INTRODUCTION
Murine typhus is a flea-borne rickettsial disease associated mainly with commensal rodents and is caused by the bacterium Rickettsia typhi [Reference Eisen and Gage1]. Humans are incidental hosts, where the pathogen causes a non-specific febrile illness often with fever, headache, arthralgia and rash [Reference Civen and Ngo2]. Murine typhus is one of the most widely distributed arthropod-borne infections throughout the world, yet it is frequently unrecognized or misdiagnosed due to its non-specific and usually mild clinical presentation [Reference Raoult and Roux3]. Despite the fact that murine typhus can cause severe illness and death [Reference Azad4, Reference Dumler, Taylor and Walker5], clinical signs and symptoms in untreated (undiagnosed) patients usually last for 7–14 days followed by spontaneous resolution of the illness [Reference Gray6, Reference Parola and Raoult7]. Hence, its incidence is likely to be under-reported.
Of the rickettsiae only R. typhi and Rickettsia felis, belonging to the typhus and transitional groups, respectively, have been reported to occur in New Zealand [Reference Eisen and Gage1, Reference Derne8]. R. typhi is primarily transmitted by the oriental rat flea, Xenopsylla cheopis, and humans become infected through the inhalation of R. typhi-containing flea faeces, or via contact through disrupted skin [Reference Raoult and Roux3]. R. typhi is maintained by the classic rat–rat flea cycle, where Rattus rats are the main reservoir hosts that can be infected by the inoculation of infected flea faeces into the bite wound [Reference Gillespie9], or the regurgitation of rickettsiae by the infected flea during blood feeding [Reference Azad and Traub10]. Studies within the United States have reported antibodies against R. typhi in opossums and domestic cats and dogs [Reference Adjemian11] and a shift in the life-cycle of R. typhi from the classic rat–rat flea cycle to opossum–cat flea cycle [Reference Civen and Ngo2, Reference Boostrom12]. In comparison, the disease ecology of R. felis is less clear [Reference Eisen and Gage1]. However, R. felis DNA is most highly reported in cat fleas, Ctenocephalides felis, worldwide [Reference Perez-Osorio13].
Up to the end of 2014, 72 locally acquired murine typhus cases had been recorded in New Zealand ([Reference Lim14]; Institute of Environmental Science and Research, written personal communication). For R. felis, cat fleas hosted by cats and dogs have been found to carry the bacterium in New Zealand [Reference Kelly, Rolain and Raoult15] and evidence of human R. felis infections have recently been reported [Reference Lim14]. It is important to note that because R. felis rickettsiosis shares a similar clinical profile to murine typhus (and potentially similar vectors and reservoirs), infection can be mistaken for a suspected case of R. typhi [Reference Perez-Osorio13].
The first reported New Zealand-acquired murine typhus case occurred in greater Auckland in 1989 [Reference Ellis-Pegler, Cooper and Croxson16], where it has continued to occur at low-incidence levels. Since then cases have also been reported up to 130 km away to the south in the Waikato region (Fig. 1) [Reference Gray6, Reference Irwin, Tredoux and Mills17]. A marked increase in cases reported in this region from 2006 to 2008 raised concerns with health authorities that the disease may be continuing to both increase in prevalence and spread further south, i.e. is an emerging disease. In response, we conducted the first seroprevalence study in New Zealand for R. typhi and R. felis, using indirect immunofluorescence, Western blot, and cross-adsorption assays of samples from blood donors. Our goal was to explore the presence of R. typhi in the Waikato region, covering beyond the southernmost known distribution.

Fig. 1. Northern part of North Island, New Zealand, highlighting the Waikato District Health Board study region and the location of the 12 Rickettsia typhi and six Rickettsia felis infections (including one dual infection).
