Introduction
Invasive ticks, mosquitoes and other blood-feeding arthropods may introduce and transmit (i.e. vector) exotic pathogens for which local populations have little or no immunity. Resulting disease can range from mild to severe to fatal and can have a significant impact on human health (e.g. Zika fever, West Nile virus encephalitis), animal health (e.g. redwater fever, blue tongue virus), hasten extinction (e.g. bird malaria) and cause economic damage to agriculture, tourism and other industries (Athni et al., Reference Athni, Shocket, Couper, Nova, Caldwell, Caldwell, Childress, Childs, De Leo, Kirk, MacDonald, Olivarius, Pickel, Roberts, Winokur, Young, Cheng, Grant, Kurzner, Kyaw, Lin, Lopez, Massihpour, Olsen, Roache, Ruiz, Schultz, Shafat, Spencer, Bharti and Mordecai2021). Invasive vectors can also potentially spread existing wildlife pathogens by creating new transmission pathways, which can have significant ecological and public health implications, particularly in the context of One Health (sensu Lerner and Berg, Reference Lerner and Berg2015).
The phylum Apicomplexa includes well-known blood-borne protozoa such as Plasmodium falciparum and P. vivax, the primary agents of human malaria (Votýpka et al., Reference Votýpka, Modrý, Oborník, Šlapeta and Lukeš2016). The Apicomplexa class Piroplasmida includes Babesia, Theileria and Cytauxzoon that are primarily transmitted by hard ticks (Ixodida: Ixodidae) and can affect a wide range of hosts (Onyiche et al., Reference Onyiche, Răileanu, Fischer and Silaghi2021; Almazán et al., Reference Almazán, Scimeca, Reichard and Mosqueda2022). While piroplasms were once classified based on morphology and host associations alone, the advent of molecular methods has greatly advanced the overall understanding of the diversity of Piroplasmida (Garrett et al., Reference Garrett, Hernandez, Balsamo, Barron, Beasley, Brown, Cloherty, Farid, Gabriel, Groves, Hamer, Hill, Lewis, McManners, Nemeth, Oesterle, Ortiz, Peshock, Schnellbacher, Schott, Straif-Bourgeois and Yabsley2019). A recent analysis by Jalovecka et al. (Reference Jalovecka, Sojka, Ascencio and Schnittger2019) indicates that there are at least 10 distinct clades within Piroplasmida, with both Babesia spp. and Theileria spp. comprising polyphyletic groups in need of taxonomic revision.
Babesia spp. are broadly divided into Babesia sensu stricto and Babesia sensu lato, with the former representing a monophyletic group considered as ‘true Babesia’, distinguishable from other piroplasms by their ability to infect the reproductive organs of the tick and to be transmitted to the eggs (transovarial transmission) (Schreeg et al., Reference Schreeg, Marr, Tarigo, Cohn, Bird, Scholl, Levy, Wiegmann and Birkenheuer2016; Jalovecka et al., Reference Jalovecka, Sojka, Ascencio and Schnittger2019; Schnittger et al., Reference Schnittger, Ganzinelli, Bhoora, Omondi, Nijhof and Florin-Christensen2022). Within Babesia sensu lato, one of the best-characterized clades is the Babesia microti group, which contains the piroplasms responsible for most human babesiosis cases worldwide, especially in the northern United States (Renard and Mamoun, Reference Renard and Mamoun2021). Importantly, B. microti includes at least 2 different genetic lineages pathogenic to humans and several only known from reservoir hosts such as mice, voles and skunks (Goethert, Reference Goethert2021). Moreover, although still relatively rare, human disease caused by other Babesia species such as B. divergens in Europe, B. venatorum in Asia and Europe and B. duncani in North America has been increasingly reported (Scott and Scott, Reference Scott and Scott2018; Hong et al., Reference Hong, Kim, Song, Roh, Cho, Kim, Um, Kwak, Cho and Lee2019; Kumar et al., Reference Kumar, O'Bryan and Krause2021; Scott et al., Reference Scott, Sajid, Pascoe and Foley2021).
