Introduction
Bambara groundnut (BGN) (Vigna subterranea L. Verdc.; Syn: Voandzeia subterranean L. Thouars.) (2n = 22) is a highly inbred, self-pollinating annual crop belonging to the Fabaceae family and subfamily Faboidea under Plantae (Bamshaiye et al., Reference Bamshaiye, Adegbola and Bamshaiye2011). In-breeding and self-pollination result in increased homozygosity and restricted gene flow which decreases genetic diversity in crops (Sharma, Reference Sharma2017). The crop is indigenous to Africa where it is known by different names such as earth pea, jugo bean, nyimo beans or ditloo (Majola et al., Reference Majola, Gerrano and Shimelis2021). Other common universal names are Bambara nut, Bambara bean, Congo goober, earth pea, ground-bean and hog-peanut. The plant resembles peanuts in having compound leaves with three leaflets (Bamshaiye et al., Reference Bamshaiye, Adegbola and Bamshaiye2011) and bearing the fruits underground.
It is an underutilized (syn minor, neglected or orphan) protein-rich (18–26%), legume mainly grown by smallholder and low-income female subsistence farmers in poor communities in sub-Saharan Africa, under arid or semi-arid conditions (Jideani and Mpotokwane, Reference Jideani and Mpotokwane2009; Bamshaiye et al., Reference Bamshaiye, Adegbola and Bamshaiye2011; Yao et al., Reference Yao, Kouassi, Erba, Scazzina, Pellegrini and Casiraghi2015). BGN is largely drought, disease and pest resistant, with the capability of thriving under nutrient-deficient soils (Olayide et al., Reference Olayide, Donkoh, Ansah, Adzawla, O'Reilly, Mayes, Feldman, Halimi, Nyarko, Ilori, Alabi and Leal Filho2018; Mayes et al., Reference Mayes, Ho, Chai, Gao, Kundy, Mateva, Zahrulakmal, Hahiree, Kendabie, Licea, Massawe, Mabhaudhi, Modi, Berchie, Amoah, Faloye, Abberton, Olaniyi and Azam-Ali2019; Pasipanodya et al., Reference Pasipanodya, Horn, Achigan-Dako, Musango and Sibiya2022). In terms of production, socioeconomic status and consumption, it ranks third amongst legumes in Africa after groundnut (Arachis hypogaea) and cowpea (Vigna unguiculate) (Murevanhema and Jideani, Reference Murevanhema and Jideani2013). It is used for human consumption as an important source of protein, especially for vegetarians and poor households who cannot afford meat-based protein sources, but it is also useful as fodder for livestock. Varieties such as South African genotypes SB11-1A, SB19-1A, SB12-3B and Bambara-12 with good vegetative biomass have been identified and these are more useful for livestock feed (Unigwe et al., Reference Unigwe, Gerrano, Adebola and Pillay2016).
Its centre of origin can be traced to north-eastern Nigeria and northern Cameroon where undomesticated wild relatives still exist (Mayes et al., Reference Mayes, Ho, Chai, Gao, Kundy, Mateva, Zahrulakmal, Hahiree, Kendabie, Licea, Massawe, Mabhaudhi, Modi, Berchie, Amoah, Faloye, Abberton, Olaniyi and Azam-Ali2019). However, an alternative or a potential secondary centre of domestication, evolution or diversification for the crop has been proposed to be the Southern/Eastern African region (Aliyu et al., Reference Aliyu, Massawe and Mayes2016). Due to different centres of origin, two subpopulations have been proposed (Pasipanodya et al., Reference Pasipanodya, Horn, Achigan-Dako, Musango and Sibiya2022), with subpopulation I consisting of West and Central African accessions, whilst subpopulation II consists of the Southern and Eastern African accessions (Molosiwa et al., Reference Molosiwa, Aliyu, Stadler, Mayes, Massawe, Kilian and Mayes2015). There are opportunities for broadening the genetic diversity by looking for favourable genes from these centres of origin. The current cultivated species was probably domesticated from these wild species. The value of wild species, despite challenges with cross-ability and/or hybridization, is immense even in legumes (Maphosa et al., Reference Maphosa, Richards, Norton and Nguyen2020).
The main limitations of their use in breeding are incompatibility and linkage drag of detrimental genes which compromise agronomic performance and grain yield (Bohra et al., Reference Bohra, Kilian, Sivasankar, Caccamo, Mba, McCouch and Varshney2021).
There is a high level of phenotypic and allelic diversity in the BGN germplasm across genotypes from similar and/or different geographic areas of origin (Aliyu et al., Reference Aliyu, Massawe and Mayes2016; Unigwe et al., Reference Unigwe, Gerrano, Adebola and Pillay2016; Ontong et al., Reference Ontong, Gerrano and Labuschagne2021). Agromorphological variability is noticeable in BGN, with the wild types displaying more spreading growth habit, which is a dominant trait compared with the domesticated bunchy types (Basu et al., Reference Basu, Mithen and Pasquet2007). Its growth habit ranges from erect to spreading, and leaf shape varies between genotypes. It also shows indeterminacy as flowering can continue until maturity, enabling both vegetative and reproductive phases to co-occur and/or overlap. This means that vegetative and reproductive phases can be exposed to unfavourable and stressful growth conditions simultaneously.
The changing climate and a growing world population are putting pressure on food production, as world food supply is heavily reliant on few crops. Production of all crops will have to increase by at least 70% to ensure food security for the estimated nine billion people by 2050 (Godfray et al., Reference Godfray, Beddington, Crute, Haddad, Lawrence, Muir and Toulmin2010). As such, in addition to major cereal crops such as wheat (Triticum aestivum), rice (Oryza sativa) and maize (Zea mays), there is a need to broaden crop usage and increase yield of minor crops. Importantly, there is now increasing emphasis not only on food availability but also on its quality and nutritional value. The increased utilization of BGN is predicted to play a significant role in increasing food security, alleviating malnutrition, lessening over utilization of commercial legumes such as beans, lentils, chickpeas and soybeans, as well as in being a valuable ingredient in the production of affordable nutritious products (Shanmugasundaram, Reference Shanmugasundaram2003).
This review aims to provide the current status of BGN production, its grain composition and its nutritional value. It identifies production constraints and discusses approaches as well as modern technologies to overcome these barriers. It further discusses opportunities for overall improvement, so that BGN, like all neglected crops, can play a valuable role in world food security.
