The circle of Willis (CW), an arterial anastomotic polygon located at the skull base, is of key importance regarding the maintenance of cerebral perfusion. Variations of the CW are common findings in healthy individuals and are associated with a greater risk of stroke in case of hypoplastic or absent segments of the CW. Prospective studies have confirmed that incomplete anterior CW is significantly associated with anterior circulation stroke in patients without prior cerebrovascular disease (van Seeters et al., Reference van Seeters, Hendrikse, Biessels, Velthuis, Mali, Kappelle and Group2015), and the risk is even higher if incomplete posterior CW is present simultaneously (van Seeters et al., Reference van Seeters, Hendrikse, Biessels, Velthuis, Mali, Kappelle and Group2015). Non-functional anterior and posterior collaterals have a higher prevalence in patients with anterior circulation stroke compared to patients with asymptomatic carotid artery disease (Hoksbergen, Legemate et al., Reference Hoksbergen, Legemate, Csiba, Csati, Siro and Fulesdi2003). The collateral flow in the CW has been widely investigated in patients with internal carotid artery (ICA) occlusion or stenosis, and intact collaterals seem to have a key role in maintaining cerebral perfusion in this patient group (Bisschops et al., Reference Bisschops, Klijn, Kappelle, van Huffelen and van der Grond2003; Henderson et al., Reference Henderson, Eliasziw, Fox, Rothwell and Barnett2000; Hendrikse et al., Reference Hendrikse, Hartkamp, Hillen, Mali and van der Grond2001; Kluytmans et al., Reference Kluytmans, van der Grond, van Everdingen, Klijn, Kappelle and Viergever1999; Qiu et al., Reference Qiu, Zhang, Xue, Jiang and Zhang2015; Rutgers et al., Reference Rutgers, Klijn, Kappelle and van der Grond2004; Schomer et al., Reference Schomer, Marks, Steinberg, Johnstone, Boothroyd, Ross and Enzmann1994) as the collaterals may compensate for the restricted flow in the stenotic vessel, which is the major artery ensuring cerebral blood supply (Morgenstern et al., Reference Morgenstern, Fox, Sharpe, Eliasziw, Barnett and Grotta1997; Tegos et al., Reference Tegos, Kalodiki, Daskalopoulou and Nicolaides2000). Other neurological conditions have been investigated in relation to CW variants as well, emphasizing the significance of CW variants. Aneurysms and their recurrence after endovascular coiling are more common with variant CW (Songsaeng et al., Reference Songsaeng, Geibprasert, Willinsky, Tymianski, TerBrugge and Krings2010). There might be an association between the variant CW anatomy and white matter disease (Manolio et al., Reference Manolio, Burke, O'Leary, Evans, Beauchamp, Knepper and Ward1999; Ryan et al., Reference Ryan, Byrne, Dunne, Harmon and Harbison2015; Saba et al., Reference Saba, Raz, Fatterpekar, Montisci, di Martino, Bassareo and Piga2015; Saba et al., Reference Saba, Sanfilippo, Porcu, Lucatelli, Montisci, Zaccagna and Wintermark2017), migraine with aura, and mesial temporal sclerosis (Cucchiara et al., Reference Cucchiara, Wolf, Nagae, Zhang, Kasner, Datta and Detre2013; Kuyumcu et al., Reference Kuyumcu, Byrne, Dawe and Kocak2017). Interestingly, the causative role of cerebrovascular variants in hypertension was assumed, warranting further research in this field (Warnert et al., Reference Warnert, Rodrigues, Burchell, Neumann, Ratcliffe, Manghat and Hart2016).
Although the importance of vascular variants has been discussed and widely researched, their developmental background remains unclear. Variations of the posterior communicating artery (PCoA) are heritable in contrast to other CW variants according to the results of a recent family study conducted on families with intracranial aneurysms (Sanchez van Kammen et al., Reference Sanchez van Kammen, Moomaw, van der Schaaf, Brown, Woo, Broderick and Ruigrok2018). Other results suggested the genetic background of CW variants (Du et al., Reference Du, Zhu, Dong, Lu, Wang, Zeng and Chen2011; Faber et al., Reference Faber, Zhang, Rzechorzek, Dai, Summers, Blazek and Hedges2018; Li et al., Reference Li, Huo, Zhang, Lu, Li, Guo and Chen2015). A number of other stochastic and environmental factors, such as maternal pre-eclampsia, might alter the development of cerebrovascular variants (Luna et al., Reference Luna, Kay, Ratsep, Khalaj, Bidarimath, Peterson and Croy2016), and pre-maturity might affect the formation of CW variants (Malamateniou et al., Reference Malamateniou, Adams, Srinivasan, Allsop, Counsell, Cowan and Rutherford2009). Thus, assessing the genetic versus environmental origin of CW variants could also provide knowledge of genetic pre-dispositions for offspring and could help to understand the role of modifiable environmental factors. Accordingly, the aim of this twin study was to assess whether heritability plays a significant role in the formation of the variants of CW or they are influenced mostly by other (environmental or stochastic) factors.