Rates of human babesiosis have been increasing in the USA, particularly in the northeastern states (Almazán et al., Reference Almazán, Scimeca, Reichard and Mosqueda2022; Swanson et al., Reference Swanson, Pickrel, Williamson and Montgomery2023). While those infected with Babesia may experience fever, chills, headache, muscle aches, fatigue and red or brown urine, some may not have any symptoms at all, especially if their immune systems are not compromised (Almazán et al., Reference Almazán, Scimeca, Reichard and Mosqueda2022). People may remain infected for years, and even if asymptomatic, can transmit piroplasms through blood transfusions or organ transplants (Bloch et al., Reference Bloch, Kumar and Krause2019), and transmission from infected mothers to developing fetuses has been demonstrated (Horowitz and Freeman, Reference Horowitz and Freeman2020). Piroplasmid infections are typically treated with various combinations of atovaquone, azithromycin, clindamycin and quinine; however, concerns regarding side-effects, drug resistance and drug efficacy indicate the need for development of novel treatment options (Renard and Mamoun, Reference Renard and Mamoun2021).
Bovine babesiosis caused by Babesia bigemina and Babesia bovis has long been a concern to American cattle ranchers and several Theileria species can sicken horses, cervids and bovids (Almazán et al., Reference Almazán, Scimeca, Reichard and Mosqueda2022; Osbrink et al., Reference Osbrink, Thomas, Lohmeyer and Temeyer2022). Both babesiosis and theileriosis cause significant economic losses annually to North American and Australasian agricultural industries due to reduced production, death, abortions, restrictions on animal movement and costs associated with preventive measures and treatments (Dinkel et al., Reference Dinkel, Herndon, Noh, Lahmers, Todd, Ueti, Scoles, Mason and Fry2021; Almazán et al., Reference Almazán, Scimeca, Reichard and Mosqueda2022; Osbrink et al., Reference Osbrink, Thomas, Lohmeyer and Temeyer2022; Schnittger et al., Reference Schnittger, Ganzinelli, Bhoora, Omondi, Nijhof and Florin-Christensen2022). Companion animals are also at risk, with infections of Babesia vulpes, Babesia conradae, Babesia vogeli, Babesia gibsoni and Babesia sp. Coco capable of causing mild to severe disease in dogs in the United States (Dear and Birkenheuer, Reference Dear and Birkenheuer2022).
Since the initial discovery of the invasive Asian longhorned tick (Haemaphysalis longicornis) in the United States in 2017 (Rainey et al., Reference Rainey, Occi, Robbins and Egizi2018), there have been concerns regarding the potential threats this ectoparasite may pose. In North America, as in Australasia where it expanded to in the early 20th century, H. longicornis reproduces asexually (clonally) by parthenogenesis (Schappach et al., Reference Schappach, Krell, Hornbostel and Connally2020), which underlies the ability of this species to develop large populations very quickly. In its Australasian range, H. longicornis represents a major threat to domestic livestock, heavily parasitizing large ruminants, and impeding production (Heath, Reference Heath2016).
Globally, H. longicornis is a known vector of piroplasms that infect humans, livestock and companion animals, including B. ovata, B. gibsoni, B. microti, T. uilenbergi and T. orientalis (Li et al., Reference Li, Luo, Guan, Ma, Liu, Liu, Ren, Niu, Lu, Gao, Liu, Dang, Tian, Zhang, He, Bai and Yin2009; Wu et al., Reference Wu, Cao, Zhou, Zhang, Gong and Zhou2017; Gray et al., Reference Gray, Estrada-Peña and Zintl2019; Dinkel et al., Reference Dinkel, Herndon, Noh, Lahmers, Todd, Ueti, Scoles, Mason and Fry2021; Dear and Birkenheuer, Reference Dear and Birkenheuer2022) and may also be a vector of Babesia caballi, the agent of equine babesiosis (Bautista et al., Reference Bautista, Ikadai, You, Battsetseg, Igarashi, Nagasawa and Fujisaki2001). As in Australia (Marendy et al., Reference Marendy, Baker, Emery, Rolls and Stutchbury2020), in Virginia, USA, H. longicornis has already been implicated in the transmission of the virulent Theileria orientalis Ikeda genotype that resulted in multiple cattle deaths (Thompson et al., Reference Thompson, White, Shaw, Egizi, Lahmers, Ruder and Yabsley2020; Dinkel et al., Reference Dinkel, Herndon, Noh, Lahmers, Todd, Ueti, Scoles, Mason and Fry2021).
