Impact statement
One of the biggest problems we have today is water scarcity. Our current and future farm productivity and food and nutritional securities are heavily reliant on water. However, the primary obstacle to sustainable development nowadays is the growing shortage of water. Water shortage is a prevalent issue, even in nations with sufficient supplies of water (Mishra Reference Mishra2023). Climate change leading to global warming and other associated concerns is expected to result in rising temperatures and precipitation deficit making water scarcity worse in the near future (Alotaibi et al. Reference Alotaibi, Baig, Najim, Shah and Alamri2023). A growing number of severe and regular droughts are affecting agricultural productivity. In addition, the world’s population will continue to rise, as will their levels of living, and as dietary preferences and the consequences of climate change deepen, making this problem alarming. The agricultural industry is one of the leading sectors responsible for water shortage. Nearly 70% of water withdrawals come from farming, and in certain developing nations, it goes up to 95% (Ingrao et al. Reference Ingrao, Strippoli, Lagioia and Huisingh2023). As time goes on, we will need to use water resources more responsibly. If present water consumption trends continue, evidence points to the possibility that by 2025, two-thirds of the world’s population may reside in water-stressed nations (du Plessis Reference du Plessis2019). We must act now if we want to evidence a ‘ZeroHunger world by 2030’ set up by the United Nations. Hence, there is of pressing need to alleviate this scarcity by adopting measures, capturing and reusing our freshwater resources, using wastewater more safely and raising agricultural production in addition to increasing water efficiency. While taking these actions would not stop climate change and associated issues like global warming, drought and so on from happening, it can lessen the likelihood that will cause hunger and other socioeconomic problems and may provide viable solutions to prevent them.
Introduction
Human civilizations have always relied heavily on water for its growth and development. Nonetheless, the growth of agriculture and the economy in recent years has made the shortage of water severe and raised political and social unrest, underscoring the necessity of resource allocation and sustainable development. In the twenty-first century, almost 25% of the world’s population will have to deal with the issue of water scarcity (Alotaibi et al. Reference Alotaibi, Baig, Najim, Shah and Alamri2023; Seckler Reference Seckler, Barker and Amarasinghe1999). Water scarcity is largely caused by urbanization and internal migration which tend to concentrate in industrialized centers (Basu and Shaw Reference Basu, Shaw, Anwarul, Umma and Rajib2014; Oki and Quiocho Reference Oki and Quiocho2020). A study reports that 933 million (32.5%) of the world’s urban population resided in water-scarce regions in 2016, with 359 million (He et al. Reference He, Liu, Wu, Pan, Fang, Li and Bryan2021). More than 2.4 billion people have lived in water-scarce countries since 2000, and by 2025, that figure is predicted to increase to 4.8 billion (Ayt Ougougdal et al. Reference Ayt Ougougdal, Yacoubi, Messouli and Lachir2020). Water scarcity affects 40% of the world’s population and is on the rise; every day, 1,000 children pass away from diseases linked to poor sanitation and water quality (Aversa et al. Reference Aversa, Adamashvili, Fiore and Spada2022). Water scarcity has been assessed globally using a variety of indicators that have been created since the late 1980s when it was acknowledged as a global issue (Liu et al. Reference Liu, Liu, Yang, Ciais and Wada2022). The most widely used indicator of water scarcity is the ratio of water supply to demand, commonly known as blue water scarcity (BWS) (Cai et al. Reference Cai, Varis and Yin2017). Blue water is freshwater that can be used and can come from groundwater, lakes and streams. Truly, the world’s biggest problem nowadays is a lack of blue/freshwater (Mancosu et al. Reference Mancosu, Snyder, Kyriakakis and Spano2015). Future food consumption is anticipated to rise, which will have a direct impact on the amount of water used for agriculture (United Nations Department of Economic and Social Affairs, Population Division 2013). Furthermore, considerable water usage for irrigation is anticipated in the context of growing competition between agriculture and other economic sectors due to increased water shortages and drought brought on by climate change (IPCC Reference Field, Barros, Dokken, Mach, Mastrandrea, Bilir, Chatterjee, Ebi, Estrada, Genova, Girma, Kissel, Levy, MacCracken, Mastrandrea and White2014). Since the processes of climate change are accelerating amid an atmosphere of uncertainty and financial instability, sustainable development has emerged as a major worry for modern society (Minenna and Aversa Reference Minenna and Aversa2019). People are becoming more conscious of their impact on the environment and wildlife. Water and other basic materials should be used wisely, and this is becoming increasingly important.