Bambara groundnut production
Despite being an agronomically and nutritionally important crop, that is cheap to cultivate, with minimal inputs even under poor sandy soils and drought-prone environments, BGN remains relatively underutilized. This may be attributed to the perception that it is of low economic value, thus often termed a ‘poor man's crop’ (Azam-Ali et al., Reference Azam-Ali, Sesay, Karikaris, Massawe, Bannayan and Hampton2001). As such, farmers designate a very low amount of the total area for its cultivation and give priority to higher market value crops such as maize, peanuts and sorghum (Shanmugasundaram, Reference Shanmugasundaram2003). Most production, especially in sub-Saharan Africa, is by small-scale farmers who have minimal to no record-keeping skills. This, together with lack of accessibility to the farmers by data collectors, compromises or underestimates the total yield and production area. Long-term data in Africa show that yields range from 0.5 to 3 t/ha (Azam-Ali et al., Reference Azam-Ali, Sesay, Karikaris, Massawe, Bannayan and Hampton2001) and are on average 0.85 t/ha and with a total of 0.3 million tonnes produced per annum (Mayes et al., Reference Mayes, Ho, Chai, Gao, Kundy, Mateva, Zahrulakmal, Hahiree, Kendabie, Licea, Massawe, Mabhaudhi, Modi, Berchie, Amoah, Faloye, Abberton, Olaniyi and Azam-Ali2019). However, yields of less than 0.1 t/ha in some fields have also been reported (Baudoin and Mergeai, Reference Baudoin, Mergeai and Raemaeker2001). There is a significant yield gap between the average yields of 0.85 t/ha and potential yields of over 3 t/ha that can be achieved when production is optimized (Mayes et al., Reference Mayes, Ho, Chai, Gao, Kundy, Mateva, Zahrulakmal, Hahiree, Kendabie, Licea, Massawe, Mabhaudhi, Modi, Berchie, Amoah, Faloye, Abberton, Olaniyi and Azam-Ali2019). The low yields are attributed to limited research investment on genetic, agronomic and management improvements (Majola et al., Reference Majola, Gerrano and Shimelis2021). Amongst the common legumes, the average yield of 0.85 t/ha is only higher than that of cowpea, dry beans and Pigeon pea, and the 0.3 million tonnes annum production is similar to chickpea but only higher than lentils (Mayes et al., Reference Mayes, Ho, Chai, Gao, Kundy, Mateva, Zahrulakmal, Hahiree, Kendabie, Licea, Massawe, Mabhaudhi, Modi, Berchie, Amoah, Faloye, Abberton, Olaniyi and Azam-Ali2019). The drivers of crop productivity are genotypes (varieties), environment (production conditions) and management practices, and their interactions. Therefore, to close the yield gap there is a need to focus the research on understanding the contribution of each component and optimizing them.
The major producers of BGN (Table 1) are the West African countries (FAOSTAT, 2018), with Burkina Faso having the largest yield but not necessarily the biggest area harvested. The Democratic Republic of the Congo has the lowest yield while Niger has the largest harvested area. BGN is also grown in other sub-Saharan African countries such as South Africa, Swaziland, Zimbabwe, Zambia and Botswana (Masindeni, Reference Masindeni2006; Majola et al., Reference Majola, Gerrano and Shimelis2021), but probably the scale is too small for FAO to capture it. Furthermore, it is grown, albeit at smaller scale, beyond African borders in Southeast Asia mainly in Indonesia and Thailand. Therefore, it is able to grow in a range of different and/or contrasting environments, such as the arid environment in Botswana which experiences low night temperatures and high day temperatures as well as in milder and more humid environments such as in Indonesia (Mayes et al., Reference Mayes, Ho, Chai, Gao, Kundy, Mateva, Zahrulakmal, Hahiree, Kendabie, Licea, Massawe, Mabhaudhi, Modi, Berchie, Amoah, Faloye, Abberton, Olaniyi and Azam-Ali2019).
Table 1. Total area harvested, production and average yields for Bambara groundnut in 2019
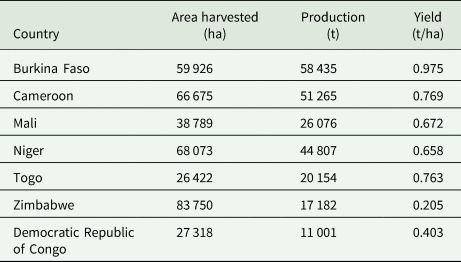
Source: FAOSTAT (2018).
Globally, and represented by the six major producers (Table 1), production and cultivated areas have increased while yields have remained largely stagnant (Fig. 1). Production has been steadily increasing from a low of 66 485 tonnes in 1995 to a high of 236 276 tonnes in 2018 but this was largely underpinned by an increase in total harvest area, which also steadily increased from 91 653 hectares in 1998 to 376 595 hectares in 2017. However, in the past 25 years, yield gains (averaged across the six major producers, and seven from 2012 with the addition of Zimbabwean data) have been stable ranging between 0.57 and 0.85 t/ha. In 2008, average yield was 1.14 t/ha and this was driven by a very high yield of 3.4 t/ha in Togo. The 25-year average yield in Togo is 0.851 t/ha and when 2008 is excluded the average is 0.771 t/ha. Assuming the yield data for 2008 is accurate, then there is potential to improve it and close the yield gap by harnessing the approaches adopted in Togo in 2008. The stagnation of yield indicates that in the past two decades there has been less attention and/or attempt to close the yield gap through concerted and coordinated research, management practices and use of improved varieties.
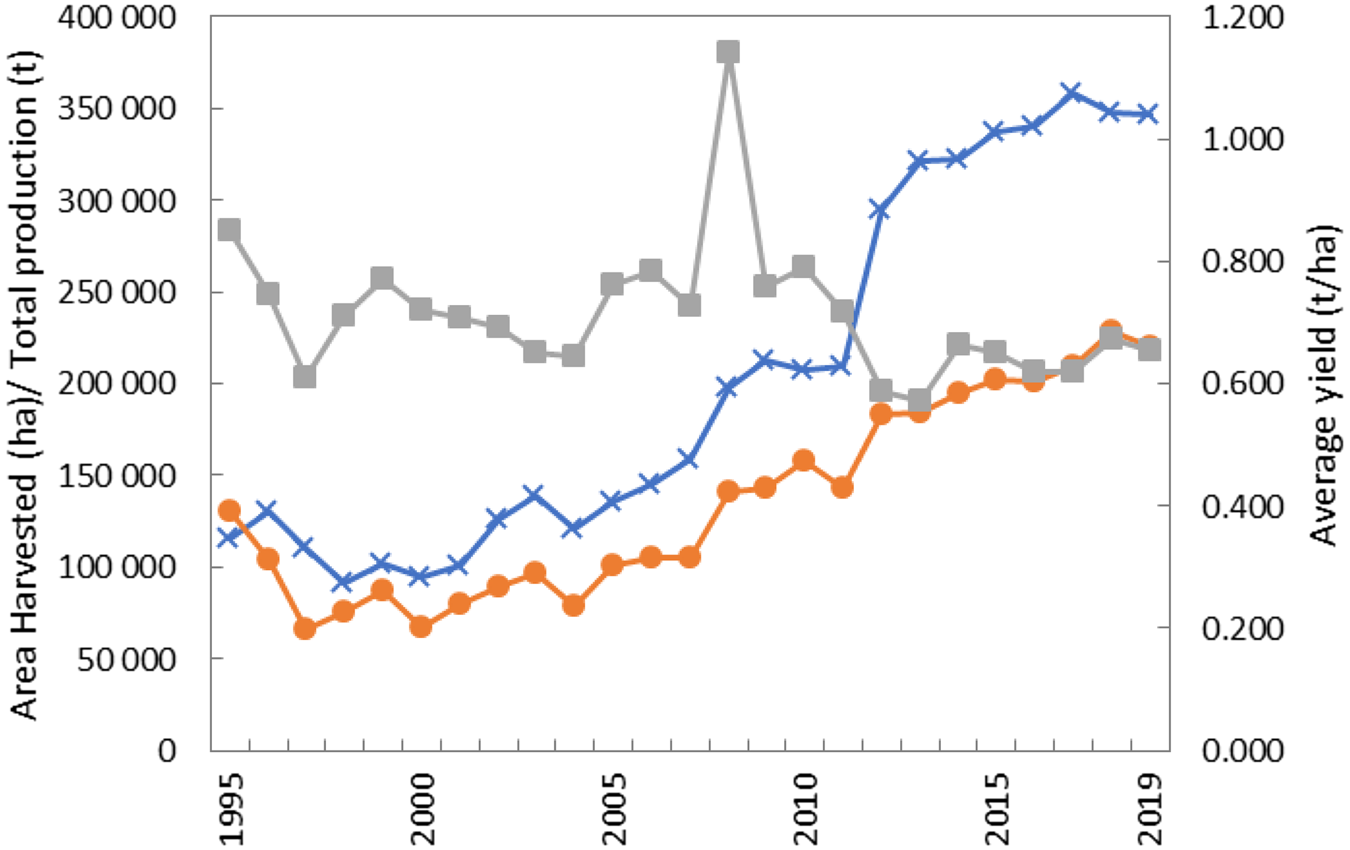
Fig. 1. Colour online. Global [represented by six* (and seven** from 2012) major producers] total production, cultivated and average yield of Bambara groundnut over a period of 25 years.
