In the UK, mortality of live born piglets is approximately 11 %(1) with over 50 % of deaths occurring within 3 d of birth(Reference Dyke and Swierstra2, Reference Van der Lende, Knol and Leenhouwers3). Many factors influence piglet mortality(Reference Varley and Varley4), but one of the main causes of mortality is cold stress due to lack of energy reserves at birth(Reference Varley and Varley4–Reference Herpin, Damon and Le Dividich7).
There is a plethora of data demonstrating that fat supplementation of sow diets during late gestation increases liver glycogen and body fat reserves in their offspring at birth(Reference Boyd, Moser, Peo and Cunningham8–Reference Boyd, Moser, Lewis, Peo, Johnson and Nimmo10). Furthermore, it is well established that fat supplementation of sow diets during late gestation improves the growth performance and survival of their offspring(Reference Wladyslaw11–Reference Averette Gatlin, Odle, Soede and Hansen13); this improvement is most pronounced in low birth weight (LBW) piglets(Reference Seerley14–Reference Cox, Britt, Armstrong and Alhusen17). There is also some evidence to suggest that the fatty acid profile of the supplement is of importance; Jean & Chiang(Reference Jean and Chiang18), for example, observed that medium-chain TAG and coconut oil were more effective than soya oil at reducing piglet morbidity and mortality.
Substantial evidence now exists to support Barker's fetal origins hypothesis, which suggests that fetal undernutrition is associated with an altered fetal and placental endocrine environment, thereby linking fetal undernutrition with abnormal organ structure, function and disease in later life(Reference Barker19, Reference Eriksson20). Fetal nutrition is dependent upon the transfer of nutrients across the placenta. Manipulation of placental development by maternal nutrition, during early–mid-gestation, is therefore likely to have direct consequences for fetal nutrition and development. Our previous work(Reference Laws, Laws, Lean, Dodds and Clarke21) suggests that energy supplementation and the fatty acid profile of the maternal diet during the first half of gestation influence the growth and development of piglets both in utero and during postnatal life. Since LBW has previously been linked to placental insufficiency(Reference Ashworth and McArdle22) and placental development occurs during the first half of gestation(Reference Pomeroy23, Reference Knight, Bazer, Thatcher, Franke and Wallace24), it seems probable that the maternal diet during early gestation will also have some influence upon the incidence of LBW. It was the aim of the present study to determine the consequences of altering the fatty acid profile of a 10 % dietary supplement, offered either during the first half or second half of pregnancy on the incidence of LBW piglets and their subsequent growth and endocrine profiles.
Methods
Animals and diets
All animals used in these studies were maintained at the Pig Research and Development Unit, Imperial College London. Experimental procedures were carried out according to the regulations of the Animals (Scientific Procedures) Act, 1986 and were licensed by the Home Office (UK). At all stages of life, animals were kept within the guidelines set out by the Department for Environment, Food and Rural Affairs(25).
Initially birth weight data for the litters of a control population of fifty multiparous sows, of the same genetic line as those used in this experiment, were pooled and a probability plot of their weight constructed in MINITAB (Fig. 1). Piglets with a birth weight under the 10th percentile for the population were considered to be of LBW, while those between the 40th and 60th percentiles were considered to be of normal birth weight (NBW). In the control population, the LBW animals weighed less than 1·09 kg and NBW animals were between 1·46 kg and 1·64 kg. These weight boundaries were used to classify LBW and NBW piglets within the litters of experimental animals at birth.

Fig. 1 (a) Probability plot and (b) distribution curve of piglet birth weight in a control population (659 piglets) born to fifty multiparous sows of a commercial genotype (25 % Meishan; 12·5 % Duroc; 62·5 % Large White × Landrace). Mean 1·54 (sd 0·36) kg.