In this paper, the authors portray a critical overview of water scarcity – its causes, impacts, present scenario, its relation to agriculture and possible adaptation strategies to mitigate the threat to sustainable development and agricultural food security globally and by emphasizing Indian perspectives. By offering useful information on the effects of water constraints in agriculture and adoption techniques, it is envisaged that this review will assist researchers and policymakers. Figure 1 showcases a pictorial overview of the various sources of water scarcity across the world and the remedial strategies through innovation and water-efficient agricultural practices and how they are linked with sustainability.
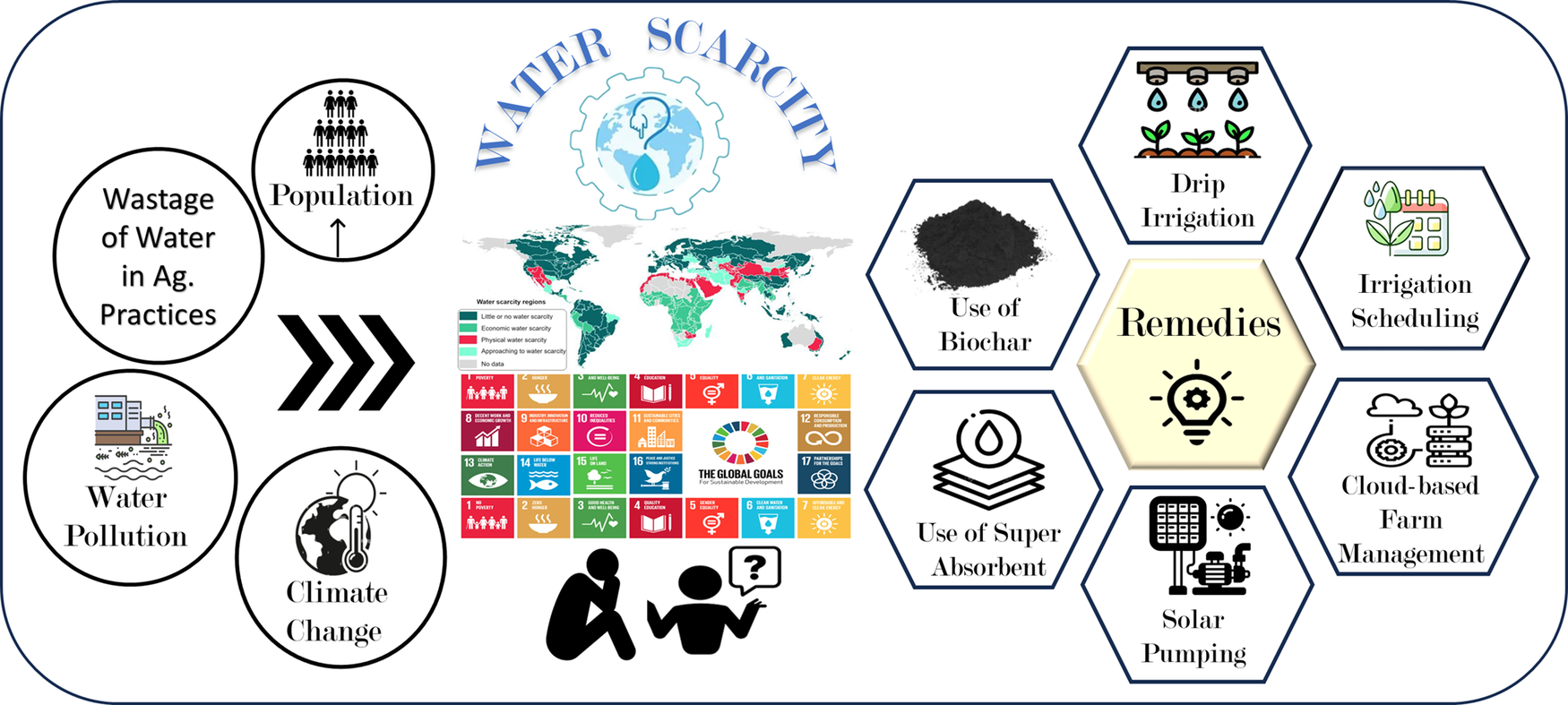
Figure 1. A pictorial overview of the various sources of water scarcity across the world and the remedial strategies through innovation and water-efficient agricultural practices and how they are linked with sustainable development goals.