METHODS
Clinical suspicion of rickettsial infections is most widely confirmed by serological tests with the indirect immunofluorescence assay (IFA) being the gold standard [Reference La Scola and Raoult18, Reference Aung19]. However, members of the Rickettsia genus are notorious for cross-reactivity in serological tests [Reference Raoult and Roux3]. For example, antibodies against R. felis in human sera have been reported to cross-react with R. typhi in IFA [Reference Perez-Arellano20]. For this reason, coupled with the fact that both R. typhi and R. felis rickettsioses share similar clinical manifestations, we established, in New Zealand, a specific laboratory methodology using Western blot (WB) and cross-adsorption assays to distinguish between R. typhi and R. felis infections [Reference Lim14]. These assays, in combination with IFA, have been shown to be reliable in serological differentiation among several rickettsioses [Reference Perez-Arellano20, Reference La Scola21].
From 2009 to 2010, serum samples were obtained from 989 blood donors visiting the New Zealand Blood Service in Hamilton City (Fig. 1). Of these, 950 were from the Waikato District Health Board region with 435 residing to the north and 515 to the south of the city limits. All donors visiting the collection centre, except those residing within Hamilton city, were invited to participate and were enrolled sequentially during the study period. Participants were provided with a project information sheet, gave written, informed consent, and completed a standardized questionnaire. The questionnaire covered risk factors such as overseas travel, animal contact, and place of residence/employment. No participants withdrew from the study. The Northern Y Regional Ethics Committee of New Zealand approved this study (no. NTY/08/09/085).
Although samples from Waikato were the focus of our study, all sera were tested to identify any range expansions in the known distribution of R. typhi. Samples were first screened for seropositivity by IFA using slides acetone-fixed with R. typhi antigen [Australian Rickettsial Reference Laboratory (ARRL), Geelong, VIC, Australia; henceforth referred to as ARRL slides]. Each serum, including positive and negative control sera (ARRL, Australia), was diluted in 2% skim milk in phosphate-buffered saline before being applied to the slides and incubated at 37 °C for 30 min in a humidified container. Slides were washed three times, incubated with fluorescein-conjugated anti-human IgG, IgM and IgA (ARRL, Australia) at 37 °C for 30 min, washed again and examined using fluorescence microscopy. The cut-off titre for seropositivity was 1:128 as recommended by the manufacturer. All samples that were positive at this dilution were then tested using a commercially available group-specific, but not species-specific, IFA kit (henceforth referred to as Focus slides) for IgG against Typhus group (TG) R. typhi and Spotted Fever group (SFG) R. rickettsii (Focus Diagnostics, USA). The Focus slides include members of the transitional group within the SFG for interpretation of testing results.
Endpoint titres were determined for all samples tested on the Focus slides. According to kit instructions, endpoint titres ⩾1:64 and <1:256 indicate either past infection or early response to a recent infection, and titres ⩾1:256 are considered presumptive evidence of recent or current infection. Hence, the cut-off titre for seropositivity on the Focus slides was 1:64. Because only R. typhi and R. felis have been reported as occurring in New Zealand, study sera that are TG-positive and SFG negative, and those that are SFG-positive and TG negative, are likely to represent R. typhi, and R. felis infections, respectively. Since R. typhi can cross-react with SFG rickettsiae [Reference Mouffok, Parola and Raoult22], and R. felis can cross-react both with other SFG rickettsiae and R. typhi [Reference Perez-Arellano20], those sera that are both TG- and SFG-positive may be due to either rickettsia. Positive reactivity may also represent overseas-acquired rickettsioses, or infections of other cross-reactive pathogens [Reference Kantso23]. Hence, WB and cross-adsorption assays using R. typhi (Wilmington) and R. felis (URRWXCal2) antigens (Unité des Rickettsies, France) were performed as described in Lim et al. [Reference Lim14] to confirm and differentiate between R. typhi and R. felis infections [Reference La Scola and Raoult18, Reference La Scola21].