Although humans are not favoured hosts of H. longicornis, opportunistic feeding is well documented both in the native and invasive ranges (Bickerton and Toledo, Reference Bickerton and Toledo2020; Wormser et al., Reference Wormser, McKenna, Piedmonte, Vinci, Egizi, Backenson and Falco2020). In East Asia, H. longicornis vectors severe fever with thrombocytopenia syndrome virus (SFTSV), an emerging human tick-borne disease recently reclassified as Dabie bandavirus (Liu et al., Reference Liu, Zhou, Sun, Yao, Li, Wang, Mu, Li, Yang, Gray, Cui, Yin, Fang, Yu and Cao2015; Luo et al., Reference Luo, Zhao, Wen, Zhang, Liu, Fang, Xue, Ma, Zhang, Ding, Lei and Yu2015; Li et al., Reference Li, Liu, Wu, Liu, Huang, Li, Liu, Li, Wang, Li and Liang2021). Under laboratory conditions, US lineages of H. longicornis can vector the closely related Heartland virus (Raney et al., Reference Raney, Perry and Hermance2022a) as well as Powassan virus (Raney et al., Reference Raney, Herslebs, Langohr, Stone and Hermance2022b), 2 native pathogenic viruses emergent in parts of the USA. They can also vector Rickettsia rickettsii, the causative agent of Rocky Mountain spotted fever (Stanley et al., Reference Stanley, Ford, Snellgrove, Hartzer, Smith, Krapiunaya and Levin2020). In addition, Bourbon virus has been detected from a larval pool, 2 nymphs and 1 adult field collected H. longicornis in Virginia, USA (Cumbie et al., Reference Cumbie, Trimble and Eastwood2022). While H. longicornis is currently not perceived as a major public health threat in the USA, this status may change given the enormous densities it can reach in favourable habitats (Bickerton and Toledo, Reference Bickerton and Toledo2020; Schappach et al., Reference Schappach, Krell, Hornbostel and Connally2020; González et al., Reference González, Fonseca and Toledo2023; Rochlin et al., Reference Rochlin, Egizi, Narvaez, Bonilla, Gallagher, Williams, Rainey, Price and Fonseca2023).
The objective of this study was to assess the potential role of H. longicornis as a vector of piroplasms in New Jersey (NJ), the most urbanized US state that, maybe surprisingly to many, also boasts the highest density of horses (Rankins and Malinowski, Reference Rankins and Malinowski2020).
Materials and methods
Study areas
This study was conducted in 3 sites approximately 1.2 km from each other within the Rutgers University Cook Campus in New Brunswick, NJ (please refer to Ferreira et al., Reference Ferreira, González, Milholland, Tung and Fonseca2023 for a map). Surveys for H. longicornis were initiated at these sites in 2018 when the species was first detected on a grassy area next to a goat pen (Egizi et al., Reference Egizi, Occi, Price and Fonseca2019b), a site that became known as the ‘Goat Farm’ (40.47444° N, 74.43683° W). The ‘Rutgers Gardens’ site (40.47455° N, 74.42030° W) is inside a 180-acre botanical garden, consisting of designed gardens, plant collections and natural habitats. Finally, the ‘University Inn’ site (40.48413 N, 74.43051 W) is a meadow and forested park behind the Rutgers University Inn & Conference Center. At all sites, local forest is dominated by oak and maple trees and huckleberry and blueberry shrubs (Breden et al., Reference Breden, Alger, Strakosch Walz and Windisch2001), with grassy ecotones.
Tick surveillance
From June through September 2021, concomitant with surveys for ticks on mammals at the same sites, questing ticks were sampled from 50–75 m2 of the vegetation at each site (Ferreira et al., Reference Ferreira, González, Milholland, Tung and Fonseca2023). Tick sampling was performed using a white crib flannel sweep measuring 50 × 100 cm with a PVC pipe handle (Egizi et al., Reference Egizi, Occi, Price and Fonseca2019b). The sweep cloth was checked in 1–2 m intervals since H. longicornis does not attach firmly to the flannel and often drops off over longer intervals (Bickerton et al., Reference Bickerton, McSorley and Toledo2021). Ticks were collected from both sides of the sweep and identified morphologically in the laboratory to the species level using a stereomicroscope (Leica S8 APO, Leica Microsystems, Deerfield, IL, USA) following appropriate taxonomical keys (Keirans and Litwak, Reference Keirans and Litwak1989; Egizi et al., Reference Egizi, Robbins, Beati, Nava, Evans, Occi and Fonseca2019a). The larvae of H. longicornis were not stored during these surveys and were not available for pathogen testing. A few questing H. longicornis that were found partially engorged (sensu Price et al., Reference Price, Witmier, Eckert and Boyer2022) were processed separately (see section below on ‘Bloodmeal analysis of partially engorged specimens’).