Water: An important economic resource – Supply and demand
Water can be considered an important economic resource that is heavily utilized by households, industries and agricultural farms for a variety of uses every day, such as drinking purposes, irrigation, generation of hydroelectricity, recreation and amenities. The quantity and quality of the available water have a crucial impact on these goods and services. Water management and distribution include considering its unique qualities as a resource (Turner et al. Reference Turner, Georgiou, Clark, Brouwer and Burke2004). Water is a ‘bulky’ resource as it is abundantly available in nature. This indicates that it has a generally low economic worth per unit of weight or volume. As a result, unless a high marginal value can be produced, its transportation involves a high cost per unit of volume and is frequently not economically feasible across large distances. When compared to the little economic value achieved by using an additional unit of water, the expenses of abstraction, storage and any kind of transportation are often significant. This may result in the creation of location-specific water values.
Water also has additional characteristics in the sense that its supply cannot be easily predicted. It is governed by numerous processes, including water flow, surface evaporation and ground percolation. The interaction between groundwater systems and rivers/streams is crucial for maintaining ecological balance and water quality. Groundwater contributes to river flow, especially during dry periods, supporting aquatic habitats and ensuring a consistent water supply. This dynamic exchange regulates temperature, filters pollutants and sustains wetlands, essential for biodiversity (Sophocleous Reference Sophocleous2002; Winter et al. Reference Winter, Harvey, Franke and Alley1998). The supply of surface water is greatly influenced by weather variables and climate dynamics. As a result, the supply is unpredictable and inconsistent; 33% of the world’s internationally shared river basins mostly in the developing countries of Africa and South Asia will be in either high or very high water stress categories by 2025 due to climate change (du Plessis Reference du Plessis2019), industrial and agricultural growth as well a rise in population from 2.1 billion to 4.0 billion (expected) and the areas affected by ‘severe water stress’ will increase globally from 36.4 to 38.6 million km2 (Alcamo et al. Reference Alcamo, Henrichs and Rosch2000). At first glance, there appears to be plenty of room to increase supply through appropriate technical work if just 15% or less of the available water is being consumed though the distribution of resources is uneven in both distance and time, making it impossible to fully realize the potential (Allan Reference Allan1993). According to estimates, the rate at which groundwater storage is being depleted is between 100 and 200 km3/year, which translates to 15–25% of total groundwater withdrawals (United Nations (UN) 2022). There are hotspots for groundwater depletion throughout the world, usually in regions where significant amounts of groundwater are drawn out for irrigation or to serve big cities. As a developing nation with a high population density, India witnesses a severe water-scarce scenario.
According to the 1991 and 2001 census data, India’s per capita surface water availability is 2,300 and 1,900 m3, respectively which are projected to drop by 1,400 and 1,190 m3 by 2025 and 2050, respectively, based only on population projections and the presumption that water resources will remain available (Bhat Reference Bhat2014). Approximately, 680 km3 of developed water resources are currently available (Saleth Reference Saleth2011). However, because of high costs, environmental issues, and interstate water problems, it is challenging to increase supplies beyond this point in a developing country like India. On the other hand, the Ministry of Water Resources (2000) projects that the total water demand will be between 784 and 843 km3 by 2025 and between 973 and 1,180 km3 by 2050, creating a demand–supply gap in water. By 2050, nine basins that account for more than four-fifths of India’s total water use will experience physical water scarcity if the demand–supply imbalance widens (Amarasinghe Reference Amarasinghe2007). Water demand will rise exponentially due to factors including population growth, rising incomes and urbanization, while supply will become more unpredictable and erratic making it more scarce and vulnerable.
Water scarcity: Types and causes
Water scarcity involves a range of conditions including water crisis and water stress. There are various degrees of water scarcity, such as, absolute or severe, chronic, seasonal, temporary, cyclical and life-threatening (Zisopoulou and Panagoulia Reference Zisopoulou and Panagoulia2021). To evaluate the degree, water scarcity is measured in terms of per capita annual water resources (AWRs) (Gosain et al. Reference Gosain, Rao and Basuroy2006). A region is ‘water stressed’ when the availability of water falls below 2000 m3 AWR per person annually, as populations encounter significant challenges during droughts or artificial water shortages (Pereira et al. Reference Pereira, Cordery and Iacovides2009). If the value of AWR per person falls between 1,000–1700 m3 per capita, the region comes under ‘regular water stress’ (Food and Agriculture Organization (FAO) 2003). Numerous regions across the globe endure more acute situations with 500–1,000 m3 or even less than 500 m3 of water availability per person annually, which can be classified as ‘chronic water shortage or water scarcity’ and ‘absolute or severe water scarcity’, respectively (FAO 2003) (Figure 2). Numerous measures, including the Water Stress Index (WSI), Withdrawal-to-availability Ratio (WTA), Social WSI (SWSI), Water Poverty Index (WPI) and others, can be used to quantify water shortage (Damkjaer and Taylor Reference Damkjaer and Taylor2017). Additionally, groundwater flow simulation codes like modular three-dimensional finite-difference ground-water flow model (MODFLOW) and contamination simulation codes such as a modular three-dimensional multi- species solute transport model (MT3DMS) and/or simulation of three-dimensional variable-density groundwater flow with multi-species solute and heat transport (SEAWAT) are essential for assessing the impacts of climate change on groundwater systems. These tools allow for quantitative and qualitative analysis, aiding in effective groundwater management. By simulating various scenarios, they help predict changes in groundwater levels and contamination spread, ensuring sustainable water resource planning (Harbaugh Reference Harbaugh2005; Langevin et al. Reference Langevin, Thorne, Dausman, Sukop and Guo2008; Zheng and Wang Reference Zheng and Wang1999).