Methods
Our study population consisted of healthy Caucasian twins who are members of the Hungarian Twin Registry (Littvay et al., Reference Littvay, Metneki, Tarnoki and Tarnoki2013). The investigation was approved by the regional ethical committees (Regional/Local Committee of Science and Research Ethics Borsod-Abaúj-Zemplén, Heves and Nógrád County, approval number: 1046-260/2014, 2014/09/22 as well as Semmelweis University Regional and Institutional Committee of Science and Research Ethics, approval number: 189/2014, 2014/10/21). All participating twins signed an informed consent. The tenets of the Declaration of Helsinki were followed. A multiple self-reported questionnaire, based on a seven-part, self-reported response, was used to maximize the accuracy of zygosity classification (Heath et al., Reference Heath, Nyholt, Neuman, Madden, Bucholz, Todd and Martin2003). Detailed history and risk factors were obtained by a questionnaire, collecting data on body weight and height, body mass index (BMI), smoking, hypertension, and hypercholesterolemia. Both former and current smokers were included in the smoking group.
The twins underwent 3D Time-of-Flight magnetic resonance angiography (TOF MRA) of the cerebral arteries if the magnetic resonance imaging (MRI) study was not contraindicated. Patients were excluded with any of the following conditions: pregnancy or positive pregnancy test, breast-feeding, immunosuppressive or immunomodulant therapy including systemic steroids in the past 30 days, chemotherapy within 1 year, major surgery within 2 months, and transfusion or blood products received within 2 months. Moreover, pacemaker, implantable cardioverter-defibrillator or other implanted devices, magnetic metal foreign bodies, claustrophobia, and aphasia were regarded as exclusion criteria. The studies were performed in part at the Borsod County University Teaching Hospital, Miskolc, Hungary, and partly at the Semmelweis University Magnetic Resonance Research Centre. Members of a twin pair were examined on the same day by a professional operator.
T1-weighted coronal, T2-weighted sagittal, axial trace-weighted diffusion, axial apparent diffusion coefficient, axial proton density, and axial T2 dark fluid images of the brain were obtained. TOF MR angiograms were obtained on the cranial vessels. No contrast agent was applied. The 3D multi-slab TOF MR angiograms were obtained and reconstructed with maximal intensity projection (MIP). At the first study location in the Borsod County University Teaching Hospital, measurements were performed using a Siemens Magnetom Verio 3 Tesla workstation (Siemens Healthcare GmbH, Erlangen, Germany). The following imaging parameters were used: RT/ET 21/3.43 ms, flip angle 18°, 768 × 768 matrix, 0.5 mm slice thickness. At the second study location, a Philips Ingenia 1.5 Tesla workstation (Philips Healthcare, Best, the Netherlands) was used with the following imaging parameters: RT/ET 23/3.45 ms, flip angle 18°, 552 × 332 matrix, 1.2 mm slice thickness. The twins also underwent transcranial Doppler sonography (TCD) study, which is an ultrasound-based method to assess real-time flow parameters in intracranial vessels. TCD was performed by the same investigator with the same ultrasound system (Multi-Dop T, Compumedics Germany GmbH, Singen, Germany) with a 2 MHz transducer. Through the temporal and transforaminal windows, mean flow velocity (MFV) and pulsatility index (PI) were recorded bilaterally from the M1 segment of the middle cerebral artery (MCA), the A1 segment of the anterior cerebral artery (ACA), P1, P2a, and P2b segments of the posterior cerebral artery (PCA), and basilar artery. MFV is calculated as the end-diastolic velocity (EDV) plus one-third of the difference between peak systolic velocity (PSV) and EDV (Bathala et al., Reference Bathala, Mehndiratta and Sharma2013). It is a useful marker of stenosis or occlusion of intracranial vessels. PI is used to assess cerebrovascular resistance and is calculated by subtracting EDV from PSV and dividing the value by MFV (Bathala et al., Reference Bathala, Mehndiratta and Sharma2013). Flow direction was determined on all the aforementioned vascular segments, which was normal in all individuals.