DNA extraction and pathogen detection
Each tick was placed in 180 μl of Qiagen buffer ATL with 20 μl Qiagen Proteinase K (10 mg mL−1) in microfuge tubes and homogenized with a 5 mm sterile glass bead (Fisher Scientific, Waltham, MA, USA) in a TissueLyser (Qiagen Inc., Valencia, CA, USA). DNA from individual ticks was extracted using Dneasy Blood and Tissue 96-well plate kits (Qiagen Inc., Valencia, CA, USA) following the manufacturer's instructions. DNA was eluted from each column twice with 50 μl of Qiagen's elution buffer AE into separate labelled microtubes.
After reviewing the literature, primers were chosen targeting the multi copy 18S rRNA locus (Table 1) to match a broad range of piroplasm species (Casati et al., Reference Casati, Sager, Gern and Piffaretti2006) and all ticks were tested individually. To further characterize an undescribed new Babesia sp. detected, specimens positive for that Babesia were also tested using primers targeting the mitochondrial cytochrome oxidase b (cytb) locus (Table 1) shown to work across multiple Babesia species (Rajapakshage et al., Reference Rajapakshage, Yamasaki, Hwang, Sasaki, Murakami, Tamura, Lim, Nakamura, Ohta and Takiguchi2012).
Table 1. Primers used for piroplasm detection in H. longicornis

Ta = annealing temperature. The expected 18S rRNA amplicon size varies depending on the species of piroplasm from 395 to 515 bp; the expected cytochrome b amplicon size is 1009 bp.
*Instead of a specific Ta, a ‘touch-down’ approach was used starting with Ta = 54°C and decreasing the Ta by 1 degree for 4 additional cycles. This was followed by 40 additional cycles at Ta = 52°C.
The targeted loci were amplified in 20 μl reactions with Amplitaq Gold Master Mix (ThermoFisher Scientific, Waltham, MA, USA) following the manufacturer's protocol. After visualizing the amplification in a 1% agarose gel, polymerase chain reactions (PCR) were cleaned with ExoSAP-IT (ThermoFisher Scientific) and Sanger sequenced separately with both primers at Azenta Genewiz (South Plainfield, NJ, USA). The sequences were trimmed and aligned with Geneious Prime 2023.0.1 (Biomatters Inc., San Diego, CA, USA) and the consensus was used as a query in NCBI's Basic Local Alignment Search Tool, BLASTn (Altschup et al., Reference Altschup, Gish, Miller, Myers and Lipman1990).
Phylogenetic analysis
Consensus sequences were aligned to available NCBI Genbank sequences (Benson et al., Reference Benson, Cavanaugh, Clark, Karsch-Mizrachi, Lipman, Ostell and Sayers2012) representative of the major piroplasm clades (Jalovecka et al., Reference Jalovecka, Sojka, Ascencio and Schnittger2019) and the alignments were trimmed to the same size (557 bp). Maximum likelihood phylogenetic trees based on the 18S rRNA and cytb loci were constructed using IQ-TREE with 1000 ultrafast bootstrap replicates (Nguyen et al., Reference Nguyen, Schmidt, von Haeseler and Minh2015; Hoang et al., Reference Hoang, Chernomor, von Haeseler, Minh and Vinh2018). ModelFinder was used to choose the best-fitting substitution model based on Bayesian information criterion (Kalyaanamoorthy et al., Reference Kalyaanamoorthy, Minh, Wong, von Haeseler and Jermiin2017). Cardiosporidium cionae (GenBank accession number EU052685) and B. microti (GenBank accession number NC034637) were used as outgroups for the 18S rRNA and ctyb phylogenetic trees, respectively.
Statistical analysis
To determine whether there are statistically significant differences in infection rates in nymphal vs female H. longicornis, a binomial generalized linear model using the glm function in R (R Core Team, 2022) was used. To determine the correlation between sample size and infection rates, the lm function in R was used.