RESULTS
Of the 989 sera, 106 (10·7%) tested positive on the ARRL slides. Of these 106 samples, 37 (3·7%, 37/989) showed seropositivity on the Focus slides to either the TG or SFG antigen, or to antigens of both groups (Fig. 2). Seven (0·7%, 7/989) were TG-positive and SFG-negative, nine (0·9%, 9/989) were SFG-positive and TG-negative, and 21 (2·1%, 21/989) cross-reacted with both TG and SFG antigens.
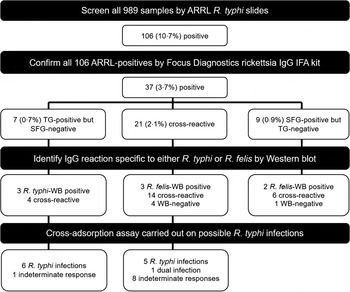
Fig. 2. Laboratory methodology for the identification and confirmation of Rickettsia typhi infections. Our combined approach of using indirect immunofluorescence assay (IFA), Western blot (WB) and cross-adsorption assay identified a total of 12 R. typhi infections, including one dual infection of both R. typhi and Rickettsia felis. ARRL, Australian Rickettsial Reference Laboratory; SFG, Spotted Fever group; TG, Typhus group.
WB was performed for all 37 samples that tested positive on the Focus slides. Five samples showed no specific WB reaction against either rickettsia (Fig. 2). Four of the five participants had not travelled overseas in the past 4 years, and thus could be infected with other IFA cross-reactive bacteria found in New Zealand. The participant with overseas travel could have been infected with rickettsiae other than R. typhi and R. felis, or other IFA cross-reactive bacteria. A rickettsial antigen was considered to represent the agent of infection when sera reacted only with the specific proteins of this antigen in the WB assay [Reference Perez-Arellano20]. Using this criterion, three were considered to be R. typhi infections, all of which were TG-positive and SFG-negative on the Focus slides (Fig. 2). Three TG- and SFG-positive samples and two SFG-positive but TG-negative samples reacted only against the R. felis WB, and thus were considered to be R. felis infections.
In identifying the final number of R. typhi infections, results from the IFA and WB assays eliminated the need for cross-adsorption assays to be performed on the five R. felis WB-positive samples, the five WB-negative samples, or the six WB cross-reactive SFG-positive samples (Fig. 2). Of the seven TG-positive but SFG-negative serum samples, six were confirmed to be R. typhi infections by the cross-adsorption assay. One participant was classified as having an indeterminate response and could have been infected with other bacteria that were known to cross-react with rickettsiae (such as Bartonella hensalae and Legionella bosmanii). Five other R. typhi infections were identified in samples that were cross-reactive on the Focus slides. One of the samples that showed cross-reactivity on the Focus slides was confirmed by the cross-adsorption assay to be a case of concomitant or consecutive infection by R. typhi and R. felis. The remaining eight samples produced indeterminate responses. When an R. typhi-infected serum sample was adsorbed with R. typhi, all homologous and heterologous antibodies were removed. After adsorption with R. felis, only homologous antibodies were removed, indicating that antibodies were specific for R. typhi. In contrast, when adsorption with R. typhi was performed on an R. felis-infected serum sample, antibodies against R. typhi were removed while antibodies specific to R. felis remained. Adsorption with R. felis resulted in the disappearance of all the antibodies, indicating R. felis rickettsiosis. In the case of dual infection, when the serum sample was adsorbed separately by R. typhi and R. felis, all homologous antibodies were removed in both adsorptions while antibodies against R. felis and R. typhi remained, respectively.
In summary, we identified 12 R. typhi and six R. felis infections (including one dual infection; Fig. 2). Endpoint titres, overseas travel, and animal contact for the 12 R. typhi infections are detailed in Table 1. Six of the 12 participants had no overseas travel in the past 4 years, with all participants listing contact with a range of risk-contributing animals [Reference Adjemian11]. All the R. typhi and R. felis infections were found in blood donors from the Waikato region (Fig. 1), giving a seroprevalence for R. typhi antibodies of 1·3% (12/950). Of these 12 R. typhi infections, six were in participants living north of Hamilton city (up to 100 km away) and six were found south of the city (up to 150 km away), indicating a seroprevalence for R. typhi antibodies of 1·4% (6/435) and 1·2% (6/515), respectively (no significant difference, χ 2 P = 0·768 at P < 0·05).