Evaluation of the CW was made both on MIP and multi-slice MR images. A single observer performed all measurements in the project. This observer was blinded to zygosity and all clinical information. We investigated CW variants from two points of view (Hartkamp et al., Reference Hartkamp, van Der Grond, van Everdingen, Hillen and Mali1999; Hoksbergen, Majoie et al., Reference Hoksbergen, Majoie, Hulsmans and Legemate2003; Li et al., Reference Li, Li, Lv, Li, Luo and Xie2011). First, we analyzed each vessel of the CW and determined whether they were non-hypoplastic, hypoplastic, or absent. Vessel diameters were measured on transverse slices of the 3D multi-slab TOF MR angiograms. We used a forced-choice method to define hypoplasia and absence of the vessels. Non-visible vessels were defined as absent (Hoksbergen, Majoie et al., Reference Hoksbergen, Majoie, Hulsmans and Legemate2003). Visible arteries with a diameter under 0.8 mm were defined as hypoplastic (Hartkamp et al., Reference Hartkamp, van Der Grond, van Everdingen, Hillen and Mali1999). Second, we categorized the anterior and posterior CW variants separately according to their appearance. We used the morphological classification described by Hartkamp et al. (Reference Hartkamp, van Der Grond, van Everdingen, Hillen and Mali1999) and Li et al. (Reference Li, Li, Lv, Li, Luo and Xie2011) as a basis. However, we made some modifications in their categories because some were not applicable to our findings. Figures 1 and 2 show our classification of the anterior and posterior CW variants.
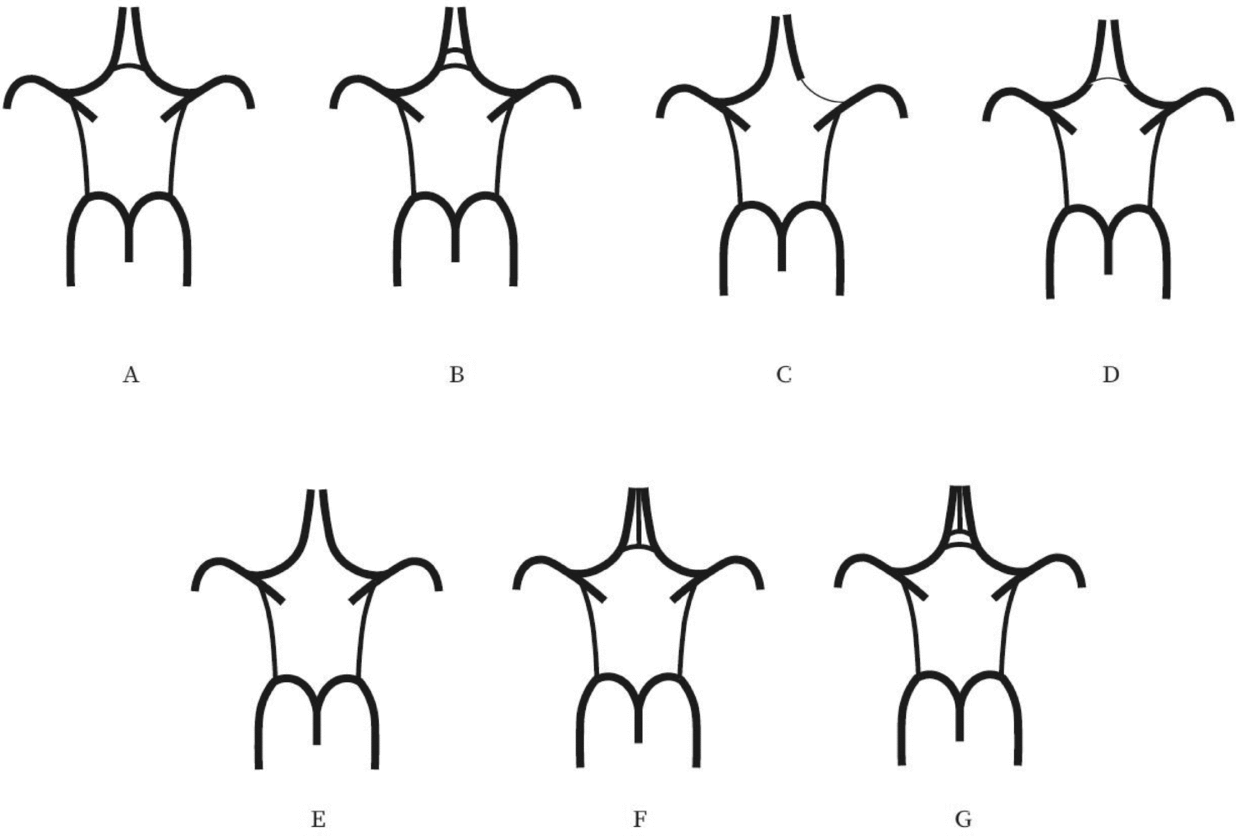
FIGURE 1 Variations of the anterior part of the circle of Willis. (A) “Normal” variant with single, non-hypoplastic ACoA and ACAs. (B) Two ACoAs. (C) Unilateral ACA A1 segment hypoplasia. (D) Hypoplastic ACoA. (E) Absent ACoA. (F) Medial artery of the corpus callosum arising from the ACoA. (G) Two ACoAs with medial artery of the corpus callosum arising from the ACoA. ACA = anterior cerebral artery, ACoA = anterior communicating artery.