Eighty-eight sows of a commercial genotype (25 % Meishan; 12·5 % Duroc; 62·5 % Large White × Landrace) were entered into the study after weaning and prior to service. Sows were categorized by parity before being randomly assigned to one of eleven dietary treatment groups, to ensure that parity was balanced across treatments. All sows were artificially inseminated with pooled Large White semen (P17 2006; JSR Genetics, Southburn, Driffield, East Yorkshire, UK). The control diet consisted of the standard diet (3 kg/d) (ABN HE sow pellets; 13·1 MJ/kg metabolizable energy; 12·7 % protein; 4·5 % fat; ABN, Peterborough, Cambridgeshire, UK), and was fed throughout gestation. Experimental diets were as the control ration plus 10 % (3·93 MJ/d) extra energy derived from either: (i) excess pellets (E); (ii) palm oil (PO); (iii) olive oil (OO); (iv) sunflower oil (SO); (v) fish (salmon) oil (FO). Experimental diets were fed during either the first half (G1; from days 0 to 60 of gestation) or second half (G2; from day 60 of gestation until term at approximately day 115 of gestation). During the period when supplemented diets were not received, experimental sows were offered the standard diet (3 kg/d) as for the control group. Fatty acid profiles of the diets were determined by GLC and can be seen in Table 1. All diets, with the exception of the control ration, were isoenergetic. Between farrowing and weaning (21–28 d post partum) sows were offered a standard lactation ration (6–9 kg) (ABN supreme lactation pellets; 14·1 MJ/kg metabolizable energy; 18 % protein; 7·2 % oil; ABN). Piglets had ad libitum access to creep feed (Primary Select; 17·35 MJ/kg digestible energy (DE), 23·5 % protein; Primary Diets Ltd., Melmerby, Ripon, North Yorkshire, UK) from day 14 of life.
Table 1 The fatty acid composition of diets*
(Mean percentages of total lipid fraction)

ND, none detected.
* For details of diets and procedures, see Methods.
All piglets were weaned at 21–28 d, provided they weighed more than 6·5 kg. Piglets that failed to reach 6·5 kg by this age were fostered on to another sow for a further 1–2 weeks. Post-weaning management of all pigs was according to the standard procedures of the College Pig Unit. Maximum group sizes were thirty animals per pen (total area 5·45 m2; minimum area per pig 0·18 m2); animals were grouped according to sex and weight and food was available ad libitum throughout post weaning (Primary Benefit; 15·6 MJ/kg DE, 22·8 % protein; Primary Diets Ltd.), growing (Delatwean 10 salocin pellets; 15 MJ/kg DE; 20·9 % protein; ABN) and fattening (Deltagrow 25 salocin pellets; 14 MJ/kg DE; 17·8 % protein; ABN) periods.
Piglet growth and composition
Piglet growth performance was observed throughout the neonatal period; body weight, crown to rump length and body composition using a total body electrical conductivity analysing system (TOBEC, Model-SA3000 EMSCAN/TOBEC, SA-3203; EM-Scan Inc., Springfield, IL, USA) were recorded on days 0, 7, 14 and 21 of life. Piglet growth rate was calculated by regression analysis of piglet weight against time. Piglet fat free mass (FFM) per kg was calculated using the equation, shown later, as suggested in the TOBEC manufacturer's instructions.

At commercial end-point (80 (se 5) kg) back fat and eye muscle depth were measured, at the P2 position, using ultrasound (Aloka-echo camera 550-500; Aloka Ltd., Osaka, Japan).
Milk intake
Individual piglet milk intake was assessed using an adaptation of the weigh-suckle-weigh method, described by Sinclair et al. (Reference Sinclair, Shaw, Edwards, Hoste and McCartney26). Intake was assessed on days 3, 7, 14 and 21 of lactation. The first recording of milk intake was conducted on day 3 to ensure that we were not measuring colostral yield. Natural suckling was allowed, and the inter-suckling interval recorded. Piglets were observed throughout and weighed individually before and after four consecutive sucklings. Urination and defecation by piglets during this period was also recorded. The following equation was used to estimate milk intake per suckling:

where W, piglet weight gain (kg); U, weight loss due to urination; D, weight loss due to defecation; M, metabolic weight loss. Weight loss due to urination was calculated using the equation described by Klaver et al. (Reference Klaver, van Kempen, de Lange, Verstegen and Boer27):

where U, number of urinations; W 0 ·75, piglet metabolic weight.