Table 1. Serological data and risk factors of participants that were confirmed to have Rickettsia typhi infection

IFA, Indirect immunofluorescence assay; TG, Typhus group; SFG, Spotted Fever group; WB, Western blot; Neg, negative.
* According to kit instructions, endpoint titres ⩾1:64 and <1:256 indicate either past infection or early response to a recent infection, and ⩾1:256 are considered presumptive evidence of recent or current infection.
DISCUSSION
Although IgG titres decline over time, detectable levels can remain for up to 3–4 years and thus exposure to R. typhi may have occurred at any time during this period [Reference Halle and Dasch24]. Since 6/12 R. typhi-infected participants, including the one with dual infection by R. typhi and R. felis, had travelled overseas in the past 4 years, it is possible that exposure could have occurred overseas. Other risk factors for murine typhus, that were common to all 12 participants, included animal contact such as rats, cats or dogs, or the presence of these animals in the home/work environment [Reference Adjemian11]. In the case of possums in New Zealand, there is as yet no clear link with the disease, unlike the involvement of opossums in the dynamics of murine typhus in the United States [Reference Civen and Ngo2, Reference Boostrom12]. Previous New Zealand studies have also identified rural lifestyle as a risk factor [Reference Gray6, Reference Irwin, Tredoux and Mills17, Reference Roberts25]. We cannot confirm this in our study as we did not enrol urban participants from Hamilton city against which to compare the rural samples.
While R. typhi seroprevalence was slightly higher to the north (1·4%, 6/435) than to the south (1·2%, 6/515) of Hamilton city, which lies near the centre of the study region (Fig. 1), it did not reach statistical significance. Since seroprevalence is similar in the north and south, it is likely that R. typhi is already established in southern Waikato, and endemic in the study region. Two participants with evidence of past R. typhi infections lived ~150 km due south of Hamilton city. These and two other participants south of Hamilton extend the southernmost range previously reported of human infections from R. typhi by up to 140 km [Reference Irwin, Tredoux and Mills17]. The two southernmost participants have lived in the same area for more than 7 years and have not travelled overseas in the past 4 years; hence the infection is likely to have been acquired locally after 2005 (given the limited length of time for detectable IgG levels).
Unlike many overseas countries, no large-scale seroprevalence studies have previously been conducted in New Zealand for rickettsioses. Compared to our study, investigations from Brazil and Greece have revealed similar seroprevalence levels for antibodies against R. typhi of 1·1% and 2%, respectively [Reference da Costa, Brigatte and Greco26, Reference Daniel27]; while studies from Colombia and Bosnia-Herzegovina have found seroprevalences as high as 25·2% and 37·7%, respectively [Reference Hidalgo28, Reference Punda-Polic, Leko-Grbic and Radulovic29]. However, caution should be exercised when comparing seroprevalence studies as factors such as study populations, serological methods, or diagnostic criteria for seropositivity may differ [Reference Aung19].
In our study in New Zealand, we trialled the use of WB and cross-adsorption for confirming IFA results and differentiating between R. typhi and R. felis infections. Although IFA is regarded as the gold standard for serological diagnosis of rickettsioses, cross-reacting antibodies within and among the rickettsial groups are not unusual [Reference Ormsbee30]. WB is considered to be the most specific serological assay for determining rickettsial infections and is especially useful for distinguishing true-positive from false-positive results obtained from IFA [Reference La Scola and Raoult18]. If we study the results based on the ARRL and Focus kit IFAs, we would conclude a higher prevalence for rickettsioses, i.e. being up to 10·7% and 3·7%, respectively (Fig. 2). However, using WB we were able to rule out five of the 37 Focus IFA-positive samples, as these five WB-negative samples were neither specific for R. typhi nor R. felis. The WB assay also eliminated the need for cross-adsorption to be performed on five identified R. felis WB-positive samples.