Figure 2. Various degrees of water scarcity based on per capita AWR availability.
Types of water scarcity
According to Seckler et al. (Reference Seckler, Amarasinghe, Molden, Silva and Barker1998), there are two primary forms of water scarcity: physical and economic.
Physical water scarcity
This is also known as ‘absolute water scarcity’, which usually occurs when water is unable to meet all demands (human and environmental flows) due to a fundamental absence of water (FAO 2020). Physical water shortage is typically observed in arid and semi-arid countries and is characterized by severe environmental degradation, diminishing groundwater and unbalanced water allocations (Giordano et al. Reference Giordano, Barron, Unver, Campanhola and Pandey2019). As reported by the FAO of the UN (Food and Agriculture Organization 2020), 1.2 billion individuals reside in regions experiencing physical water scarcity (Figure 3). Farmers often necessitate new irrigation wells in these areas and submit applications for permissions. The competent authority evaluates if extracting water from the well could deplete the water levels of adjacent wells. Groundwater flow models, such as MODFLOW, an open-source model provided by the U.S. Geological Survey, have been employed to model and forecast groundwater flow and depletion rates for the past 30 years (Winston Reference Winston2022). Furthermore, a newly developed MODFLOW framework, known as MODFLOW 6 has been introduced to accommodate numerous models within a single simulation (Hughes et al. Reference Hughes, Langevin and Banta2017). Physical water scarcity is often seasonal and tends to grow dramatically due to rapid population growth along with climate change (Ingrao et al. Reference Ingrao, Strippoli, Lagioia and Huisingh2023). Both blue and green components of water footprints are affected by physical water scarcity. In agricultural production, when a rainfall regime is unable to meet the crop’s water requirements, green water scarcity (GWS) occurs (Rosa et al. Reference Rosa, Chiarelli, Rulli, Dell’Angelo and D’Odorico2020). Similarly, BWS is noticed when the water requirements for irrigation get restricted due to insufficient blue water (Ingrao et al. Reference Ingrao, Strippoli, Lagioia and Huisingh2023). Food and energy security are directly impacted by BWS, which is increasingly perceived as a global socio-environmental hazard (D’Odorico et al. Reference D’Odorico, Chiarelli, Rosa, Bini, Zilberman and Rulli2020).

Figure 3. Water scarce regions in the world [data source: World Water Assessment Programme (2012)].
Economic water scarcity
Economic water scarcity (EWS) is the state in which there are physically abundant renewable blue water resources, but society’s access to those resources is restricted due to a lack of institutional and financial resources (Molden Reference Molden2013; Rijsberman Reference Rijsberman2006). An early definition of EWS identified nations with sufficient renewable water resources to fulfill present and future water demands, but which would require significant reforms to their water development strategies in order to fully utilize their freshwater resources (Seckler et al., Reference Seckler, Barker and Amarasinghe1999). EWS is caused either by inadequate infrastructure, which consists of storage, timely distribution and access to water (Ahopelto et al. Reference Ahopelto, Veijalainen, Guillaume, Keskinen, Marttunen and Varis2019), or due to poor management of water resources that hinders access to abundant water resources (Bond et al. Reference Bond, Burrows, Kennard, Bunn, Sabater, Elosegi and Ludwig2019). Regions witnessing EWS generally have adequate water resources to meet both human and ecological needs, but there is a limitation to access (Petruzzello Reference Petruzzello2023). As per the estimation of Food and Agriculture Organization (2020), over 1.6 billion individuals experience EWS (Figure 3). Underdevelopment and poor management can contaminate readily available water supplies or render them unfit for human consumption. This can also be the outcome of unregulated water utilization for industrial and agricultural systems, frequently at the expense of the general public. In addition, water use inefficiency is also the consequence of underestimating the economic value of water as a limited natural resource (Ingrao et al. Reference Ingrao, Strippoli, Lagioia and Huisingh2023). The relationship between access and restriction and possession and dispossession of water resources is ultimately influenced by institutional, political and power dynamics, according to emerging research (Boelens et al. Reference