Bloodmeal analysis of partially engorged specimens
DNA from 2 partially engorged H. longicornis collected on 15 July 2021 (1 nymph and 1 adult) was isolated using DNeasy Blood and Tissue columns (Qiagen, Valencia, CA, USA). An extraction control was included, and all work was performed in a dedicated clean lab inside a laminar flow hood (Mystaire, Creedmor, NC, USA). Primers CytbVertR1 (5'-GGACGAGGACTATACTACGG-3' from Egizi et al., Reference Egizi, Healy and Fonseca2013) and BMF1 (5'-AAACTGCAGCCCCTCAGAATGATATTTGTCCTCA-3'), originally called H15149 (Kocher et al., Reference Kocher, Thomas, Meyer, Edwards, Paabo, Villablancatt and Wilson1989), were used to amplify a 132-nucleotide fragment in the cytochrome oxidase b locus using an annealing temperature of 55°C. A PCR product obtained from the adult H. longicornis was purified (ExoSAP-IT, Affymetrix, Santa Clara, CA, USA) then sequenced at Azenta Genewiz (South Plainfield). The sequences were trimmed and aligned with Geneious Prime 2023.0.1 (Biomatters Inc.) and the consensus was used as a query in NCBI's Basic Local Alignment Search Tool, BLASTn (Altschup et al., Reference Altschup, Gish, Miller, Myers and Lipman1990).
Results
Overall, 667 H. longicornis nymphs and adults collected from the environment were screened and evidence of piroplasm parasites was found in 18 ticks (2.7%, Table 2). Adult and nymph infection rates did not differ (infection rates of 2.4 and 2.8%, respectively, P value = 0.60). Infection rates among sampling sites reflected sample size (r 2 = 0.92, P value <0.01; using adult and nymph data separately to increase the statistical power).
Table 2. Numbers of H. longicornis nymphs and adults collected from the environment at 3 sites at Rutgers Cook campus and tested for piroplasm DNA (# Pos represents the number of ticks that were positive). Collections were started on 24 June 2021 and proceeded approximately bi-weekly until 10 September 2021 (refer to Ferreira et al., Reference Ferreira, González, Milholland, Tung and Fonseca2023 for details).

1 Babesia microti S837 (5 positive ticks)
2 Babesia sp. Coco (2 positive ticks)
3 Babesia sp. (4 positive ticks)
4 Theileria cervi (a total of 6 positive ticks)
5 Theileria sp. (1 positive ticks)
There was no evidence of piroplasm coinfections (such as double chromatogram peaks) in the positive ticks. The primers targeting the 18S rRNA gene amplified fragments ranging in size from 395 to 515 base pairs (bp) spanning the V4 hypervariable region (Cauvin et al., Reference Cauvin, Hood, Shuman, Orange, Blackburn, Sayler and Wisely2019).
The 18S rRNA fragment from a nymph collected at University Inn had a 100% pairwise identity to 5 Theileria sp. sequences (GenBank accession numbers MW008536, MW008531, MK262962, MK262963 and MK262959) that are considered ‘Type X’ or ‘divergent’ (Cauvin et al., Reference Cauvin, Hood, Shuman, Orange, Blackburn, Sayler and Wisely2019; Olafson et al., Reference Olafson, Buckmeier, May and Thomas2020). This sequence differed by 20 bp (17 mismatches and 3 deletions) from 6 other sequences obtained from H. longicornis also collected from the University Inn site. The closest match for these 6 sequences (99.8–100% pairwise identity) was a Theileria cervi type F sequence (GenBank U97054). Three of the 6 sequences, 2 from nymphs and 1 from an adult, were identical to U97054 while the remaining 3, all from nymphs, differed by 1 bp (GenBank accession numbers OR612075, OR612078, OR612080). All sequences clustered within a clade containing T. cervi and T. orientalis (Fig. 1a).
