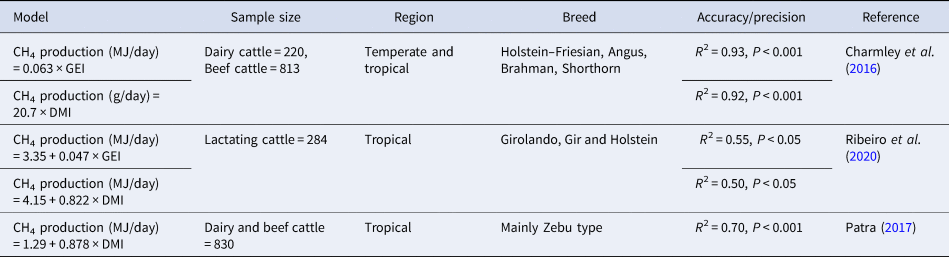
GEI, Gross energy intake (MJ per day); DMI, Dry matter intake (kg per day).
Use of proxies or markers to indicate greenhouse gas emissions
Many proxies have been developed to estimate the enteric CH4 emission. These proxies, indicators or indirect traits, vary from simple and low-cost measures to complex and expensive methods (Negussie et al., Reference Negussie, de Haas, Dehareng, Dewhurst, Dijkstra, Gengler, Morgavi, Soyeurt, van Gastelen, Yan and Biscarini2017). The ideal proxies for estimating GHG through research in developing countries should be cost-effective and require minimal expertise and facilities. Although there are plenty of proxies to estimate enteric CH4 emission, the methods that use rumen samples, faeces, urine and blood are common, but these samples are not always easy to obtain without intrusive procedures.
Negussie et al. (Reference Negussie, de Haas, Dehareng, Dewhurst, Dijkstra, Gengler, Morgavi, Soyeurt, van Gastelen, Yan and Biscarini2017) published a systematic review on potential CH4 proxies including feed intake and feeding behaviour, rumen function, metabolites and microbiome, milk production and composition, hindgut and faeces, and measurements at the level of the whole animal. According to the review, milk mid-infrared spectroscopy (MIR) and milk fatty-acid profile are promising methods to derive proxies for CH4 estimation. Notably, Bougouin et al. (Reference Bougouin, Appuhamy, Ferlay, Kebreab, Martin, Moate, Benchaar, Lund and Eugène2019) found that milk fatty-acids have better potential to estimate enteric CH4 production when combined with other variables (e.g. dry matter intake, body weight) rather than using only milk fatty-acids. However, the milk fatty-acid based method is expensive, which makes it less practical to use under many developing countries conditions.
There is an interesting perspective to consider GHG emissions model data proxies. Models rely on animal activity data, such as regional animal numbers. Sometimes regional animal numbers may not be available, but the district veterinary officer often knows how many vaccines to order each year. This may be a proxy to estimate animal numbers. Researchers could also use local information on household structures to estimate the number of cows per household in a region. Further, measuring dry matter intake to estimate enteric CH4 emission is challenging. However, animal productivity data (e.g. milk production per day), can use as a proxy to estimate feed dry matter intake of the animal. These proxies are mainly important for inventory purposes. Overall, future exploration of potential proxies to estimate GHG emissions through dairy research is an essential step to support sustainable dairy production development in developing countries.
In conclusion, the dairy industry globally faces increasing pressure to reduce GHG emissions. There is a great potential for developing countries to contribute to this reduction, as a large proportion of dairy cattle are part of the farming systems in these countries with higher emission intensities compared to dairy sectors in developed countries. The multi-functional value of dairy cattle in subsistence agriculture is important to be considered when it comes to the development of sustainable dairy production and research in developing countries. The research capacity to quantify GHG emissions in developing countries is limited. Despite this, local expertise and facilities could be supported with application of appropriate research technologies from developed countries to help understand the source and scale of GHG emissions from dairy cattle in the different farming systems.