Within agricultural systems, BGN is grown either in mixed/inter cropping with cereals, other legumes or as a standalone crop in rotation. As a legume, it has the capacity to fix atmospheric nitrogen thereby contributing to soil fertility and minimizing the need for artificial fertilizers (Mayes et al., Reference Mayes, Ho, Chai, Gao, Kundy, Mateva, Zahrulakmal, Hahiree, Kendabie, Licea, Massawe, Mabhaudhi, Modi, Berchie, Amoah, Faloye, Abberton, Olaniyi and Azam-Ali2019). It can fix between 32 and 81 kg of nitrogen per ha (Majola et al., Reference Majola, Gerrano and Shimelis2021). The varieties mostly grown are landraces, where farmers use their own saved or locally procured seeds, an indication of lagging behind in terms of breeding progress and uptake of available commercial varieties.
BGN has a well-developed taproot with several lateral stems up to 20 cm long, from which leaves grow (Bamshaiye et al., Reference Bamshaiye, Adegbola and Bamshaiye2011). The leaves are trifoliate and can be up to 5 cm long. Their petiole is grooved, up to 15 cm long and their base is either green or purple in colour (Gulu, Reference Gulu2018). BGN flowers are typically papilionaceuos and grow in a raceme on long, hairy peduncles, which arise from the nodes on the stem (Massawe et al., Reference Massawe, Mwale, Azam-Ali and Roberts2005). Cross-pollination of the spreading type is commonly done by ants while the branching type usually self-pollinates. After fertilization, the flower stem elongates, the sepal enlarges and the fruit develops just above or just below the soil surface (Gulu, Reference Gulu2018). This makes mechanical harvesting very difficult, and as a result BGN is commonly handpicked, a major drawback when it comes to large-scale harvesting (Gulu, Reference Gulu2018). The pods are very small, about 1.5 cm in size depending on variety. They are wrinkled, round or semi-oval in shape and house one or two seeds (Bamshaiye et al., Reference Bamshaiye, Adegbola and Bamshaiye2011). The unripe pods are yellow-green while the mature pods are yellowish, reddish, dark brown or purple (DAFF, 2011).
Bambara groundnut varieties
There is a large variation in BGN seeds as demonstrated by different seed colours and patterns (Fig. 2). Seven seed types have been reported, namely cream with brown-eye, cream with black-eye, red, cream with no eye, black, purple and speckled/flecked/spotted (Diedericks, Reference Diedericks2014). However, the most common type of BGN seeds is the cream varieties in sub-Saharan Africa. The black and purple varieties are common in Indonesia while the red seed is common in Thailand. The colour of the testa varies according to ripeness and variety, with colours such as cream, black, red and purple.
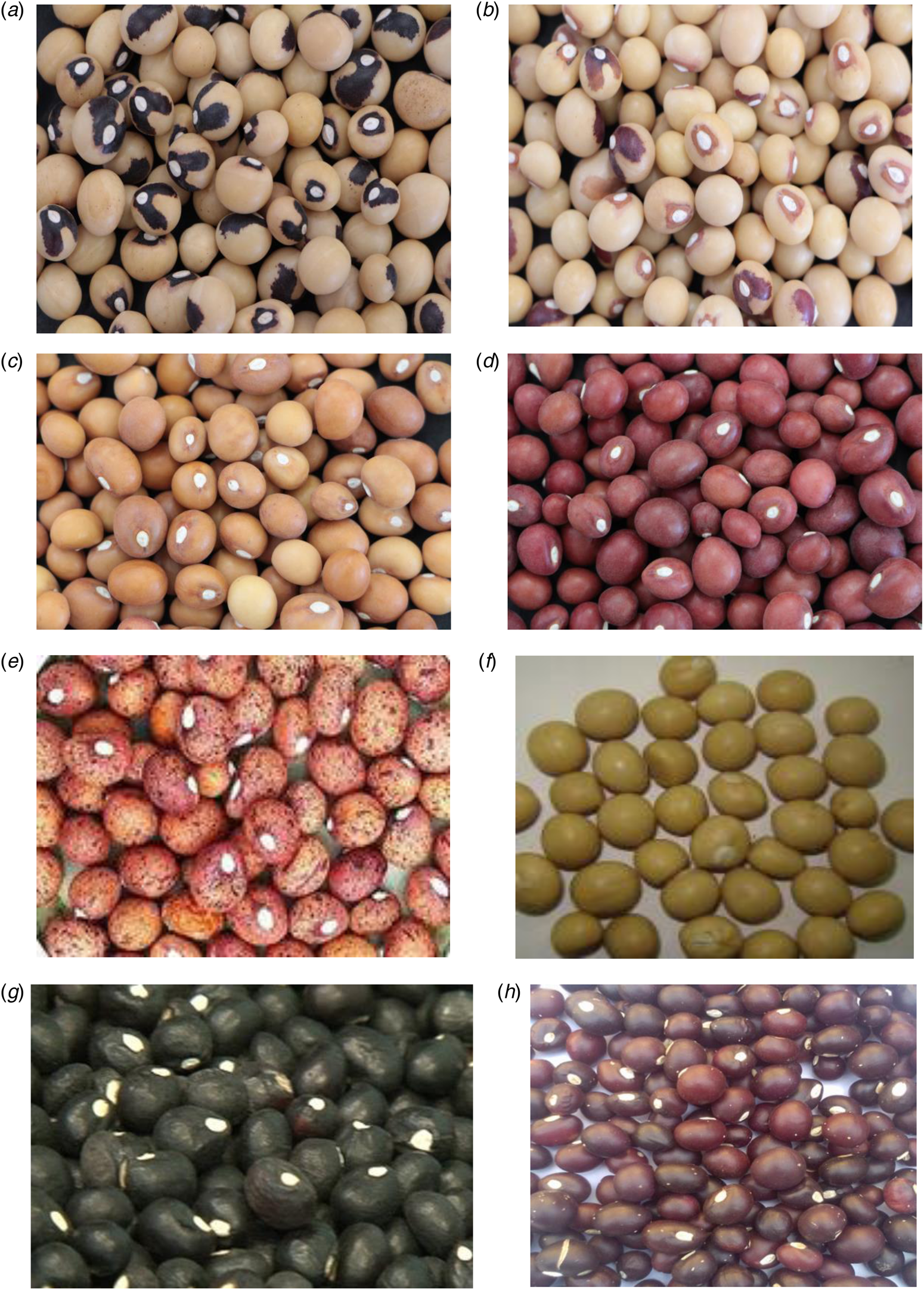
Fig. 2. Colour online. Different varieties of Bambara groundnut seeds: (a) cream black-eye, (b) cream brown-eye, (c) brown, (d) red, (e) speckled/spotted, (f) cream no-eye, (g) black, (h) purple (Diedericks, Reference Diedericks2014).