Boelens, Hoogesteger, Swyngedouw, Vos and Wester2016; Huitema et al. Reference Huitema, Mostert, Egas, Moellenkamp, Pahl-Wostl and Yalcin2009; Ostrom Reference Ostrom2015). Therefore, the range of sociopolitical elements that interact at various scales must be taken into account to fully comprehend EWS. EWS can further be categorized as GWS, BWS, agricultural EWS and total water scarcity (TWS) (Figure 4). When crop production becomes stressed and irrigation is required to increase yields since green water or rainfall is not enough to support it, the ratio of irrigation water requirement to the total crop water requirement (CWR) is known as GWS (Rosa et al. Reference Rosa, Rulli, Davis, Chiarelli, Passera and D’Odorico2018). BWS comes when there is not enough renewable blue water available and irrigation is not sustainable, the CWR cannot be met. In these situations, irrigation reduces freshwater supplies and affects environmental flows and the ratio of societal demand for blue water to the availability of renewable blue water is known as BWS (Vanham et al. Reference Vanham, Hoekstra, Wada, Bouraoui, de Roo, Mekonnen, van de Bund, Batelaan, Pavelic, Bastiaanssen, Kummu, Rockström, Liu, Bisselink, Ronco, Pistocchi and Bidoglio2018). Furthermore, a lack of BWS in the presence of GWS is known as an agricultural economic water shortage (Rosa et al., Reference Rosa, Chiarelli, Rulli, Dell’Angelo and D’Odorico2020). Moreover, when there is a lack of institutional or financial capacity together with GWS and BWS is known as TWS.

Figure 4. Various types of economic water scarcity including green and blue components of water resource availability. Data given in scarcity level is arbitrary. to provide an improved understanding and to distinguish between these scarcities more prominently.
Causes of water scarcity
Although there are innumerable reasons for the severe water scarcity, which many nations of the world are facing nowadays, however, only a few major causes have been discussed in this paper.
Climate change and droughts
The variability of weather patterns due to climate changes and extremes has resulted in the occurrence of inadequate water availability, water scarcity and contaminated water supplies (UNICEF 2023). The probability of occurrence of severe droughts in numerous areas of the world is more likely as a result of climate change (Clarke et al. Reference Clarke, Otto, Smith and Harrington2022). During droughts, as rainfall and precipitation decrease to a significant extent, soils and crops dry out easily and die, eventually. This has a significant influence on water levels in lakes and reservoirs when it happens over a long period in conjunction with heat wave events, leading to water scarcity for adjacent communities and cities (Lai Reference Lai2022). Between 2001 and 2018, approximately 74% of natural disasters were related to water, such as floods and droughts. As climate change progresses, it is anticipated that the occurrence and severity of these events will escalate (UNICEF 2023).
Population growth
The world’s demographical scenario has experienced a significant increase over the last century, and it is projected to continue rising by 2030 and 2050 (Foresight 2011). At present, the global population is expanding by approximately 83 million individuals each year (Islam and Karim Reference Islam, Karim, Farahani, Vatanpour and Taheri2020). Currently, a considerable fraction of the world’s inhabitants, approximately 41%, reside in river basins experiencing water stress (UNESCO 2022). To feed this huge ever-growing population, food production must rise by 50% by the year 2030. Due to this augmentation in food production, water demand also needs to be increased by 40 to 50%, while municipal and industrial water requirements will escalate by 50 to 70% (UNESCO World Water Assessment Programme 2018).
Water pollution
Water pollution occurs when harmful substances contaminate water sources, such as rivers, streams, oceans and aquifers, making it unsafe for both humans and the environment (Denchak Reference Denchak2023). Pollution can originate from various sources, such as agricultural runoff carrying pesticides and fertilizers, untreated human wastewater and industrial wastes. Groundwater is also vulnerable to pollution, with various pollutants seeping into underground aquifers. The consequences of contamination can be immediate, such as when harmful bacteria from human waste pollute water, or can accumulate over time, such as the long-term effects of toxic substances from industrial processes on the environment and the food chain (Akpor and Muchie Reference Akpor and Muchie2011).