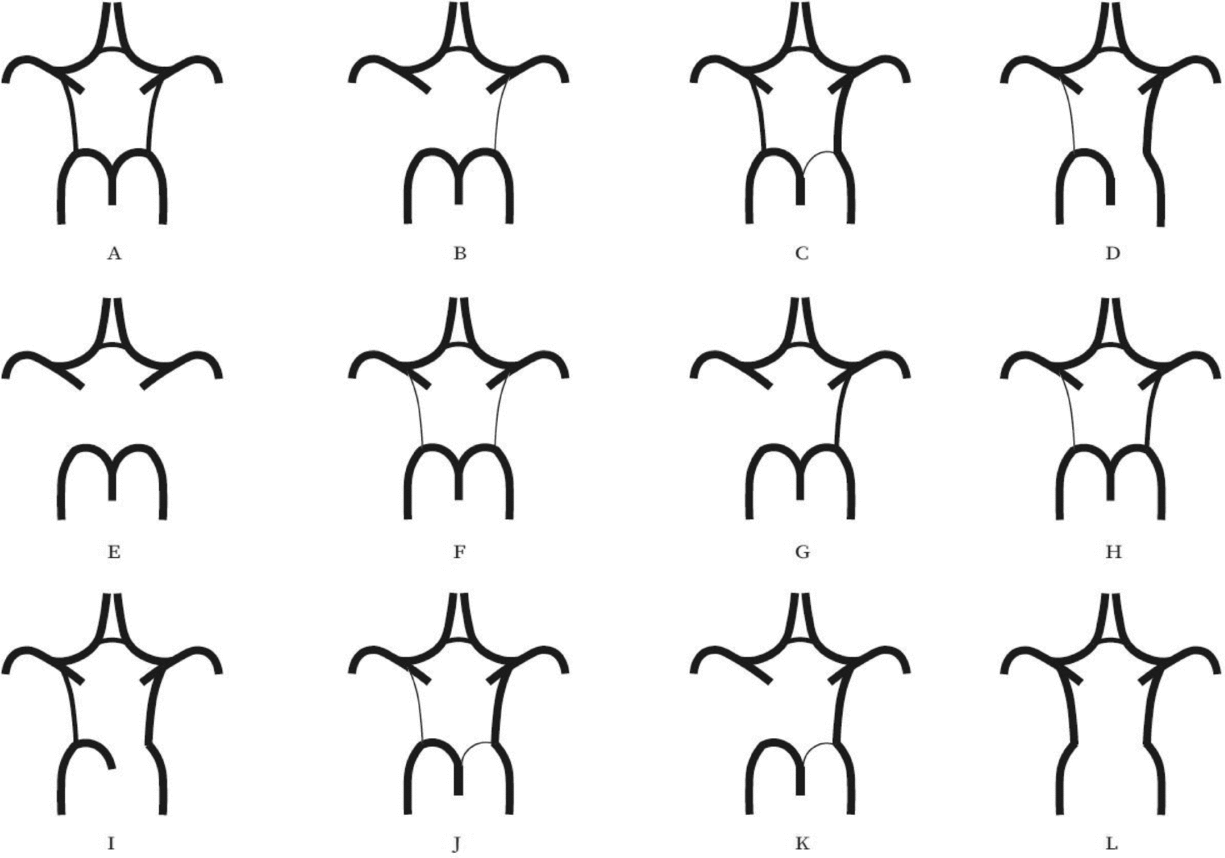
FIGURE 2 Variants of the posterior part of the circle of Willis. (A) “Normal” configuration with non-hypoplastic PCoAs present on both sides. (B) PCoA hypoplasia on one side, absent contralateral PCoA. (C) Unilateral fetal-type variant with ipsilateral dominant PCoA and hypoplastic PCA P1 segment. (D) Unilateral fetal-type variant with absent ipsilateral PCA P1 segment and contralateral PCoA hypoplasia. (E) Bilaterally absent PCoAs. (F) Bilaterally hypoplastic PCoAs. (G) Unilaterally absent PCoA. (H) Unilaterally hypoplastic PCoA. (I) Unilateral fetal-type variant with ipsilateral absent PCA P1 segment. (J) Unilateral fetal-type variant with ipsilateral hypoplastic PCA P1 segment and contralateral hypoplastic PCoA. (K) Unilateral fetal-type variant with ipsilateral hypoplastic PCA P1 segment and contralateral absent PCoA. (L) Bilateral fetal-type variant with bilaterally absent PCA P1 segments. PCA = posterior cerebral artery, PCoA = posterior communicating artery.