A 10 g loss was allowed per defecation(Reference Sinclair, Shaw, Edwards, Hoste and McCartney26). An estimate of metabolic loss was calculated using the equation described by Noblet & Etienne(Reference Noblet and Etienne28):

>
Blood sampling
Blood samples were obtained from the piglets on days 3 and 21 of life. Blood was put into an EDTA tube and immediately placed on ice and after centrifugation at 1600 g the plasma was removed and stored at − 20°C until further analysis. Plasma concentrations of leptin (Biogenesis XL-85; Biogenesis Ltd., Poole, Dorset, UK), insulin (ICN 07-260102; ICN Pharmaceuticals, New York, USA), insulin-like growth factor (IGF)-1; DSL-2800; Diagnostic Systems Laboratories Inc., Webster, Tx, USA) and thyroid hormones (triiodothyronine (ICN 07B74102; ICN Pharmaceuticals) and thyroxine (ICN 06B256447; ICN Pharmaceuticals) were determined by RIA using commercially available kits. Glucose (GL 366; Randox Laboratories Ltd., Crumlin, Antrim, UK), TAG (TR 148; Randox Laboratories Ltd.) and NEFA (944-75 409; Wako Chemicals GmbH, Neuss, Germany) were measured enzymatically. A reference sample was included in every assay and the intra- and inter-assay CV were below 5 and 10 % respectively.
Statistical analysis
One of the control sows became unwell shortly after farrowing and her piglets had to be weaned early. All data from this animal and her offspring were removed prior to analysis. Data were analysed with general linear model ANOVA using MINITAB version 13.1 computer software (Minitab Ltd., Coventry, UK). The main effect included in the model was dietary treatment; parity was included as a covariate. Data were adjusted for litter size or number of piglets reared as appropriate. When treatment effects were significantly different, pair-wise comparisons of means were conducted using Tukey's test. Probability values of less than 0·05 were considered to be statistically significant.
Individual piglet growth rate was calculated by regression analysis of piglet weight against time. Analysis was conducted for two separate periods: (i) birth to weaning; (ii) over the lifetime of the pig up to commercial end-point. The slope of the line gave a measure of their growth rate (kg/d).
Results
After adjustment for both parity and litter size, the type and timing of maternal supplementation influenced the incidence of LBW piglets (P < 0·05; Table 2). The percentage of piglets born alive that could be categorized as LBW was highest in the litters of SOG1 sows, and lowest in those of OOG1 animals (P < 0·05; Table 2). The incidence of NBW piglets was similar irrespective of sow parity, litter size and type or timing of maternal supplementation (Table 2).
Table 2 Effect of timing and type of maternal supplement on the incidence of low birth weight (LBW; <1·09 kg) and normal birth weight (NBW; 1·46–1·64 kg) piglets†
(Least squares means with their standard errors)

E, excess; PO, palm oil; OO, olive oil; SO, sunflower oil; FO, fish oil; G1, first half of gestation; G2, second half of gestation.
* Mean values were significantly different (P < 0·05).
† For details of diets and procedures, see Methods.
‡ Data for number of piglets born alive were analysed in Minitab using ANOVA general linear model (parity (P < 0·05) and litter size (P < 0·001) were analysed as covariates).
Comparison of the distribution curve for piglet birth weights, within each treatment, gives some insight into the effect of the different dietary supplements (Fig. 2). The OOG1 diet resulted in a shift of the distribution curve to the right (i.e. an increase in the average piglet birth weight, while deviation from the mean was similar to that observed in other groups), meaning that fewer than 10 % of the OOG1 piglets fell below the 10th percentile weight (1·09 kg) derived from the control population. In contrast, the SOG1 diet resulted in a shift of the distribution curve to the left and a slight skewing towards the left (i.e. a slight decrease in average birth weight, with a tendency for an increased proportion of LBW piglets), meaning that more than 10 % of piglets fell below the 10th percentile weight (1·09 kg) for the control population. Interestingly, the POG1 diet appeared to result in a shift of the distribution curve to the left and to result in a wider shallower curve (i.e. a slight decrease in average birth weight and a greater proportion of piglets at either end of the weight range); these differences appear to be largely attributable to the increased litter size observed on this treatment.
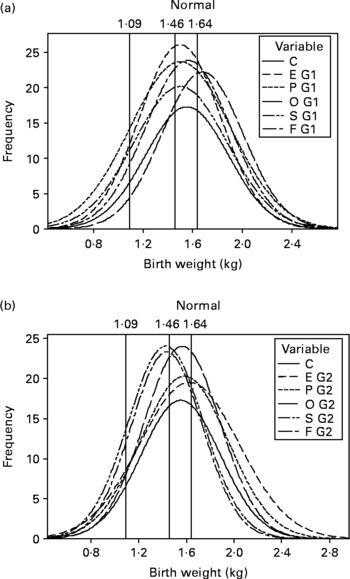
Fig. 2 Effect of type of maternal energy supplement on the distribution of piglet birth weights when supplementation occurs during (a) the first 60 d of gestation (G1) or (b) from 60 d of gestation until term (approximately 115 d) (G2). C, control; E, excess; P, palm oil; O, olive oil; S, sunflower oil; F, fish oil. For details of diets and procedures, see Methods.