Agricultural practices
According to the World Economic Forum (2015), the agricultural sector is responsible for depleting 70% of the world’s freshwater supply. Unfortunately, as much as 60% of this precious resource is lost due to inappropriate irrigation systems, poor application techniques and the cultivation of water-intensive crops that require more water than is available in their growing environment (Qureshi and Ashraf Reference Qureshi and Ashraf2019). This excessive water consumption is leading to the depletion of rivers, lakes and underground water sources. Several countries that produce significant amounts of food, including Australia, China, India, Spain and the United States, have already exceeded or are on the verge of exceeding their water resources’ capacity (Schwab Reference Schwab2019). Furthermore, agriculture contributes significantly to freshwater pollution, primarily through the indiscriminate use of fertilizers and pesticides, posing a threat to humans and other species.
The above-mentioned causes are major ones across the globe, but there are a few other causes as well, which are mentioned below:
Water wastage: While some regions may not face water scarcity issues, they can still play a role in aggravating the water crisis by inefficiently using potable water (Bensen Reference Bensen2022).
Government policies: When a society is unable to overcome supply–demand gaps in resources such as water by responsive instructional and technological measures, institutional scarcity sets in. Similarly, political scarcity occurs when political subordination prevents a group of people from using water resources (Hussain et al. Reference Hussain, Wang, Wang, Yang, Arfan, Hassan, Wang, Azam and Faisal2022). The population of certain nations, particularly those governed by dictatorial regimes may face water scarcity due to tight regulations imposed by those in power (Chakkaravarthy and Balakrishnan Reference Chakkaravarthy and Balakrishnan2019). Such cases are profound in many developing nations, for example, Karachi in Pakistan, which is experiencing the worst water crisis in its history of the past 30 years, not as a result of a natural shortage but rather as a result of poor planning and administration (Tayyab et al. Reference Tayyab, Abbas and Hussain2022). This highlights the significance of institutional concerns in managing water supplies as water scarcity can result from institutional failings that cannot sustain a steady supply (Dell’Angelo et al. Reference Dell’Angelo, Rulli and D’Odorico2018; Ingrao et al. Reference Ingrao, Strippoli, Lagioia and Huisingh2023).
Distance: Numerous remote locations, such as deserts or secluded areas, across the globe, face water scarcity due to their lack of proximity to water sources (Rinkesh Reference Rinkesh2023) (https://www.conserve-energy-future.com/causes-effects-solutions-of-water-scarcity.php).
Insufficient infrastructure: Outdated water treatment plants, inefficient sewerage systems and leakage in poorly constructed pipes can cause the loss of billions of gallons of water (Bensen Reference Bensen2022).
Water scarcity: A hindrance to sustainable development
Water is a crucial resource for sustaining life on Earth, serving an essential role in both the environment and society (D’Odorico et al. Reference D’Odorico, Chiarelli, Rosa, Bini, Zilberman and Rulli2020). As a fundamental need, water is necessary for various purposes, such as domestic use, industrial production and agriculture (Mancini et al. Reference Mancini, Benini and Sala2016). Sustaining water resources is also essential for current and future generations, serving critical purposes like drinking, agriculture, industry and ecosystem maintenance. The UN General Assembly recognized access to safe water as a human right and adopted SDG 6 which underscores the global commitment to ensuring water and sanitation for all by 2030. However, as a result of climate change, population growth and industrial development, water resources are becoming increasingly strained in both quality and quantity, and achieving universal clean water access remains challenging (Famiglietti Reference Famiglietti2014; Taylor et al. Reference Taylor, Scanlon, Doell, Rodell, Beek, Wada, Longuevergne, Leblanc, Famiglietti, Edmunds, Konikow, Green, Chen, Taniguchi, Bierkens, Macdonald, Fan, Maxwell, Yechieli and Treidel2013). The statistics published by the UN in 2018 showed that more than 2.2 billion people do not have access to safely managed clean water, which affects half of schools and 25% of healthcare facilities (Irannezhad et al. Reference Irannezhad, Ahmadi, Liu, Chen and Matthews2022). In addition, according to the World Health Organization and UN Children’s Fund report, 4.2 and 3 billion people, respectively, lack access to essential sanitation and handwashing facilities (WHO-UNICEF 2020). As a result, there is a growing consensus that the traditional approach of ‘use and discard’ is no longer sustainable for managing vital natural resources, including water (Chartzoulakis and Bertaki Reference Chartzoulakis and Bertaki2015).