The cream with black-eye as well as the cream with brown-eye are generally high yielding and have large (>10.50 mm) and medium (9.50–10.49 mm) sized kernels, respectively, while the cream with no-eye are poor yielding, and have very small (<9.50 mm) kernels (Mpotokwane et al., Reference Mpotokwane, Gaditlhatlhelwe, Sebaka and Jideani2008; DAFF, 2011). The brown variety has medium (9.50–10.49 mm) to large (>10.50 mm) sized kernels varying between light and dark brown in colour (Mpotokwane et al., Reference Mpotokwane, Gaditlhatlhelwe, Sebaka and Jideani2008; Maphosa, Reference Maphosa2016). The black variety is early maturing and has small to medium-sized (9.50–10.49 mm) kernels. The purple and speckled varieties have one-seeded pods and small (<9.50 mm) kernels (Anon., 2011; DAFF, 2011). The red variety is late maturing, has large (>10.50 mm) kernels and is prone to rotting onsite (DAFF, 2011). The red variety has been reported to have almost double the amount of iron as the cream varieties and, hence, it can be useful in curbing iron deficiency (Hillocks et al., Reference Hillocks, Bennett and Mponda2012).
Nutritional composition of Bambara groundnut
BGN has immense nutritional potential and, hence, is included as a valuable crop by the African Orphan Crop Consortium. It has nutrients in adequate quantities to provide a balanced diet (Maphosa, Reference Maphosa2016). BGN has a high nutritional value with carbohydrates, proteins, dietary fibre and fat in the range 55–70%, 17–25%, 5.2–6.4% and 1.4–12%, respectively. The nutritional composition of BGN is given in Table 2.
Table 2. Proximate nutritional composition of Bambara groundnut seeds
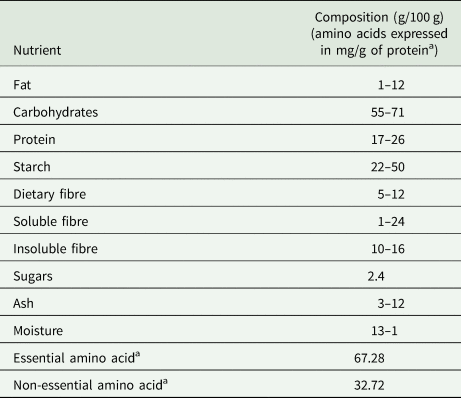
a Essential and non-essential amino acids expressed in mg/g of protein.
Adapted from: Murevanhema and Jideani (Reference Murevanhema and Jideani2013); Olaleye et al. (Reference Olaleye, Adeyeye and Adesina2013); Maphosa (Reference Maphosa2016); Yao et al. (Reference Yao, Kouassi, Erba, Scazzina, Pellegrini and Casiraghi2015); Khan et al. (Reference Khan, Alam, Ali, Bibi and Khalil2007); Majola et al. (Reference Majola, Gerrano and Shimelis2021).
BGN has a comparable protein (20.8%) and carbohydrate (61.9%) content to more commercialized legumes such as cowpea, kidney beans, broad bean and chickpea, but it has a lower protein and fat content than soybeans as shown in Fig. 3 (Gulu, Reference Gulu2018). Of the legumes reported in Fig. 3, soybean has the highest protein (36.5%) and fat (19.9%) as well as the lowest carbohydrate content (30.2%). BGN protein is rich in the essential amino acids, methionine (3%) and lysine (2%) (Murevanhema and Jideani, Reference Murevanhema and Jideani2013). It has a higher leucine, isoleucine, lysine, methionine, phenylalanine, threonine and valine composition (Table 3) than groundnut, one of the most consumed legumes (Olaleye et al., Reference Olaleye, Adeyeye and Adesina2013). The concentration of lysine makes it suitable for complementing cereals such as wheat and rice, which have a low lysine content (Murevanhema and Jideani, Reference Murevanhema and Jideani2013). Furthermore, the high protein content of BGN (17–25%) makes it an excellent source of proteins for those who do not consume animal protein as well as lower socioeconomic class individuals who cannot afford meat-derived protein (Guillon and Champ, Reference Guillon and Champ2002; Massawe et al., Reference Massawe, Mwale, Azam-Ali and Roberts2005; Bamshaiye et al., Reference Bamshaiye, Adegbola and Bamshaiye2011).
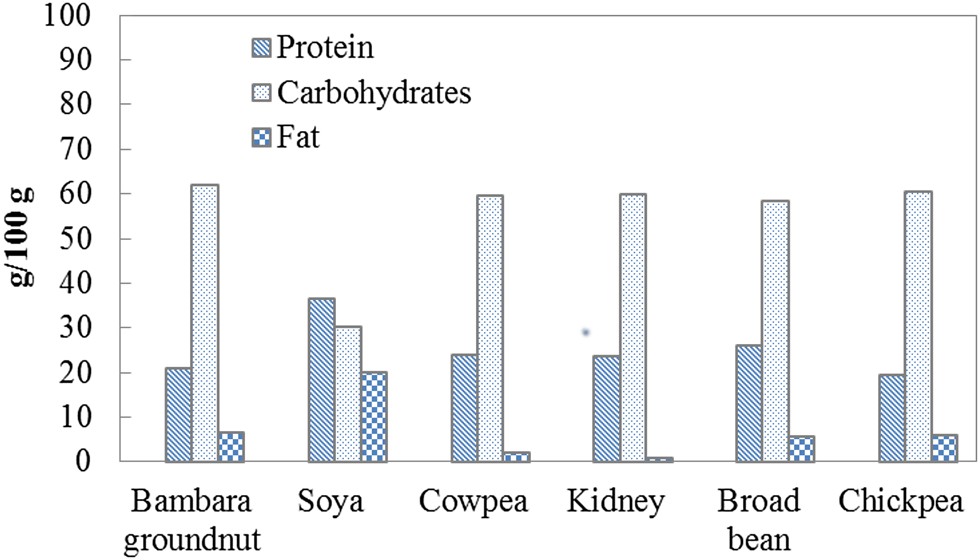
Fig. 3. Colour online. Protein, carbohydrate and fat composition of various legumes (Gulu, Reference Gulu2018).
Table 3. Amino acid composition of Bambara groundnut seeds
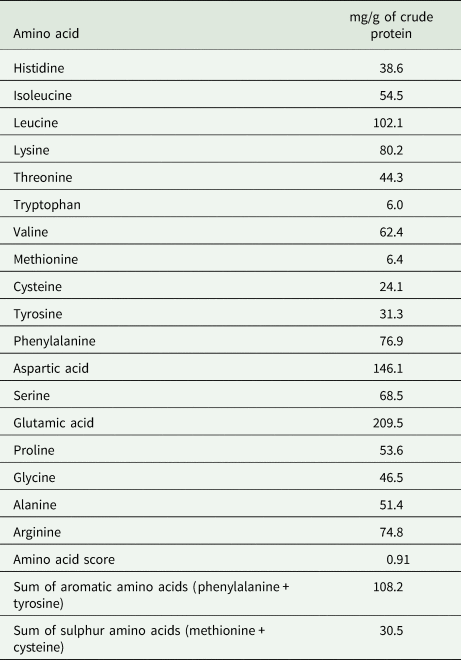
Source: Olaleye et al. (Reference Olaleye, Adeyeye and Adesina2013).
BGN also contains minerals such as potassium (1545–2200 mg/100 g), magnesium (32–335 mg/100 g), calcium (30–128 mg/100 g), iron (2–9 mg/100 g) and phosphorus (81–563 mg/100 g) (Table 4). The recommended dietary allowance for adults are also presented in Table 4.