Twin studies aim to determine the genetic and environmental basis of a certain phenotypic variance. The concept of twin studies is based on the assumption that monozygotic (MZ) twins share 100% of their genes on average, whereas dizygotic (DZ) twins share 50% of their genes similarly to non-twin siblings. The similarity between co-twins is estimated with the concordance rate in case of categorical variables (which were used in the current study for CW variants). If MZ twins have more similar phenotypes (and thus higher concordance rate) than DZ twins, then there is a bigger contribution to the phenotypic variance by genetic effects. Furthermore, twins share the same early environment, and therefore, common and non-common environmental factors can be estimated. In the current study, this detailed analysis was not possible due to the small study population. Therefore, concordance and discordance rates were calculated (Sahu & Prasuna, Reference Sahu and Prasuna2016).
We determined the concordance rates of anterior and posterior CW morphologies separately. Furthermore, we calculated concordance rates for the presence of hypoplastic or absent vessels in the CW. Pairwise concordance rates were estimated by the following formula (Witte et al., Reference Witte, Carlin and Hopper1999):

A pair of twins was defined as concordant if both members of the pair had any kind of variant CW anatomy. In discordant twin pairs, one member of the twin pair had classical CW anatomy and the other member had variant anatomy in the CW. Both anterior and posterior CW parts were investigated separately according to these categories.
Comparison of the MZ and DZ groups regarding BMI and age was evaluated with an independent samples t test. Similarly, an independent samples t test was used to compare the BMI among MZ discordant twins with and without CW variants. A chi-square test was applied to investigate differences between MZ and DZ twins and MZ discordant twins for smoking, diabetes, hypertension, and hypercholesterolemia. We analyzed intrapair differences in the MZ discordant group regarding flow parameters recorded with TCD using paired t tests. Statistical Package for the Social Sciences (SPSS) software (IBM SPSS Statistics for Windows, Version 24.0, Released 2016, IBM Corp., Armonk, New York) was used for statistical analysis.
Results
A total of 122 twins attended the study (61 pairs), of whom 39 pairs were MZ and 22 were DZ after the exclusion of 4 twin pairs. These twin pairs had to be excluded either because they did not show up at the scheduled appointment for MRI (three pairs) or because of claustrophobia (one pair). The average age was 48.4 and 52.0 years in the MZ and DZ groups, respectively. There was no significant difference regarding average age between the two groups (p = .16). Similarly, no significant difference was observed between the MZ and DZ groups regarding anthropometric variables and risk factors, except for hypercholesterolemia (p = 0.01). Table 1 shows the baseline characteristics of our population. Supplementary Tables S1 and S2 show the prevalence of variants of the anterior and posterior CW. The most common anterior CW configuration was the “normal” form of the CW without hypoplasticity or absence in the ACA and anterior communicating artery (ACoA) (A, 45.0%) followed by hypoplastic ACoA (D, 25.8%) and absent ACoA (E, 10.8%). Among posterior CW configurations, bilaterally missing PCoA (E, 22.5%) was the most commonly observed variant. Normal morphology (A, 17.5%), bilaterally hypoplastic PCoA (F, 15.0%), and unilaterally hypoplastic PCoA (H, 11.7%) were also among the most common configurations. The total percentage of unilateral fetal configuration (C, D, I, J, and K) was seen in 10.9%. No significant difference was observed regarding anterior and posterior CW variants shown in Figures 1 and 2 between the MZ and DZ groups with one exception: the bilaterally hypoplastic PCoA (F variant) appeared in a significantly higher rate in the DZ group (p = .02).
TABLE 1 Baseline Characteristics of Our Study Population

Note: BMI = body mass index; DZ = dizygotic; MZ = monozygotic.
Data are shown as mean ± standard deviation with continuous variables.
Table 2 shows the concordance and discordance rates of the anterior and posterior CW variants according to the morphological classification. MZ concordance was not higher than DZ concordance in anterior and posterior variants, which presumes the dominance of environmental factors on CW configurations. Figures 3 and 4 show examples of differences in CW configurations in MZ twins.
TABLE 2 Concordance Rates of the Anterior and Posterior Anatomies of the Circle of Willis According to Morphological Aspects

Note: CW = circle of Willis; DZ = dizygotic; MZ = monozygotic.

FIGURE 3 Differences in anterior CW variants of a monozygotic twin pair. Twin A has a right-sided hypoplastic ACA A1 segment (arrow). Twin B has normal anterior CW configuration. ACA = anterior cerebral artery, CW = circle of Willis.