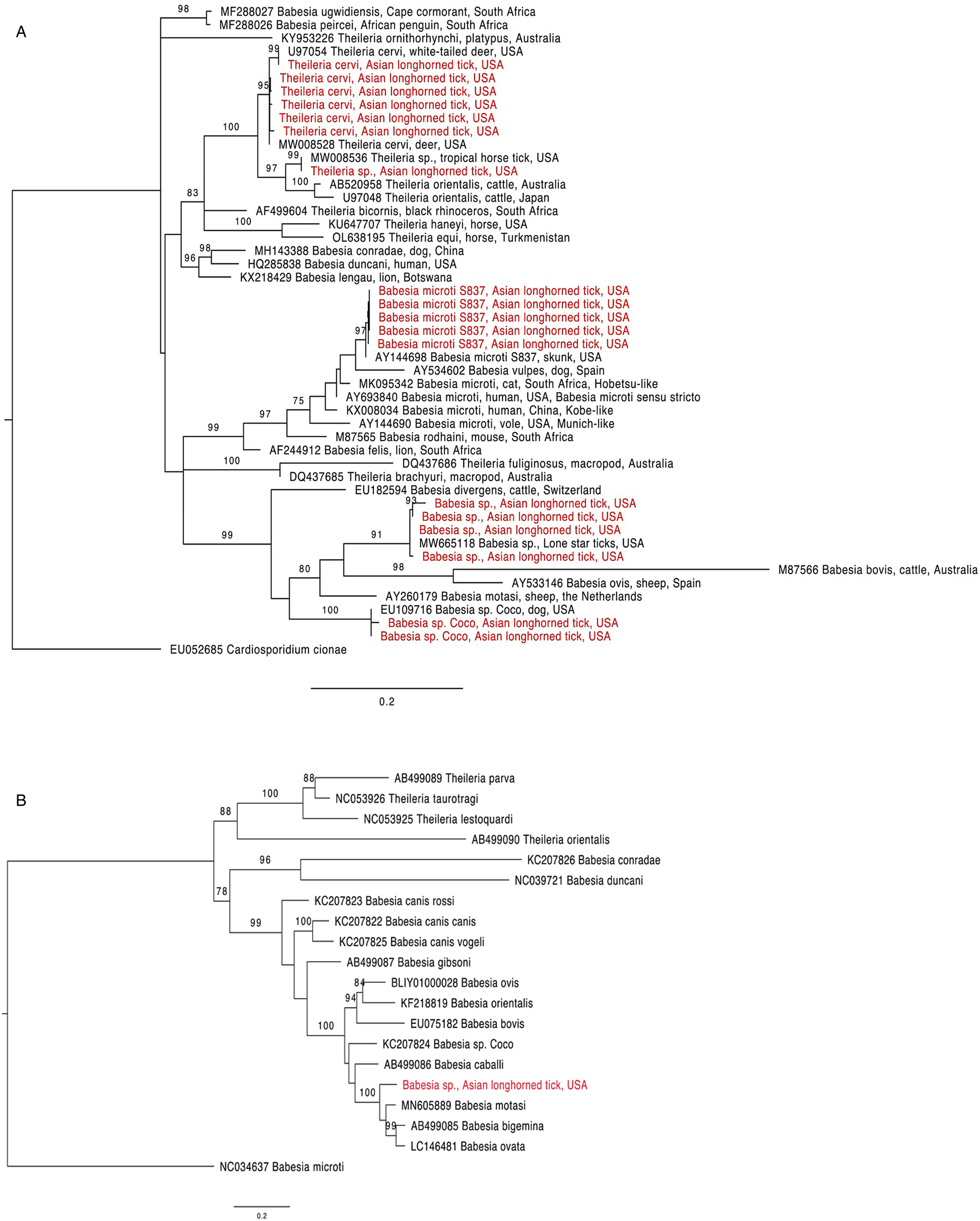
Figure 1. Phylogenetic trees with piroplasm sequences obtained from Haemaphysalis longicornis (in red). (a) Tree based on 18S rRNA locus. Constructed with TIM2 + F + I + G4 substitution model. (b) Tree based on cytochrome b locus. Constructed with K3Pu + F + I + G4 substitution model.
Two 18S rRNA sequences, one from a Goat Farm adult and the other from a University Inn nymph, were identical to a Babesia sp. isolate NYT-435 (GenBank accession number MW665118) recently sequenced from a pool of lone star ticks (Ambylomma americanum) from Staten Island, NYC (Jain et al., Reference Jain, Tagliafierro, Marques, Sanchez-Vicente, Gokden, Fallon, Mishra, Briese, Kapoor, Sameroff, Guo, Marcos, Hu, Lipkin and Tokarz2021). The sequence of the Babesia sp. isolate NYT-435 was also the closest match for 2 other sequences, 1 from another Goat Farm adult and a second University Inn nymph (99.7 and 99.5% pairwise identities with a 1 and 2 bp difference, respectively; GenBank accession numbers OR612068, OR612072, respectively). The next best match for these 4 sequences (97.9–98.2% pairwise identity) was a Babesia sp. from a white-tailed deer in Texas (GenBank accession number HQ264120). These 4 sequences clustered within the Babesia sensu stricto clade with B. bovis and Babesia ovis (Fig. 1a). From one of the specimens that were positive for the unknown Babesia, a 1009 bp DNA fragment was amplified with the cytb primers (GenBank accession number OR610156). The closest match to this sequence was Babesia motasi isolate Lintan (GenBank acc. num. MN605889) with 90.8% pairwise identity. The sequence from this H. longicornis clustered with B. motasi in the Babesia sensu stricto clade (Fig. 1b).