G2 supplementation with OO or SO diets resulted in a shift of the distribution curve for piglet birth weight to the left (i.e. a decrease in the average birth weight). In contrast supplementation with the E diet during G2 resulted in a shift of the peak to the right as a result of a skewing towards heavier piglets (i.e. an increase in the average birth weight of piglets, with a more gradual decline in frequency at the heavier end of the range).
By 21 d of age, after correction for maternal parity and litter size, the surviving LBW offspring of FOG1 and FOG2 sows were still lighter than their NBW siblings (P < 0·05; Table 3). A similar pattern was observed in the weights of the offspring of SOG2 sows (P < 0·05; Table 3) on day 21 of life. At 21 d of age the offspring of SOG2 sows were lighter than those of FOG1 or EG2 animals (P < 0·001 and P < 0·05 respectively; Table 3).
Table 3 Effect of timing and type of maternal supplement and piglet birth weight category on weight change during the first 21 d of life *†
(Least squares means with their standard errors)

LBW, low birth weight; NBW, normal birth weight; G1, first half of gestation; G2, second half of gestation; Con, control; E, excess; PO, palm oil; OO, olive oil; SO, sunflower oil; FO, fish oil.
abc Mean values within a row with unlike superscript letters were significantly different (P < 0·05).
AB Mean values within a column with unlike superscript letters were significantly different (P < 0·05).
* Data were analysed in Minitab using ANOVA general linear model (parity (P < 0·001), sex and litter size (P < 0·001) were analysed as covariates).
† For details of diets and procedures, see Methods.
After correction for maternal parity and litter size, growth rate in terms of weight gain per d was similar for all LBW piglets, irrespective of type and timing of maternal supplementation. When the growth rates of low and NBW piglets are compared within treatment groups, less of a difference was apparent when maternal supplementation occurred during G1 (Table 3). This was particularly noticeable when the timing of maternal supplementation with the E, PO or SO diets were compared; the difference between the growth rates of LBW and NBW piglets reached significance only when supplementation occurred during G2 (P < 0·05; Table 3).
After correction for maternal parity and litter size, the LBW offspring of control sows had a greater FFM/kg at birth when compared with either the LBW offspring of supplemented animals or to their NBW siblings (P < 0·01; Table 4). Interestingly, the LBW offspring of control sows exhibited a more rapid decline in FFM/kg over the pre-weaning period than those of supplemented sows (P < 0·05) and of their NBW siblings (P < 0·001; Table 4).
Table 4 Effect of timing and type of maternal supplement and piglet birth weight category on change in fat free mass (FFM) per kg body weight, during the first 21 d of life*Ȃ
(Least squares means with their standard errors)

G1, first half of gestation; G2, second half of gestation; LBW, low birth weight; NBW, normal birth weight; Con, control; E, excess; PO, palm oil; OO, olive oil; SO, sunflower oil; FO, fish oil.
ab Mean values within a row with unlike superscript letters were significantly different (P < 0·05).
ABCD Mean values within a column with unlike superscript letters were significantly different (P < 0·05).
* Data were analysed in Minitab using ANOVA general linear model (parity, sex and litter size (P < 0·001) were analysed as covariates).
† For details of diets and procedures, see Methods.
The average daily milk intake of LBW piglets was lower throughout the pre-weaning period than that for their NBW siblings (Table 5). Differences between birth weight groups did not reach significance, however, due to the wide variation in milk intake between LBW piglets.
Table 5 Effect of timing and type of maternal supplement and piglet birth weight category on milk intake*†
(Least squares means with their standard errors)

LBW, low birth weight; NBW, normal birth weight; G1, first half of gestation; G2, second half of gestation; Con, control; E, excess; PO, palm oil; OO, olive oil; SO, sunflower oil; FO, fish oil.
* Calculated from average individual piglet milk consumption (over four nursings) multiplied by number of suckling per d. Data were analysed in Minitab using ANOVA general linear model (parity (P < 0·01), sex and litter size (P < 0·001) were analysed as covariates).