FIGURE 4 Differences in posterior CW variants of the same monozygotic twin pair as shown in Figure 3. Twin A has bilateral fetal configuration (asterisk) with absent PCA P1 segments on both sides. The arrows in the left picture point to the superior cerebellar arteries. Twin B has bilaterally absent PCoAs. The arrows in the right picture show the anterior choroidal arteries. PCA = posterior cerebral artery, PCoA = posterior communicating artery, CW = circle of Willis.
Out of our MZ twin group, 23 twin pairs were discordant regarding anterior or posterior CW variants. We analyzed which environmental effect could have a significant impact on the development of anterior or posterior CW variants in discordant MZ twin pairs. Smoking, diabetes, hypertension, and hypercholesterolemia did not differ significantly between MZ discordant twins with variant and classical CW configuration (Supplementary Table S3). Flow parameters, such as MFV and PI, did not show a significant difference within twin pairs of the MZ discordant group in any segment of the CW (Supplementary Table S4) in our healthy subjects, except for the right ACA.
Discussion
Our results suggest that CW variants seem to be determined by environmental factors rather than genetic effects. The presence of anterior and posterior CW variants was not significantly associated with current BMI, hypertension, and hypercholesterolemia, suggesting that these factors do not affect the development of the cerebrovascular variants. Also, there was no significant difference regarding cerebral blood flow in MZ twins discordant to CW variants, indicating that these variants may not alter the flow in healthy individuals.
In a recent study, heritability of CW variants was assessed for the first time in humans in the frame of a family study (Sanchez van Kammen et al., Reference Sanchez van Kammen, Moomaw, van der Schaaf, Brown, Woo, Broderick and Ruigrok2018). According to the results, the occurrence of PCoA was significantly higher within families with intracerebral aneurysms, and this was not the case regarding ACA A1 asymmetry, fetal configuration, and the classical morphology (Sanchez van Kammen et al., Reference Sanchez van Kammen, Moomaw, van der Schaaf, Brown, Woo, Broderick and Ruigrok2018). Comparing these findings to our results is challenging due to the different study designs and because our study included healthy individuals, but it is unlikely that genetic pre-disposition is present for only one variant. Furthermore, it is also possible that the findings are simply due to the higher general prevalence of PCoA variants, since hypoplasia or absence of PCoA is the most commonly observed CW variant (De Silva et al., Reference De Silva, Silva, Amaratunga, Gunasekera and Jayesekera2011; Eftekhar et al., Reference Eftekhar, Dadmehr, Ansari, Ghodsi, Nazparvar and Ketabchi2006; Hashemi et al., Reference Hashemi, Mahmoodi and Amirjamshidi2013; Klimek-Piotrowska et al., Reference Klimek-Piotrowska, Kopec, Kochana, Krzyzewski, Tomaszewski, Brzegowy and Walocha2013).
What environmental factors may be behind CW variants is yet to be examined. Our results show that environmental factors may determine CW anatomy; however, we could not confirm any significant association between classical cardiovascular risk factors at study time and the presence of CW variants. Our volunteer twin population consisted of healthy individuals. Results on the association of cardiovascular risk factors and CW variants are controversial in the literature. A recent animal study showed that chronically elevated blood pressure and aging decreased PCoA diameter in inbred mice, but obesity, hyperlipidemia, metabolic syndrome, and diabetes mellitus did not affect this arterial diameter (Faber et al., Reference Faber, Zhang, Rzechorzek, Dai, Summers, Blazek and Hedges2018). Jin et al. (Reference Jin, Dong, Cai, Zhang, Zhang, Gao and Yang2016) found that incomplete posterior circulation was present in subjects without hypertension more commonly than in those with hypertension. Although we did not investigate gender differences in CW variants, Zaninovich et al. (Reference Zaninovich, Ramey, Walter and Dumont2017) found that complete CW differed significantly between men and women above the age of 39 years, suggesting that age combined with other environmental factors may have a significant impact. Another study did not find any significant association between cardiovascular risk factors and the number of hypoplastic arteries in the CW, in line with our findings, except for increasing age (Gutierrez et al., Reference Gutierrez, Sultan, Bagci, Rundek, Alperin, Elkind and Wright2013). Significant differences among age groups were described, with incomplete CW being more common with increasing age (El-Barhoun et al., Reference El-Barhoun, Gledhill and Pitman2009; Li et al., Reference Li, Li, Lv, Li, Luo and Xie2011; Zaninovich et al., Reference Zaninovich, Ramey, Walter and Dumont2017). Our population was relatively young (average age 50.4 ± 13.6 years), and therefore, the prevalence of CW variants may not be high enough for comparison. Our findings could also be explained by the small population size, which limited us to perform a more detailed heritability analysis.