The 18S rRNA from 2 sequences, one from a University Inn nymph and another from a Goat Farm adult, matched Babesia sp. Coco. The sequence from the Goat Farm adult was 100% pairwise identical to Genbank EU109716, whereas the sequence from the University Inn nymph differed by 1 bp and had a 99.8% pairwise identity (GenBank accession number OR612082).
Finally, 5 18S rRNA sequences, from 2 nymphs and 1 adult from the Goat Farm and 2 nymphs from the University Inn, had a 100% pairwise identity to B. microti isolate S837 (GenBank accession number AY144698). In the phylogenetic tree, these sequences clustered with B. vulpes within the B. microti-like group (Fig. 1a; Jalovecka et al., Reference Jalovecka, Sojka, Ascencio and Schnittger2019).
A 132 bp DNA fragment was amplified and sequenced from a partially engorged adult H. longicornis and was 100% identical to a cytb fragment from white-tailed deer, Odocoileus virginianus (GenBank accession number AF535863).
Discussion
DNA sequences belonging to 3 piroplasm clades were detected in 2.7% of field-collected H. longicornis nymphs and adults in NJ, United States. Infection rates for Theileria, Babesia sensu stricto and B. microti, were 1, 0.9 and 0.8%, respectively. While these may be low piroplasm infection rates compared to studies that detected T. orientalis in 12.7% of H. longicornis in Virginia (Thompson et al., Reference Thompson, White, Shaw, Egizi, Lahmers, Ruder and Yabsley2020, Reference Thompson, White, Doub, Sharma, Frierson, Dominguez, Shaw, Weaver, Vigil, Bonilla, Ruder and Yabsley2022), those were collected from the cattle farm where the first US outbreak of T. orientalis Ikeda occurred, which would have increased the likelihood that local vectors were infected. Overall, there have been few exploratory examinations of piroplasms in H. longicornis in the United States.
This is the first report of Theileria species besides T. orientalis Ikeda in H. longicornis in the United States. Most sequences matched T. cervi type F, a piroplasm that commonly infects deer and other cervids (Cauvin et al., Reference Cauvin, Hood, Shuman, Orange, Blackburn, Sayler and Wisely2019; Olafson et al., Reference Olafson, Buckmeier, May and Thomas2020). However, 1 sequence differed in at least 20 bp from T. cervi type F and instead matched a strain denoted ‘divergent’ or ‘type X’ found in wild and farmed deer in Florida and in Anocenter nitens, the tropical horse tick, parasitizing white-tailed deer in Texas (Cauvin et al., Reference Cauvin, Hood, Shuman, Orange, Blackburn, Sayler and Wisely2019; Olafson et al., Reference Olafson, Buckmeier, May and Thomas2020). US populations of H. longicornis have often been reported feeding on white-tailed deer (Tufts et al., Reference Tufts, Goodman, Benedict, Davis, VanAcker and Diuk-Wasser2021), and finding deer DNA in a partially engorged tick supports this. These findings indicate that H. longicornis may be involved in the transmission cycle of Theileria in NJ.
This is also the first report of Babesia in questing un-engorged H. longicornis in the United States. Specifically, the phylogenetic analyses using both 18S and cytochrome b loci indicate the unknown Babesia sequences fall within the Babesia sensu stricto clade. Babesia sensu stricto (also referred to as ‘true Babesia’) are pathogens of both veterinary and medical importance capable of transovarial transmission in their tick vectors, allowing the parasite to propagate in the absence of vertebrate reservoirs (Jalovecka et al., Reference Jalovecka, Sojka, Ascencio and Schnittger2019).