† For details of diets and procedures, see Methods.
At commercial end-point (determined by weight), there were no significant differences in pig age, weight or body composition irrespective of birth weight category or maternal diet. Similarly, there was no difference in pig growth rate from birth to slaughter (Table 6).
Table 6 Effect of timing and type pf maternal supplement and piglet birth weight category on performance at commercial end point*†
(Least squares means with their standard errors)

LBW, low birth weight; NBW, normal birth weight; G1, first half of gestation; G2, second half of gestation; Con, control; E, excess; PO, palm oil; OO, olive oil; SO, sunflower oil; FO, fish oil.
* Data were analysed in Minitab using ANOVA general linear model (parity, sex and litter size were analysed as covariates).
† For details of diets and procedures, see Methods.
There was a trend ((P < 0·1) for plasma glucose concentration to be lower on day 3 of life in LBW piglets (6·1 (SE 0·55) mm) born to EG1 sows compared with their NBW counterparts (7·69 (SE 0·56) mm) but there were no other noticeable differences observed between either birth weight or dietary treatment groups at days 3 and 21 of age. Plasma glucose concentrations tended to be higher on day 3 in those offspring in the G2 group (P < 0·05) compared with G1 piglets. In G2, LBW piglets (6·12 (SE 0·66) mm) born to SO diet sows had lower plasma glucose (P < 0·05) than their NBW siblings (8·12 (SE 0·52)) and LBW animals in the other groups on both days 3 and 21 of postnatal life.
On day 3 of age, plasma TAG and NEFA were higher (P < 0·001) in low (TAG 2·96 (SE 0·32) mm; NEFA 3·04 (SE 0·19) mm) compared with normal (TAG 1·69 (SE 0·21) mm; NEFA 0·90 (SE 0·11) mm) birth weight piglets born to PO-supplemented sows in G1 and all other treatment groups. These differences were no longer apparent by day 21 as concentrations had decreased (P < 0·001) over the neonatal period. Plasma TAG and NEFA were generally higher in LBW offspring born in G1 compared with G2 (P < 0·01) on day 3 but this observation was less marked with regard to the NBW group.
NBW (18·15 (SE 2·05) μIU/ml) piglets born to EO sows exhibited elevated (P < 0·001) concentrations of plasma insulin compared with their LBW siblings (8·42 (SE 2·29) μIU/ml) and all other treatment groups; these differences were not apparent by day 21 of age. LBW FO animals (1·25 (SE 0·22) μIU/ml) had significantly higher (P < 0·05) circulating insulin compared with NBW piglets (0·87 (SE 0·14) μIU/ml) and those born to other fat-supplemented mothers in G2 (P < 0·001) but by day 21 plasma insulin was similar between groups. There was no effect of type or timing of maternal dietary supplementation in gestation or birth weight group on plasma leptin, IGF-1, thyroxine or triiodothyronine at any sampling point.
Discussion
It is well established that supplementation of the maternal diet during late gestation improves the growth performance and survival of LBW piglets(Reference Seerley14–Reference Cox, Britt, Armstrong and Alhusen17). This is particularly true with the addition of medium-chain TAG to the sow diet(Reference Jean and Chiang18, Reference Azain29). Furthermore Bee(Reference Bee30) found that maternal energy consumption during early gestation determined the ratio of primary to secondary muscle fibres, while birth weight affected muscle fibre area. Both the ratio of primary to secondary muscle fibres and muscle fibre area are known to have consequences for growth performance and carcass composition(Reference Powell and Aberle31–Reference Dwyer, Stickland and Fletcher33). In the present study, both the timing and type of energy supplementation were found to be of importance, with regard to the incidence of LBW piglets, although the body composition of LBW piglets appeared to be improved irrespective of the type or timing of energy supplementation.