A global scenario of water scarcity and its implications
Water scarcity has become one of the most significant problems in the 21st century, and it is projected that by 2025, 2.7 billion people will face water scarcity (UNESCO 2003). The World Economic Forum (2015) stated that water scarcity is the primary societal risk, globally, surpassing even the spread of infectious diseases, and it is anticipated to increase by 43% by 2025. By 2080, more than 56.2% of the world’s population is expected to be affected by water scarcity as a high-impact and high-probability societal risk (Veldkamp et al. Reference Veldkamp, Wada, Aerts and Ward2016). In 2019, a serious water crisis affected 1.8 billion people in 17 nations worldwide (Dormido Reference Dormido2019). Extreme weather events and changes in water cycle patterns make it challenging to access safe drinking water, particularly for vulnerable children. Over 40% of children will reside in regions with extreme water stress by 2040, and more than 1,000 children under the age of five pass away every day from illnesses linked to poor access to water, sanitation and hygiene (UNICEF 2017). Nations, such as India, Pakistan, San Marino, Botswana and Turkmenistan, are among the countries affected by water scarcity in Asia, Africa, Europe and Central Asia (Arora and Mishra Reference Arora and Mishra2022).
The World Resources Institute’s Aqueduct Water Risk Atlas analysis also showed that 12 of the 17 countries experiencing water scarcity are located in the Middle East and North Africa (MENA) (Dormido Reference Dormido2019). The MENA have the greatest physical water stress due to their rapidly expanding, highly populated metropolitan centers that demand more water, as well as their lower rainfall pattern compared to other areas. Since practically, all of the food in the United Arab Emirates (UAE) is imported, less water is needed for cultivation. The desalination of plentiful ocean water, a costly and energy-intensive procedure, is also a major source of income for the UAE and other affluent MENA nations.
The local effects of physical water stress, in addition to the escalation and dissemination of freshwater pollution, are contributing to the growing prevalence of water shortage. On average, 10% of the world’s population lives in countries with high or critical water stress. The number of large cities experiencing water scarcity would rise from 193 (37%) to 292 (56%) and the global urban population experiencing water scarcity would double from 933 million (33%) in 2016 to 1.693–2.373 billion (35–51%) in 2050 (He et al. Reference He, Liu, Wu, Pan, Fang, Li and Bryan2021). A global baseline water stress interactive map was developed using the Data Basin tool (https://databasin.org/maps/new/#datasets) by the Conservation Biology Institute (CBI 2023) that indicates the major part of the United States, Europe, Middle East, Central Asia, India, China, East Asia and South-Eastern Australia fall under ‘High (40–80%)’ to ‘Extreme (>80%)’ water stress (Figure 5). Nevertheless, many of these nations, particularly the developed ones have the potential to fulfill their water demands by taking appropriate mitigation approaches and adaptation.

Figure 5. Global water stress severity map. The color bar in the legend (right) indicates the severity of baseline water stress from ‘Low’ (<10%) as ‘yellow’ to ‘Extremely high’ (>80%) as ‘dark brown’ in colors [data source: Data Basin, access provided by the Conservation Biology Institute (CBI), Accessed in May 2023].
Due to the growing demand, developing countries, especially are located in central to northeastern Asia, western Asia and eastern and northern Africa, often lack the financial support to invest in hydraulic infrastructure (Emile et al. Reference Emile, Clammer, Jayaswal and Sharma2022; Lakmeeharan et al. Reference Lakmeeharan, Manji, Nyairo and Poeltner2020; Oki and Quiocho Reference Oki and Quiocho2020). In addition, excessive groundwater pumping and wasteful and inefficient water management systems are further causes for worry in developing nations (Johnson et al. Reference Johnson, South and Walters2016; Winschewski Reference Winschewski2017). The largest urban populations experiencing water scarcity reside in China (159 million) and India (222 million). By 2030, the water demand is expected to be twice the available supply, leading to severe water scarcity for millions in India. Highly populated cities like Delhi, Bangalore, Chennai and Hyderabad may reach zero groundwater levels shortly (Emile et al. Reference Emile, Clammer, Jayaswal and Sharma2022). Hence, appropriate measures need to be taken to reduce water usage and preserve more for a sustainable water supply in developing nations including India.