Babesia sp. Coco, another ‘true Babesia’ was also detected in 2 H. longicornis. Babesia sp. Coco can be pathogenic to dogs but usually only if they are immunocompromised (Birkenheuer et al., Reference Birkenheuer, Neel, Ruslander, Levy and Breitschwerdt2004; Holman et al., Reference Holman, Backlund, Wilcox, Stone, Stricklin and Bardin2009; Sikorski et al., Reference Sikorski, Birkenheuer, Holowaychuk, McCleary-Wheeler, Davis and Littman2010; Dear and Birkenheuer, Reference Dear and Birkenheuer2022), so it is unclear whether dogs are incidental hosts or are an important reservoir species that manifest clinical disease when immunocompromised. Dogs are considered an important blood host for H. longicornis in the United States (Trout Fryxell et al., Reference Trout Fryxell, Vann, Butler, Paulsen, Chandler, Willis, Wyrosdick, Schaefer, Gerhold, Grove, Ivey, Thompson, Applegate, Sweaney, Daniels, Beaty, Balthaser, Freye, Mertins, Bonilla and Lahmers2021; Thompson et al., Reference Thompson, White, Doub, Sharma, Frierson, Dominguez, Shaw, Weaver, Vigil, Bonilla, Ruder and Yabsley2022).
Finally, this is the first report of B. microti genotype S837 in H. longicornis. This genotype is found in skunks Mephitis mephitis (Goethert, Reference Goethert2021), which are an important host for this tick species in NJ (Ferreira et al., Reference Ferreira, González, Milholland, Tung and Fonseca2023) and, critically, H. longicornis has been shown to be a competent vector of B. microti under experimental conditions (Wu et al., Reference Wu, Cao, Zhou, Zhang, Gong and Zhou2017). Of note, there is an overall lack of knowledge of the biology and epidemiology of wildlife piroplasms in the northeastern USA. In areas endemic for human babesiosis, molecular studies of wildlife piroplasms rarely employ sequencing to confirm parasite identity, although piroplasm parasites thought to only infect wildlife and/or domestic animals have recently been reported infecting humans in North America (Scott et al., Reference Scott, Sajid, Pascoe and Foley2021) and elsewhere (Hong et al., Reference Hong, Kim, Song, Roh, Cho, Kim, Um, Kwak, Cho and Lee2019).
Although the health risk of H. longicornis to US livestock was established by the outbreak of T. orientalis Ikeda in cattle in the state of Virginia (Thompson et al., Reference Thompson, White, Shaw, Egizi, Lahmers, Ruder and Yabsley2020; Dinkel et al., Reference Dinkel, Herndon, Noh, Lahmers, Todd, Ueti, Scoles, Mason and Fry2021), there is limited research regarding the veterinary and medical significance of H. longicornis in the USA, which has focused primarily on testing the ability of US specimens to transmit pathogens of known public health concern (Breuner et al., Reference Breuner, Ford, Hojgaard, Osikowicz, Parise, Rosales Rizzo, Bai, Levin, Eisen and Eisen2020; Stanley et al., Reference Stanley, Ford, Snellgrove, Hartzer, Smith, Krapiunaya and Levin2020; Raney et al., Reference Raney, Perry and Hermance2022a). The discovery of various piroplasm parasites in questing H. longicornis specimens in NJ brings forth new concerns. As a result, it becomes crucial to prioritize studies that delve into the role of H. longicornis as an actual vector for these pathogens.
Data availability statement
Nucleotide sequence data reported in this paper are available in GenBank™, EMBL and DDBJ databases under the accession numbers OR610156 and OR612065-OR612082.
Acknowledgements
We thank Nicole Wagner MS from the Rutgers Center for Vector Biology and Julia Brennan, now developing her PhD at Virginia Tech, for their technical support. We also thank Dr Glen Scoles, USDA-ARS Beltsville, for sharing his piroplasm expertise.
Author contributions
Heidi E. Herb: conceptualization, methodology, formal analysis, investigation, data curation, writing – original draft, writing – review and editing, visualization. Julia González and Francisco C. Ferreira: investigation, data curation, writing – review & editing. Dina M. Fonseca: conceptualization, methodology, resources, writing – review & editing, supervision, project administration, funding acquisition.
Financial support
This work was supported in part by a United States Department of Agriculture National Institute of Food and Agriculture Multistate Grant, NE1943, to D. M. F., a State of New Jersey FY22 Tick Research and Control-Special Purpose Funding to CVB/NJAES and by Cooperative Agreement Number 1U01CK000509 between the Centers for Disease Control and Prevention (CDC) and the Northeast Center of Excellence in Vector Borne Diseases (PI at Rutgers, D. M. F). The contents of this work are solely the responsibility of the authors and do not necessarily represent the official views of the Centers for Disease Control and Prevention or the Department of Health and Human Services.
Competing interests
None.
Ethical standards
Not applicable.