Table 4. Mineral composition of Bambara groundnut and corresponding recommended daily allowances for adults
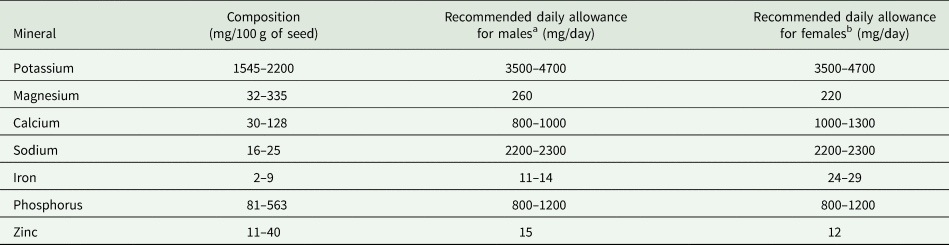
a Males (weight = 65 kg, age = 19–65 years).
b Females (weight = 55 kg, age = 19–65 years).
Adapted from: Majola et al. (Reference Majola, Gerrano and Shimelis2021); Maphosa (Reference Maphosa2016); Yao et al. (Reference Yao, Kouassi, Erba, Scazzina, Pellegrini and Casiraghi2015); Murevanhema and Jideani (Reference Murevanhema and Jideani2013); Olaleye et al. (Reference Olaleye, Adeyeye and Adesina2013); Khan et al. (Reference Khan, Alam, Ali, Bibi and Khalil2007); FAO (2001).
The BGN fat is naturally cholesterol-free and low in saturated fatty acids (Shanmugasundaram, Reference Shanmugasundaram2003) making it healthier than animal protein. The fatty acid profile of BGN makes its inclusion in diets important for aiding weight loss, reducing the risk of heart-related disease and lowering cholesterol levels. Table 5 shows the fatty acid composition of BGN. The main fatty acids in BGN are oleic (18:1 n-9), linoleic (18:2 n-6) and palmitic (16:0) representing 23, 36 and 21%, respectively (Yao et al., Reference Yao, Kouassi, Erba, Scazzina, Pellegrini and Casiraghi2015). BGN has both the omega 3-(n-3) and omega 6-(n-6) fatty acids among other polyunsaturated fatty acids (PUFA) (Okafor et al., Reference Okafor, Jideani, Meyer and Le Roes-Hill2022) suggesting that its consumption would promote health benefits associated with these PUFA.
Table 5. Fatty acid composition of lipid extract from Bambara groundnut seeds
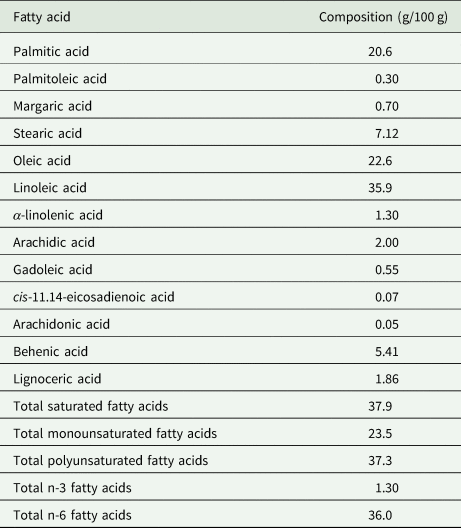
Source: Minka and Bruneteau (Reference Minka and Bruneteau2000); Olaleye et al. (Reference Olaleye, Adeyeye and Adesina2013); Yao et al. (Reference Yao, Kouassi, Erba, Scazzina, Pellegrini and Casiraghi2015).
BGN has a high concentration of soluble dietary fibre (15–17%) (Maphosa, Reference Maphosa2016). This content is higher than that of common legumes such as pea, broad pea and soybean which ranges between 3.3 and 13.8% (Guillon and Champ, Reference Guillon and Champ2002; Khan et al., Reference Khan, Alam, Ali, Bibi and Khalil2007). This further accentuates its nutritional quality, as soluble dietary fibre plays a major physiological role in reducing constipation, obesity, diabetes, heart complications, piles and some cancers (Khan et al., Reference Khan, Alam, Ali, Bibi and Khalil2007). Furthermore, soluble dietary fibre plays a role in lowering blood cholesterol, improving glucose tolerance and reducing glycaemic response by reducing glucose and cholesterol assimilation into the bloodstream (Khan et al., Reference Khan, Alam, Ali, Bibi and Khalil2007). Table 6 shows the non-starch polysaccharide composition of BGN.
Table 6. Non-starch polysaccharide composition of Bambara groundnut seeds
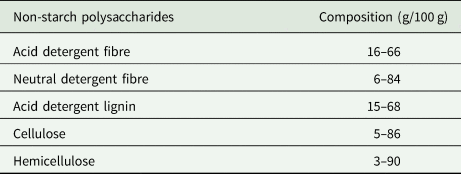
Source: Olaleye et al. (Reference Olaleye, Adeyeye and Adesina2013).
Table 7 shows the carotenoids, tocopherols, polyphenols and antioxidant capacity of BGN. The total polyphenolic content and ferric reducing antioxidant power of BGN are 706.9 mg CE/100 g and 0.62 mmol of Fe2+/100 g, respectively (Olaleye et al., Reference Olaleye, Adeyeye and Adesina2013). Antioxidant compounds have the capacity to react with free radicals forming stable or non-reactive radicals thereby reducing inflammation in the human body, as well as providing anti-cancer and anti-ageing benefits (Maphosa and Jideani, Reference Maphosa and Jideani2016).
Table 7. Carotenoids, tocopherols, total polyphenols and total antioxidant capacity of Bambara groundnut seeds
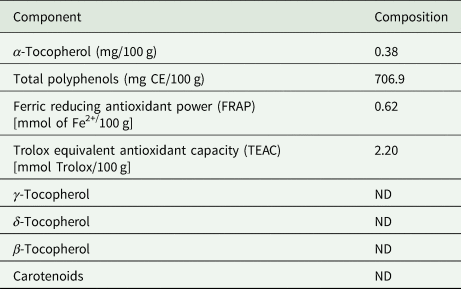
CE, catechin equivalents; ND, not detected.
Source: Olaleye et al. (Reference Olaleye, Adeyeye and Adesina2013); Yao et al. (Reference Yao, Kouassi, Erba, Scazzina, Pellegrini and Casiraghi2015).
The presence of antinutrients, such as condensed tannins, interferes with the digestion and bioavailability of nutrients. Condensed tannins are polyphenolic compounds present in many legumes and range from 0.01 to 2.37 mg/m in BGN (Harris et al., Reference Harris, Jideani and Le Roes-Hill2018; Okafor et al., Reference Okafor, Jideani, Meyer and Le Roes-Hill2022). They inhibit protein digestion by inhibiting the proteolytic activity and forming indigestible complexes with protein, consequentially reducing the bioavailability of amino acids. Trypsin inhibitors inhibit the activity of digestive enzymes. Processing and pre-processing methods such as cooking, roasting, germination and soaking significantly reduce the tannin content in BGN seeds (Olaleye et al., Reference Olaleye, Adeyeye and Adesina2013; Yao et al., Reference Yao, Kouassi, Erba, Scazzina, Pellegrini and Casiraghi2015).