The high number of MZ discordant twins may be explained by epigenetic effects. These interactions between the genes and the environment might lead to changes in phenotypes. Although twins share the same uterus, it does not necessarily mean that they share the same in-utero environment, since the fetoplacental unit might be unique in each member of the twin pair (Czyz et al., Reference Czyz, Morahan, Ebers and Ramagopalan2012). Hypoxia and metabolic effects could have an influence on CW variants already at intrauterine development and in adult life. Changes in the volume of the amniotic fluid, blood flow, and hemodynamic circumstances during brain development were shown to influence calibers of the intracranial vessels (Hillen, Reference Hillen1986; Mari et al., Reference Mari, Wasserstrum and Kirshon1992; Van Overbeeke et al., Reference Van Overbeeke, Hillen and Tulleken1991). The findings of Hartkamp et al. (Reference Hartkamp, van Der Grond, van Everdingen, Hillen and Mali1999) underline the importance of hemodynamic and metabolic factors later in adult life as patients with ICA stenosis of 70–99% or occlusion of the ICA tend to have complete CW on the side of the stenosis or occlusion, and this corresponds with the results of a study conducted on patients who underwent carotid endarterectomy or stenting, which demonstrated that 1 month after the operation (once the need for collateral supply decreased), collaterals of the CW became reduced in size (Bost et al., Reference Bost, Hendrikse, Algra, de Borst, Kappelle, Jongen and van der Worp2014).
Term and pre-term birth (Malamateniou et al., Reference Malamateniou, Adams, Srinivasan, Allsop, Counsell, Cowan and Rutherford2009), intrauterine circumstances, and certain diseases during pregnancy may also influence CW configurations of the fetus. Pre-eclampsia leads to deficiency in placental growth factor (PGF), which has an impact on cerebrovascular development in mice: Unilaterally hypoplastic CW and a decreased number of collaterals were noted in PGF-/- mice (Luna et al., Reference Luna, Kay, Ratsep, Khalaj, Bidarimath, Peterson and Croy2016). These results should stimulate further research regarding the presence of CW variations early in life. Future studies should investigate whether circumstances of delivery (e.g., cardiotocography monitoring or data regarding cesarean section) are associated with the presence of CW variants.
Li et al. (Reference Li, Huo, Zhang, Lu, Li, Guo and Chen2015) identified four genes in gerbils that could possibly play a role in the formation of CW variants. Another study, also conducted on gerbils, investigated CW variants through generations and concluded that configurations of ACoA are heritable, mainly from the mother's side, which was not shown in the case of posterior variants (Du et al., Reference Du, Zhu, Dong, Lu, Wang, Zeng and Chen2011). On the other hand, Beckmann (Reference Beckmann2000) described individual differences among mice within the same strain. The differences mainly involve the presence of PCoA (Beckmann, Reference Beckmann2000), which corresponds with our findings in humans. Since our findings do not support genetic pre-disposition to CW variants, we speculate whether epigenetic effects could have a more significant influence. Epigenetic effects play a substantial role in the evolution of different phenotypes, as described by the Waddington (Reference Waddington1957) epigenetic landscape metaphor. According to this theory, the development of cells can be regarded as a pathway with several junctions leading to different phenotypes. It is possible that the various CW variants are the results, at least partly, of a similar process. There are genetic processes that initiate development of cerebral arteries, but as the occipital lobe grows in the human brain, its functional demand leads to hemodynamic changes, and thus, CW variations may arise as consequence of these effects (Van Overbeeke et al., Reference Van Overbeeke, Hillen and Tulleken1991). This is in line with the concept of angioadaptation, which is an adaptive change of the vessel wall structure and its continuous interaction with the environment due to hemodynamic, metabolic, and molecular inputs (le Noble et al., Reference le Noble, Klein, Tintu, Pries and Buschmann2008; Zakrzewicz et al., Reference Zakrzewicz, Secomb and Pries2002). Thus, the presence of variants could depend heavily on the development of the arteries (Lasjaunias et al., Reference Lasjaunias, Berenstein and ter Brugge2001). Furthermore, there might be developmental interactions between the CW and the skull structure, as described by Jamniczky and Hallgrimsson (Reference Jamniczky and Hallgrimsson2011). The findings of Faber et al. (Reference Faber, Zhang, Rzechorzek, Dai, Summers, Blazek and Hedges2018) in mice underline the importance of random stochastic factors on PCoA variants in the developmental phase, since low birth weight was not significantly associated with the presence of CW variants. Aging and hypertension, but not other cardiovascular risk factors, influenced PCoA diameter significantly, and the PCoA configuration differed among mice strains with different genetic background (Faber et al., Reference Faber, Zhang, Rzechorzek, Dai, Summers, Blazek and Hedges2018). Thus, it is suggested in this article that genetic and stochastic factors are the primary determinants of PCoA configuration, which is then further affected by environmental factors later in life (Faber et al., Reference Faber, Zhang, Rzechorzek, Dai, Summers, Blazek and Hedges2018).