The greatest incidence of LBW piglets was observed in the litters of SOG1 sows, in contrast with those of OOG1 animals, which appeared to have a reduced incidence of LBW piglets. The differences observed between these two supplements are likely to have been due to the fatty acid profile of the diet, since parity, total litter size, number of piglets born alive and gestation length were similar for both groups(Reference Laws, Amusquivar, Laws, Herrera, Lean, Dodds and Clarke34). A possible explanation for the differences observed is that gestational gain in back fat thickness at the P2 position was greater in the SOG1 animals, than in OOG1 sows(Reference Laws, Amusquivar, Laws, Herrera, Lean, Dodds and Clarke34). This suggests that a greater proportion of the supplement was partitioned towards maternal reserves rather than to the growth of the products of conception in SOG1 sows. During the first half of gestation, energy directed towards growth of the products of conception could be expected to improve placental development(Reference Knight, Bazer, Thatcher, Franke and Wallace24) and may provide an explanation for the decreased occurrence of LBW piglets in the litters of OOG1 animals. It is known that fetal growth is directly dependent on the supply of nutrients across the placenta and will therefore be influenced by placental development(Reference Hammond35, Reference Hammond36) and efficiency(Reference Ashworth, Finch, Page, Nwagwu and McArdle37). Analysis of distribution curves of piglet birth weights for the different treatment groups revealed that the mean birth weight of OOG1 piglets is increased. Standard deviation from the mean was similar for OOG1 to that calculated for other supplements, suggesting that more nutrients were available in utero to the offspring of OOG1 sows. It is speculated that improved nutrient availability may be as a result of improved placental development during G1.
It is well established that the growth of small piglets during the pre-weaning period may be improved as a consequence of fat supplementation of their dams(Reference Cox, Britt, Armstrong and Alhusen17), suggesting that they can compete more successfully with their litter mates. In the present series of experiments, the growth rate of LBW piglets was similar, irrespective of type or timing of maternal energy supplementation. This is surprising since the average growth rate, from whole litter data, was influenced by the type of maternal energy supplement during G1(Reference Laws, Laws, Lean, Dodds and Clarke21, Reference Laws, Laws, Lean, Dodds and Clarke38). It is speculated that the low numbers of LBW piglets, compared with those available from the whole litter, together with other factors affecting piglet growth (litter size, thermal stress, etc.) may have masked the effects of maternal supplementation.
The growth of LBW piglets is known to be slower than that of heavier piglets from the same litter(Reference Powell and Aberle39–Reference Gadd41). In the current experiments comparison of growth rates of LBW and NBW piglets within treatment groups revealed less of a difference in growth rate in the offspring of sows supplemented with extra energy during G1; this is interesting because placental development occurs during the first 60 d of gestation(Reference Knight, Bazer, Thatcher, Franke and Wallace24). It is probable that the reduced growth performance of LBW piglets, born to sows supplemented during G2, may be primarily due to compromised fetal development as a result of placental insufficiency. Other studies have also linked reduced fetal growth to placental insufficiency(Reference Ashworth and McArdle22, Reference Ashworth, Finch, Page, Nwagwu and McArdle37, Reference Warshaw42). The increased weight at 21 d of age of the NBW offspring of FOG1 sows agrees with the results for average litter performance(Reference Laws, Laws, Lean, Dodds and Clarke21, Reference Laws, Laws, Lean, Dodds and Clarke38) and is in agreement with the findings of others(Reference Rooke, Sinclair, Edwards, Cordoba, Pkiyach, Penny, Penny, Finch and Horgan12, Reference Rooke, Shanks and Edwards43, Reference Rooke, Sinclair and Edwards44). It has previously been suggested that the improved performance of piglets born to oil-supplemented dams is as a result of improved piglet vigour at birth(Reference Rooke, Sinclair, Edwards, Cordoba, Pkiyach, Penny, Penny, Finch and Horgan12, Reference Cordoba, Pkiyach, Rooke, Edwards, Penny and Pike45); it is speculated that improved vigour at birth may also be responsible for the improved growth performance of NBW FOG1 piglets in the current study. The increased weight at 21 d of age of NBW offspring, of EG2 sows, is in agreement with the results for whole litter performance(Reference Laws, Laws, Lean, Dodds and Clarke38) and suggests that the balance of energy to protein in maternal diets is important during the second half of gestation if piglet performance is to be maximized.