Uses of Bambara groundnut
BGN is mainly cultivated for food purposes (Table 8) and is consumed mostly at household level with little or no trading taking place. Apart from food applications, some cultures believe that BGN has medicinal properties and can be used in traditional ceremonies such as funerals, weddings, rituals and gift exchanges (Gerrano et al., Reference Gerrano, Jansen van Rensburg and Adebola2013). The fresh or dry seeds are boiled, fried, steamed or roasted and consumed on their own or are incorporated in other dishes such as soups, gravies and relish (Table 8). A probiotic beverage and yoghurt from BGN were found to have potential as alternatives for lactose-free milk in the market (Murevanhema and Jideani, Reference Murevanhema and Jideani2013). The probiotic beverage was characterized by a high phenolic and flavonoid content suggesting the presence of antioxidant properties (Jideani and Murevanhema, Reference Jideani and Murevanhema2013). Sensory evaluation indicated that the probiotic beverage and yoghurt would be widely accepted in the market by all age groups. Notable is the work of Jideani and Murevanhema (Reference Jideani and Murevanhema2013) who patented a process to produce a prebiotic beverage from BGN flour.
Table 8. Various food uses of Bambara groundnut
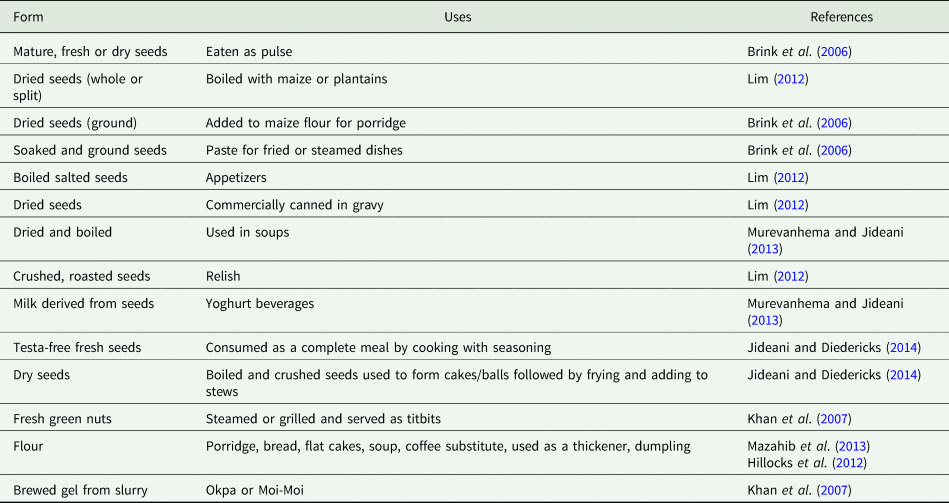
The addition of boiled BGN to fermented maize dough increased its protein content from 10 to 16.4% (Hillocks et al., Reference Hillocks, Bennett and Mponda2012). BGN flour can be utilized by the cereal and baking industry as a wheat replacement in biscuit and flat cakes (Hillocks et al., Reference Hillocks, Bennett and Mponda2012). Insoluble fibres from BGN black-eye, brown-eye, red and brown varieties significantly increased the dietary fibre content of white bread, lowered the specific loaf volume and had positive textural effects especially on crumb softness (Diedericks, Reference Diedericks2014). As such, BGN insoluble fibres would make good ingredients and fortifying agents in the baking industry. Jideani and Diedericks (Reference Jideani VA, Diedericks and Oguntibeju2014) patented the extraction of dietary fibre from BGN seeds using the enzymatic-gravimetric method. The effect of BGN flour on the stability and rheology of oil-in-water emulsions was studied (Adeyi, Reference Adeyi2014) and was shown to positively influence the stability as well as the time-dependent, time-independent and oscillatory characteristics of the studied oil-in-water emulsions. BGN flour is a better emulsifier than BGN starch as it significantly reduces the migration rate of oil droplets and reduces oil droplet size resulting in higher stability (Gabriel et al., Reference Gabriel, Jideani and Ikhu-Omoregbe2013). This is because BGN flour has proteins and dietary fibre, in addition to the starch, which contributes to its emulsifying and stabilizing power (than groundnut, one of the most consumed legumes) (Olaleye et al., Reference Olaleye, Adeyeye and Adesina2013; Maphosa, Reference Maphosa2016).
Maphosa and Jideani (Reference Maphosa and Jideani2016) extracted and studied BGN-soluble and insoluble dietary fibre, reporting many desirable physicochemical properties of both. Soluble dietary fibre proved to be a good orange oil beverage emulsion stabilizer. Maphosa et al. (Reference Maphosa, Jideani and Ikhu-Omoregbe2022) produced a nanocomposite from BGN starch and soluble dietary fibre and used it to stabilize beverage emulsions at significantly lower concentrations than BGN-soluble dietary fibre.
However, despite these potential and diverse uses and benefits, BGN is often neglected as a consumable legume due to its hard-to-cook phenomenon and the lack of sufficient knowledge on ways of utilizing the seeds (Murevanhema and Jideani, Reference Murevanhema and Jideani2013). Fresh BGN seeds are preferred to dry seeds by a majority of consumers because they do not require large amounts of time and energy to cook (Mubaiwa et al., Reference Mubaiwa, Fogliano, Chidewe and Linnemann2016).
Production constraints
The average growth period of BGN is 110–150 days (Basu et al., Reference Basu, Mithen and Pasquet2007). During the growth period, a range of production constraints limit production, but there is scope to use modern approaches to improve and overcome these production barriers. Biotic stresses, that is, diseases caused by fungi, bacteria and viruses; insect pests; and nematodes, affect productivity as well as overall yield and quality (Majola et al., Reference Majola, Gerrano and Shimelis2021). However, some of the diseases can be controlled using crop protection chemicals and good agronomic management practices such as crop rotation, burning of previous season's debris and the application of ash on storage seeds. The diseases commonly identified in BGN were extensively reviewed by Majola et al. (Reference Majola, Gerrano and Shimelis2021) and they are mostly caused by fungi, viruses, insects and nematodes. Fungal diseases of BGN include leaf spot (Cercospora canescens) (Ellis and Martin), leaf blight (Colletotrichum graminicola [Ces.] G.W. Wils), Fusarium wilt (Fusarium oxysporum Schlechtend f.sp. voandzeia), and rust (Puccinia graminis f.sp. Tritici) and dieback disease (Lasiodiplodia theobromae [Pat.] Griff. & Maubl). Phomopsis sp., Aspergillus genera and Sclerotium rolfsii (Sacc.) also cause diseases in BGN. The common viral diseases are cowpea aphid-borne mosaic virus, black-eye cowpea mosaic virus, peanut mottle potyvirus, cowpea mottle comovirus, cowpea yellow mosaic virus and Rosette virus disease. The pests that affect BGN include root-knot nematode [Meloidogynae javanica Treub], leafhoppers [Hilda patruelis (Stal) and Empoasca facialis (Jacobi), Aphids, groundnut jassid (Empoasca kerri Pruthi)] as well as brown leaf beetles (Ootheca mutabilis Schönherr). After harvest the postharvest storage insect pests often encountered are cowpea weevil (Callosobruchus maculatus F.) and Bruchids (Callosobruchus maculatus Boh.). Diseases can affect seed germination, physiological processes, crop growth, seed quality and may result in production of mycotoxin which is harmful to both humans and livestock when the grain or straw is consumed.