According to our results, the presence of CW variants does not influence the cerebral flow among healthy individuals with non-pathologic circumstances, underlying the fact that the clinical manifestation of the presence of CW variants is expected in low cerebral blood flow states, such as in ICA stenosis or occlusion (Hoksbergen, Legemate et al., Reference Hoksbergen, Legemate, Csiba, Csati, Siro and Fulesdi2003; Park et al., Reference Park, Kim, Lee, Chun, Kim, Lee and Kim2018). Recent results pointed out the association between compromized CW and brain ischemia in a population with high-grade ICA stenosis (Varga et al., Reference Varga, Di Leo, Banga, Csobay-Novak, Kolossvary, Maurovich-Horvat and Huttl2018), and especially the failure of collateral flow may pre-dispose to ischemic events (Hoksbergen et al., Reference Hoksbergen, Legemate, Csiba, Csati, Siro and Fulesdi2003; Varga et al., Reference Varga, Di Leo, Banga, Csobay-Novak, Kolossvary, Maurovich-Horvat and Huttl2018). TCD and MRA findings have not been compared in healthy individuals until this report; however, findings of these two modalities correlated in patients with intracerebral arterial pathology (Han et al., Reference Han, Feng, Han, Liu, Zhang, Yang and Duan2014; Hendrikse et al., Reference Hendrikse, Klijn, van Huffelen, Kappelle and van der Grond2008).
Our study has limitations that have to be acknowledged. The relatively small population may influence our results, which limited us to perform complex genetic analysis, identifying the common and unique environmental factors due to low power (ACE modeling). The comparison of concordance rates alone does not allow conclusions regarding heritability. A high concordance does not mean that the phenotype is heritable, and conversely, low concordance rates do not exclude genetic effects (van Dongen et al., Reference van Dongen, Slagboom, Draisma, Martin and Boomsma2012). Our imaging method (TOF MRA) can be regarded as a limitation factor of this study because the longer acquisition time increases the motion artefacts on a TOF MRA image. Contrast-enhanced MRI has better resolution and is more sensitive to detect the CW variants, but it is an invasive procedure and requires contrast administration, which is ethically not acceptable in a healthy volunteer population. Moreover, previous studies found no significant difference between contrast-enhanced MRA and TOF MRA regarding displaying intracranial arteries (Alfke et al., Reference Alfke, Jensen, Pool, Rohr, Bruning, Weber and Jansen2011; Heiserman et al., Reference Heiserman, Drayer, Keller and Fram1992). Detecting ACA, MCA, and PCA with TOF MRA has a sensitivity of 94–100% compared with the gold standard method, i.e., digital subtraction angiography. The value is lower (65%) in case of anterior and posterior communicating arteries (Stock et al., Reference Stock, Wetzel, Kirsch, Bongartz, Steinbrich and Radue1996). This may explain why in our study population absent or missing ACoA and PCoA were the most common variants. TOF MRA is useful in the diagnosis of stenoocclusive disease as sensitivity and specificity of TOF MRA of the MCA compared to conventional angiography were 88% and 97%, respectively (Korogi et al., Reference Korogi, Takahashi, Mabuchi, Miki, Shiga, Watabe and Fujiwara1994). In addition, contrast-enhanced MRA has the risk of gadolinium accumulation in the brain and bone tissue, even in patients with normal renal function (Kanda et al., Reference Kanda, Oba, Toyoda, Kitajima and Furui2016), and therefore, it was not an option in the current study.
Conclusion
Our study is the first to investigate genetic versus environmental background of the CW variants with MRI in the frame of a twin study. Our results show that anatomical variants of the CW, which commonly occur and might pre-dispose to cerebrovascular events, might be shaped and influenced by environmental or stochastic factors; however, our results do not allow us to draw conclusions on heritability. Anterior and posterior CW variants were not associated with current BMI, smoking, hypertension and hypercholesterolemia, and altered blood flow using TCD. Since CW variants are known risk factors of cerebrovascular ischemia, determining their origin is essential to develop preventive strategies. Our results should stimulate further research on the origin of CW variants in larger twin populations.
Acknowledgments
No financial support can be stated. The authors acknowledge the help of MR operators. The authors thank Dr Philip Auyang for English language editing.
Disclosure of Interest
None.
Supplementary material
To view supplementary material for this article, please visit https://doi.org/10.1017/thg.2018.50