Body composition of piglets at birth has been the subject of much previous research, it is well known that body fat and glycogen reserves are increased with the addition of fat to maternal diets(Reference Boyd, Moser, Peo and Cunningham9, Reference Boyd, Moser, Lewis, Peo, Johnson and Nimmo10, Reference Seerley14, Reference Seerley, Pace, Foley and Scarth46, Reference Le Dividich, Esnault, Lynch, Hoo-Paris, Castex and Peiniau47). In the current study, it was observed that the LBW piglets of control sows had reduced body fat (as indicated by their higher FFM per kg), compared with their NBW siblings and that the addition of extra energy to the maternal diet resulted in increased body fat of LBW piglets, irrespective of the type or timing of supplementation. The present results may provide an explanation for the increased survival of LBW piglets reported in previous studies with maternal supplementation(Reference Boyd, Moser, Peo and Cunningham8, Reference Cast, Moser, Peo and Cunningham15, Reference Cieslak, Leibbrandt and Benevenga16, Reference Seerley, Pace, Foley and Scarth46), since nutritional status and energy balance during the immediate neonatal period is known to be of importance with regard to survival(Reference Le Dividich, Esnault, Lynch, Hoo-Paris, Castex and Peiniau47, Reference Leenhouwers, Knol, De Groot, Vos and Van de Lende48).
The LBW offspring of control sows had an increased rate of fat deposition during the pre-weaning period, compared with those of supplemented sows and their NBW siblings. By 21 d of age, surviving LBW offspring of control sows had a similar quantity of FFM per kg body weight to that exhibited by low and NBW piglets in other groups; their body weight was also similar to that for LBW piglets from other treatment groups. Rapid fat deposition also suggests that growth was less efficient than in either NBW siblings or LBW offspring from other groups(Reference Dwyer, Stickland and Fletcher33, Reference Powell and Aberle39). Since the weight gain of all LBW piglets was similar during the first 21 d of life, but the fat deposition of those born to control sows was greater, their energy intake would be expected to be greater over this period. In the current study, milk energy yield per kg was similar throughout lactation irrespective of type or timing of maternal energy supplementation(Reference Laws, Amusquivar, Laws, Herrera, Lean, Dodds and Clarke34). Similarly, the milk consumption of LBW piglets was similar irrespective of type or timing of energy supplementation, although there was more variation in milk consumption of control animals than those in other groups. An alternative explanation for the rapid fat deposition observed in LBW offspring of control sows is that they may have consumed more creep feed than those from other groups, although this seems unlikely since the consumption of creep feed during the first 3 weeks of life is usually very low(Reference Pluske, Williams, Aherne and Varley49).
Previously, LBW piglets have been observed to reach a lower mature body weight, when compared with heavier litter mates(Reference Ritacco, Radecki and Schoknecht40). Consequently, there is an inverse relationship between birth weight and time taken to reach commercial slaughter weight(Reference Gadd41). Similarly, average daily gain has been observed to be lower in LBW pigs prior to weaning and from weaning to 70 d of age(Reference Dwyer, Fletcher and Stickland32). In the present study, there was no significant difference in age of pigs at slaughter, irrespective of timing or type of maternal supplementation or piglet birth weight. Although not significant, age at slaughter was generally slightly higher for LBW animals, but the reverse was true in the case of those animals whose dams had received EG2, FOG2 or POG1 diets; it is not clear whether these results demonstrate improved performance of some LBW piglets as a result of catch-up growth(Reference Widdowson and McCance50).
Previously it has been found that LBW piglets have lower feed conversion efficiency, due to their lower potential for lean deposition, compared with NBW pigs(Reference Dwyer, Fletcher and Stickland32, Reference Powell and Aberle39). In the current studies, no difference was observed in the back fat depth or eye muscle thickness at the P2 position at slaughter. This is in agreement with the findings of England(Reference England51) and Hegarty & Allen(Reference Hegarty and Allen52), who found no difference in the carcass composition of low and NBW animals at similar slaughter weights. Recently, it has been suggested that the feed conversion efficiency of small pigs (0·9–1·3 kg) is similar to that observed for larger pigs, but that very small pigs (800–900 g) have a 30 % lower feed conversion efficiency(Reference Gadd41); this has previously been hinted at by Powell & Aberle(Reference Powell and Aberle39). In the present study, average birth weight of LBW piglets was above 0·9 kg, irrespective of maternal diet, and may provide an explanation for the lack of difference observed between birth weight groups.
In addition to those already discussed, there are a number of other factors that were not measured that influence pig growth. It is well established that social stressors influence pig performance(53–Reference Schmolke, Li and Gonyou55). On the College Unit, piglets are grouped by sex and weight at weaning into pens of thirty animals. By dividing pigs into groups in this way, it becomes possible for a LBW pig to become more dominant than would be possible if whole litters were transferred together. Similarly, a dominant animal, which gets grouped with the largest pigs weaned, may end up in a subordinate position. It is not well documented as to how such social situations would affect the feed consumption and efficiency of thermoregulation and growth in pigs from different birth weight groups.