Major abiotic constraints to BGN production include erratic rainfall, temperature extremes (very high and low), altitude and poor soils (Gerrano et al., Reference Gerrano, Jansen van Rensburg and Adebola2013). In addition to potentially shifting seasonal conditions, climate change can also alter the epidemics and threats of plant pests and diseases (West et al., Reference West, Townsend and Stevens2012). The effects of predicted climatic changes are expected to be dire and might shift the rainfall season causing a disruption to the planting calendar of subsistence farmers who are reliant on rainfall patterns (Aliyu et al., Reference Aliyu, Massawe and Mayes2016; Pasipanodya et al., Reference Pasipanodya, Horn, Achigan-Dako, Musango and Sibiya2022).
Furthermore, most subsistence farmers are farming on poor and depleted soils in marginal lands. Stresses can occur simultaneously or have contrasting effects, for example, heat and drought stress (or salinity and drought) tend to occur co-concurrently and can be under similar or same genetic control mechanisms (Tricker et al., Reference Tricker, ElHabti, Schmidt and Fleury2018). BGN is largely considered to be a drought resilient crop (Mayes et al., Reference Mayes, Ho, Chai, Gao, Kundy, Mateva, Zahrulakmal, Hahiree, Kendabie, Licea, Massawe, Mabhaudhi, Modi, Berchie, Amoah, Faloye, Abberton, Olaniyi and Azam-Ali2019), though drought affects a range of its traits and physiological processes (Table 9).
Table 9. Bambara groundnut traits and physiological processes affected by drought
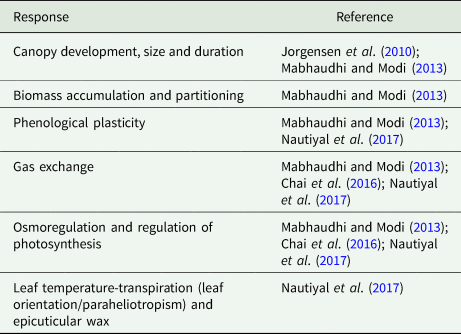
It requires an average rainfall of about 600–700 mm during the growing season to maximize yield, though later research has estimated the minimum rainfall requirement of the crop to be around 300 mm (Majola et al., Reference Majola, Gerrano and Shimelis2021). Plant response to drought involves avoidance, escape or tolerance (Mayes et al., Reference Mayes, Ho, Chai, Gao, Kundy, Mateva, Zahrulakmal, Hahiree, Kendabie, Licea, Massawe, Mabhaudhi, Modi, Berchie, Amoah, Faloye, Abberton, Olaniyi and Azam-Ali2019) and all the three mechanisms have been observed in BGN to varying levels (Jorgensen et al., Reference Jorgensen, Liu, Oue´draogo, Ntundu, Sarrazin and Christiansen2010; Mabhaudhi and Modi, Reference Mabhaudhi and Modi2013; Chai et al., Reference Chai, Aliyu, Massawe and Mayes2016). Excessive rainfall especially during maturity and/or harvest potentially causes waterlogging and affects the underground grain causing yield losses. To avoid water logging, BGN crops are planted on moulds or ridges (Gulu, Reference Gulu2018).
It is frost sensitive at any growth stage and warm temperatures accelerate plant development, with 19–30°C being ideal for optimal development (Masindeni, Reference Masindeni2006). Temperatures above 30°C cause heat stress and limit yield (Majola et al., Reference Majola, Gerrano and Shimelis2021). Extreme temperatures (high or low) cause dying or wilting of the leaves, and reduction of biomass accumulation and ultimately yield. Fruits (grains) are below ground and thus a properly aeriated, well-drained, loose sandy loam soil is preferable as it does not damage the seeds. It is also susceptible to anoxic/hypoxic stress factors (sensitivity to flooding) (Sesay et al., Reference Sesay, Mpuisang, Morake, Al-Shareef, Chepete and Moseki2013), and thrives best in soils with a pH between 5 and 6.5.
Production improvement approaches
Co-ordinated and cross-disciplinary approaches are needed in crop improvement for BGN. These would facilitate proper and detailed environmental characterization, adoption of modern phenotyping technologies in both the field and laboratories to complement breeding approaches. Within breeding programmes, the role of landraces and wild relatives in providing genetically diverse material and potential genes of interest should be incorporated.
Environmental characterization
In controlled environments, data capture is easier compared to under field conditions. However, crops behave differently in pot experiments under controlled glasshouse conditions compared to the field where they exist as a community, and the results from controlled conditions are not easily extrapolatable to the field. Lack of detailed and accurate environmental data especially in the field can hinder production and is therefore an area that needs improvement. This includes proper soil characterization especially the rhizosphere microorganism community, accurate recording of temperature and rainfall, exact geographic location of field, weed and disease control measures, sowing time, intercropping and rotations. In drought studies, for example, proper environmental characterization, beyond recording irrigation applied or rainfall received, is important as soil moisture and/or soil holding capacity is likely to differ between environments. The concept of ‘envirotyping’ has been proposed as a third ‘typing’ technology, complementing genotyping and phenotyping (Xu, Reference Xu2016). The approach encourages precise dissection of the environment through zooming into specific field plots and individual plants, as opposed to wholesome measurement of field environmental data wherein field variation would always exist (Xu, Reference Xu2016). This concept can potentially be key in improving BGN productivity and quality.
Modern phenotyping technologies
The high throughput phenotyping (HTP) technology is continuously being employed extensively in agriculture and plant science research, and excellent research and reviews have discussed this concept in greater detail (White et al., Reference White, Andrade-Sanchez, Gore, Bronson, Coffelt, Conley, Feldmann, French, Heun, Hunsaker, Jenks, Kimball, Roth, Strand, Thorp, Wall and Wang2012; Araus and Cairns, Reference Araus and Cairns2014; Wang et al., Reference Wang, Tian, Yao, Zhu and Cao2014; Zhou et al., Reference Zhou, Zheng, Xu, He, Ge, Yao, Cheng, Zhu, Cao and Tian2017; Sankaran et al., Reference Sankaran, Jianfeng Zhou, Khot, Trapp and Miklas2018; Maphosa et al., Reference Maphosa, Richards, Norton and Nguyen2020) and is a promising tool for improving BGN production. In BGN, the approach has been adopted, with normalized difference vegetation index, enhanced vegetation index 2, green normalized difference vegetation index and simple ratio generated from the red-green near infrared bands shown to be correlated to grain yield in BGN using a high throughput and low-cost UAV-based remote-sensing technology (Jewan et al., Reference Jewan, Pagay, Billa, Tyerman, Deepak Gautam, Debbie Sparkes, Hui Hui Chai and Singh2021). A detailed but non-exhaustive list of HTP technologies applicable to legumes was given by Maphosa et al. (Reference Maphosa, Richards, Norton and Nguyen2020) and thus would not be repeated in this manuscript. The approach involves application of sensor or image-based tools, to non-destructively and non-evasively measure crop traits across time and space. The screening tools can be handheld (Tracy et al., Reference Tracy, Nagel, Postma, Fassbender, Wasson and Watt2020), airborne (Bian et al., Reference Bian, Zhang, Chen, Chen, Cui, Li, Chen and Fu2019), ground-based vehicles (Maphosa et al., Reference Maphosa, Thoday-Kennedy, Vakani, Phelan and Badenhorst2017), thermal and hyperspectral imagery or shovelomics (Chen et al., Reference Chen, Ghanem and Siddique2017). Key production traits that can be captured include phenology, early vigour, crop growth status, water content, biomass, yield potential and grain quality (colour and size). Common approaches to measuring and/or recording these important traits are manual, laborious and time consuming, for example, biomass cuts. Also, recording phenology is largely based on subjective scales that can introduce bias especially if more than one person is involved in the scoring. With these commonly used approaches there is a limit on the number of genotypes that can be accurately screened thus necessitating adoption of rapid HTP ones.