Cross-adsorption assays were used to identify the cross-reacting bacterium and the bacterium responsible for the infection when results from WB were not definitive. Our subsequent cross-adsorption assays allowed for species-specific diagnosis, resulting in nine further R. typhi infections being identified. However, we believe this technique is of limited applicability in New Zealand due to the large amount of antigen required and the relatively time-consuming methods, both resulting in a high cost per sample. Hence, we do not suggest the routine use of cross-adsorption assays to assist in confirmatory tests but WB may be useful for confirming IFA results and differentiating between true and false positives.
Our study also provided evidence of past R. felis infection in individuals living in New Zealand. All of the six R. felis-infected participants recorded risk factors associated with R. felis, having had contact with cats and dogs, the host reservoirs on which C. felis fleas have been found to carry R. felis in New Zealand [Reference Kelly, Rolain and Raoult15]. However, five out of these six participants also recorded overseas travel in the past 4 years, and since R. felis has a worldwide distribution [Reference Perez-Osorio13], there remains the possibility that these infections were acquired overseas. It is interesting to note that R. felis infections were found within 5–25 km of those for R. typhi (Fig. 1). The identification of R. felis infections is of public health concern because of the high prevalence of R. felis in C. felis fleas in New Zealand [Reference Kelly, Rolain and Raoult15] as well as the high rate of ownership of cats and dogs in the nation. Moreover, R. felis infections may be considerably under-reported in our study due to the ARRL slides being pre-treated with R. typhi antigen only, as was desired in answering our original study question. Globally, R. felis is an emerging disease of concern [Reference Perez-Osorio13] and where R. typhi and R. felis have been found co-circulating, R. felis can be more prevalent in fleas and hosts [Reference Boostrom12, Reference Abramowicz31, Reference Eremeeva32].
The overall findings from our study have important public health implications. We were able to demonstrate that R. typhi has a wider geographical endemicity than originally thought. As murine typhus is frequently misdiagnosed overseas and therefore substantially under-reported [Reference Raoult and Roux3], it is likely the disease is also under-diagnosed in New Zealand. In addition, while R. felis is not yet acknowledged as a public health issue in New Zealand, it may pose a significant risk. This issue mirrors the situation found overseas where R. felis is recognized as an emerging disease potentially overshadowing R. typhi in some regions [Reference Boostrom12, Reference Perez-Osorio13, Reference Abramowicz31, Reference Eremeeva32]. Infection from R. felis should be routinely considered when locally acquired murine typhus is suspected. Given that many of the routine diagnostic tests for rickettsioses are not species-specific, alternative laboratory methods that already exist may need to be utilized by public health laboratories. Furthermore, as the animal vectors and exposure route for R. typhi and R. felis can differ (predominantly rat fleas, X. cheopis; compared to cat and dog fleas, C. felis, respectively), targeted public health interventions (e.g. vector control) may need to be modified to match the associated disease. Thus, for public health interventions to be more effectively targeted in time and place, and thereby decrease the risk of rickettsioses emerging as a significant public health problem, it is recommended that whenever possible the rickettsial species involved in human infection should be identified.
ACKNOWLEDGEMENTS
We thank the New Zealand Blood Service, Hamilton, for coordinating the serum sample collection and all persons who participated in the study. We also thank the Unité des Rickettsies, Marseilles, France, and the Australian Rickettsial Reference Laboratory, Geelong, Victoria, for advice in the early stages of the project.
This work was supported by funding from the Institute of Environmental Science and Research, Porirua, New Zealand.
DECLARATION OF INTEREST
None.