A previous study by Boyd et al. (Reference Boyd, Moser, Lewis, Peo, Johnson and Nimmo10) demonstrated that the addition of fat to the maternal diet did not influence piglet plasma glucose at birth. However, other studies have reported that the addition of medium-chain TAG or saturated fat to the sows' diet during late gestation cause an increase in glucose concentration of up to 16 %(Reference Seerley14, Reference Jean and Chiang18). The present data support these findings and are further modified by the timing of maternal supplementation as plasma glucose was higher in G1 compared with G2 offspring. Moreover, this mirrors the differences in maternal glucose towards the end of pregnancy. It is proposed that exposure to high concentrations of glucose in utero (as a cause of glucose-sparing) alters the hypothalamic set-point of the offspring and thus they strive to maintain this elevated set-point after birth. By day 21 the differences in plasma glucose are no longer apparent, suggesting that the glucose set-point may be readjusted as the offspring adapts to postnatal life.
Several studies have reported that fat in the late gestation diet of sows has no effect on piglet plasma NEFA(Reference Boyd, Moser, Lewis, Peo, Johnson and Nimmo10, Reference Rooke, Bland and Edwards56, Reference Newcomb, Harmon, Nelssen, Thulin and Allee57) and TAG(Reference Rooke, Bland and Edwards56) concentration. With the exception of the POG1 offspring, a similar scenario was demonstrated in the current investigation. The reasons why pigs born to POG1 sows exhibited elevated concentrations of NEFA and TAG remains to be fully established.
NBW piglets born to either G1 or G2 sows exhibited higher concentrations than their LBW counterparts and all offspring born to fat-supplemented mothers. The higher concentrations of insulin on day 3 of age may also provide one explanation for the lower plasma glucose observed in these pigs. There is great variation in the insulin results obtained in the current study, which may be due to the inability to starve the pigs prior to blood sampling as they had access to feed ad libitum. Insulin concentrations are usually low before a feed and much higher after an intake of food. Since blood samples were not necessarily taken from the pigs at the same time interval after feeding, there will be a high degree of variation in the results. It is interesting to note that there was no difference in maternal plasma glucose between EG1 and EG2, which may in part explain why insulin concentrations are similar in these groups.
It is well documented that IGF-1 is an important regulator of postnatal growth and development and so, as expected, overall plasma IGF-1 concentrations were shown to increase from day 3 to day 21. Averette et al. (Reference Averette, Odle, Monaco and Donovan58) has previously shown that IGF-1 is higher in the colostrums of sows fed fat and these authors proposed that this may be one explanation for the improved neonatal growth rate of their piglets. There is no evidence to support this hypothesis in the present study as differences in IGF-1 were not apparent, despite differences in growth between LBW and NBW piglets.
Thyroid hormones are known to influence both the physical and behavioural development of the newborn animal. Low plasma concentrations of thyroxine and triiodothyronine have been linked to LBW and poor growth performance in sheep(Reference Clarke, Darby, Lomax and Symonds59) but a similar situation was not observed in the present study.
Conclusions
Maternal dietary supplementation altered the distribution of piglet birth weights and improved the energy status of LBW piglets, irrespective of the fatty acid profile or timing of the supplement. Supplementation with MUFA during G1 appeared to have beneficial effects as it reduced the incidence of LBW, whereas offering a diet high in PUFA had the reverse effect.
Acknowledgements
The authors wish to acknowledge the financial support of the Commission of the European Communities (QLK1-2001-00 138). perilip; this grant was awarded to P. F. D., L. C. and I. J. L. This work does not necessarily reflect the views of the Commission and in no way anticipates its future policy in this area. The authors thank United Fish Industries for providing the salmon oil and the staff at The Pig Research and Development Unit for their care of animals throughout the study. The authors would also like to thank Emilio Herrera and Encarnacion Amusquivar (Universidad San Pablo-CEU, Madrid, Spain) for analysing the fatty acid profile of the experimental diets. The technical help of Kate Perkins and Kirsty Massey was also greatly appreciated. The investigation team are former members of the Animal Science Research Section of Imperial College London (Wye Campus). There are no conflicts of interest.