Improved breeding
The focus of breeding programmes is to improve grain yield and nutritional quality. The BGN genome has not been fully explored, compared to other high value crops, and this presents opportunities to further explore the available germplasm and genetic variation for breeding and cultivar development (Majola et al., Reference Majola, Gerrano and Shimelis2021). Limited controlled breeding programmes for BGN are hampering research, improvement and release of advanced varieties. There are few known cultivars, with production still heavily reliant on landraces, and the breeding approach is not clearly defined leading to limited germplasm development progress. Research can benefit from related studies in model species through comparative genetic approaches (Aliyu et al., Reference Aliyu, Massawe and Mayes2014) as there often tends to be strong conserved synteny across related crop species (King et al., Reference King, Armstead, Harper, Ramsey, Snape, Waugh and King2013). Like most underutilized crops, BGN's pedigree structure can be defined as ‘flat genetic structure’ since it is composed of a mixture of genotypes with disconnected non-hierarchical populations (Aliyu et al., Reference Aliyu, Massawe and Mayes2014). Costs, though decreasing, remain a limitation for breeding underutilized species as they are seldom funded.
The available diversity at both phenotypic and genotypic levels is sufficient to support breeding improvement programmes. Characterization, at molecular and phenotypic level of available landraces (Masindeni, Reference Masindeni2006; Unigwe et al., Reference Unigwe, Gerrano, Adebola and Pillay2016), can form the foundation and/or theoretical framework for improvement through identifying potential favourable parents. For promising genotypes, the original source can be tracked back and be searched for additional favourable traits (Aliyu et al., Reference Aliyu, Massawe and Mayes2014). The challenge with landraces, however, is that they have high heterozygosity as they often are a mixture of genotypes which complicates understanding the underlying genetic control mechanisms and overall genotype-by-environment (G × E) interactions. G × E interactions play a huge role in crop productivity (Richards et al., Reference Richards, Preston, Napier, Jenkins and Maphosa2020), and together with management can result in quantum leaps in yield, and closure of the yield gap. The environmental influence in G × E interactions reduces heritability, thus complicating the selection process. To advance breeding and achieve homozygosity, measures must be in place to create pure lines through single seed descent. From this seed a characterizable genotype can be developed and tested in multi environment trials.
Within a breeding programme, a range of statistical methodologies that can test genotype stability or environmental sensitivity such as GGE biplot analysis can be effectively used in selecting potential genotypes (Malosetti et al., Reference Malosetti, Ribaut and van Eeuwijk2020). Sound agronomic genomics, an integration of agronomy with the available ‘omics’ to optimize gene expression (Xu, Reference Xu2016), is another approach with potential to breed for enhanced crop varieties. These enable identification of adapted and environmentally stable genotypes. However, to some limited extent, BGN improvement has been aided by adopting modern genetics and genomic approaches to complement conventional breeding in the selection of favourable traits (Basu et al., Reference Basu, Mithen and Pasquet2007). This would be further enhanced by the availability of the completed genome sequence. Like other crops with significant nutritional and cultural importance, BGN genetic resources (6145 accessions) are held in gene banks worldwide, with IITA-GRC – Nigeria holding the largest (2031) number collected from 25 different countries (Muhammad et al., Reference Muhammad, Rafii, Ramlee, Nazli, Harun, Oladosu, Musa, Arolu, Chukwu, Haliru, Akos, Halidu J and Arolu2020; Pasipanodya et al., Reference Pasipanodya, Horn, Achigan-Dako, Musango and Sibiya2022). A suite of breeding approaches were widely discussed by Maphosa et al. (Reference Maphosa, Richards, Norton and Nguyen2020) and references therein such as conventional, molecular and genomic assisted breeding, physiological, shuttle and speed breeding and these can be employed in improving BGN.
Breeding programmes have adopted several marker types as amplified fragment length polymorphism (AFLP), restriction fragment length polymorphism, simple sequence repeats (SSRs), inter sequence repeats, random amplified polymorphic DNA and genotyping by sequencing to genotype BGN (Aliyu et al., Reference Aliyu, Massawe and Mayes2016). Furthermore, as reviewed extensively by Pasipanodya et al. (Reference Pasipanodya, Horn, Achigan-Dako, Musango and Sibiya2022) linkage mapping using bi-parental populations has been used to study marker trait association and identification of useful QTLs. A marker (bgPabg-596774) associated with number of pods, number of nodes, pod weight, number of seed, seed weight and biomass dry weight traits has been identified in BGN (Ahmad et al., Reference Ahmad, Basu, Redjeki, Murchie, Massawe, Azam-Ali, Kilian and Mayes2013). However, the availability of the draft reference genome of BGN (National Center for Biotechnology Information (NCBI) database) offers further opportunities to identify genetic regions associated with traits of interest and accelerate genetic gains. The draft genome is 535.05 Mb in size from which 31 707 protein-coding genes are predicted (Chang et al., Reference Chang, Liu, Liu, Liao, Sahu, Fu, Song, Cheng, Kariba, Muthemba, Hendre, Mayes, Ho, Yssel, Kendabie, Wang, Li, Muchugi, Jamnadass, Lu, Peng, Deynze, Simons, Yana-Shapiro, Van de Peer, Xu, Yang, Wang and Liu2019). Also, environment association analysis which uses georeferenced genetic materials to model associations between genetic sequences and environmental attributes can be adopted to identify candidate genes for environmental adaptation (Rellstab et al., Reference Rellstab, Gugerli, Eckert, Hancock and Holderegger2015).
Conclusion and prospects
Concerted improvement of grain yield and nutritional value will increase productivity and adoption of BGN by farmers. This however must be underpinned by improvements in crop management practices, value chains and market access, as well as overall sustainable collaboration across disciplines. At farm level, this would involve the use of improved and well-characterized varieties, adoption of modern phenotyping technologies, following sound agronomic advice and management practices as well as detailed characterization of the field. Despite having nutritional advantages over other commercial legumes, BGN has not developed as a traded commodity. This could be due to insufficient demand in the formal market resulting from lack of marketing and promotion. Empirical evidence and various research outcomes support the potential of BGN both as a crop and as food. BGN deserves more publicity and increased utilization than it is currently receiving. It is a low budget, low cost, reliable crop that thrives in adverse conditions, considered too harsh for other crops. The public, especially the lower income groups, need to be educated on the nutritional value of BGN. For example, the production of a probiotic beverage from BGN flour proved to be energy saving and, thus, would result in an affordable product in the marketplace. BGN has potential to be used in different branches of the food industry. Efforts should be intensified to improve the utilization of BGN in Africa and the rest of the world. There is a considerable need for new and innovative uses of BGN. The challenge is to narrow the gap and improve food security at household level. To attract the attention of consumers, innovative ways of presenting BGN as a food or food ingredient are required. Innovation may include the production of snacks from BGN, marketing BGN as a prebiotic and extracting the constituents of BGN such as dietary fibre for inclusion in other food applications.
Author contributions
Yvonne Maphosa: substantially contributed to the conception and design of the article and interpreting the relevant literature; wrote the article. Victoria A. Jideani: supervised the writing process; critically revised the article for important intellectual content. Lancelot Maphosa: substantially contributed to the conception and design of the article and interpreting the relevant literature; wrote the article.
Financial support
This research received no specific grant from any funding agency, commercial or not-for-profit sectors.
Conflict of interest
None.
Ethical standards
Not applicable.