Water scarcity in India – A case example of a developing country
India is one of the world’s most water-stressed countries, with only 4% of the world’s water resources yet being home to 18% of the world’s population (The World Bank 2023). In 2019, the NITI Aayog think-tank issued a report indicating that around 600 million individuals in India were experiencing a ‘high’ to ‘extreme’ water crisis (NITI Aayog 2019). A baseline water stress interactive map for India was developed using the India Water Tool 2.0 (IWT 2.0) software platform by the World Resources Institute (WRI 2023) that indicates ~54% of regions of India are currently facing ‘high’ to ‘extremely high’ water stress, which is profound in the western, southern and south-eastern parts of India (Figure 6). The Water Resources Group has predicted that India will experience a severe water crisis by 2030, with only 50% of its required water supply available (Mahato et al. Reference Mahato, Upadhyay and Sharma2022). The current usage and exploitation patterns suggest that the per-capita water availability has already decreased by approximately 75% since its independence in 1947 (Mohan Reference Mohan2023). The annual per capita availability of fresh water was 5,177 m3 in 1951, which significantly came down to 1,545 m3 in 2011 (Dubbudu Reference Dubbudu2016). The current situation suggests that this figure has further declined to 1,368 m3 in 2019 and is anticipated to reduce even more to 1,296 m3 by 2025. If this trend continues, the accessibility of freshwater is projected to decline to 1,140 m3 by 2050 (Upadhyay Reference Upadhyay2020; Ritchie and Roser Reference Ritchie and Roser2018). According to the water quality index, India is ranked 120th out of 122 countries, with almost 70% of its water supply being polluted (Srivastava et al. Reference Srivastava, Pawar and Srivastava2023). The report also warned that within a few years, only 21 major cities, including the capital city of New Delhi, would exhaust their groundwater supplies. Back in 2019, Chennai, the southern metropolis faced an intense water shortage, prompting officials to transport water by train from approximately 200 km to salvage the situation (Ahluwalia and Kesavan Reference Ahluwalia and Kesavan2021). However, today, Chennai has achieved a significant milestone by becoming the first Indian city to adopt large-scale recycling of its wastewater to fulfill the non-drinking water demands of its industries (The World Bank 2023). Another latest report indicated that the number of urban dwellers around the world experiencing water scarcity will reach 2.4 billion (one-third to nearly half of the global urban population), with India being the country expected to be hit the hardest (UNESCO World Water Assessment Programme 2023).

Figure 6. Baseline water stress severity map of India. The color bar in the legend (right) indicates the severity of water stress from ‘Low’ (<10%) as ‘yellow’ to ‘Extremely high’ (>80%) as ‘dark brown’ in colors [data source: India Water Tool 2.0, access provided by World Resources Institute, Accessed in 2023].
Surprisingly, more than 20 million wells are operating with free power supply, resulting in excessive water wastage, increased salinity, and rapid depletion of groundwater resources in various parts of the country (Central Water Commission 2017). The contamination of the country’s water supply has reached alarming levels, with 70% of it being polluted (Schneider Reference Schneider2018), resulting in an estimated 200,000 deaths per year (Temple Reference Temple2019). Furthermore, more than 500 million people, which accounts for 40% of the population, will lack access to drinking water by 2030 (NITI; Aayog 2019).
Water demand and scarcity in agriculture
Water requirement and crop production
Water is essential at the critical growth stages of a plant’s development as at this stage, plants require an adequate amount of water to grow properly (Das et al. Reference Das, Chapman, Christopher, Choudhury, Menzies, Apan and Dang2021). The critical growth stage of a plant varies depending on the type of crop, but it generally includes the period from seedling emergence to the early reproductive stage. In addition, water requirement for crops varies between crop types (Prasad Reference Prasad2002) (Table 1). The growth stage of a plant from seedling emergence to the early reproductive stage may require additional water supply through irrigation for proper growth and development if the natural sources of water and/or stored soil moisture in the field are inadequate. During the rapid vegetative development stage, a water shortage can reduce the height of maize plants (Cakir Reference Cakir2004) resulting in low yields. Likewise, Das et al. (Reference Das, Christopher, Apan, Choudhury, Chapman, Menzies and Dang2020) reported that water limitation can be the single largest constraint to wheat production in rain-fed conditions. For example, water stress at a critical wheat development stage has the potential to decrease yield from ~3.0 to ~1.0 t/ha within a field in Australian dryland environments (Das et al. Reference Das, Christopher, Apan, Choudhury, Chapman, Menzies and Dang2020). Studies (Hatfield et al. Reference Hatfield, Boote, Kimball, Ziska, Izaurralde, Ort, Thomson and Wolfe2011; Wang et al. Reference Wang, Li, Zhang, Chen and Li2021) report that adequate irrigation water during critical crop development stages can significantly increase the number of ears, and seeds per ear, and improve soil and air temperature, leading to increase photosynthesis and yields of maize and tomato crops. It is, therefore, crucial to manage irrigation during the critical growth stage to ensure that plants receive adequate water and maintain optimal conditions for growth and development. However, it is widely accepted that crop production improves with root zone water availability up to saturation, after which there is no influence (Hillel Reference Hillel1997).
Table 1. Water requirements of different crops

Adopted from (Kapuria and Banerjee Reference Kapuria and Banerjee2022).
Agronomic simulation tools like the water crop productivity simulation model (AquaCrop), environmental policy integrated climate (EPIC), and agricultural policy/environmental extender (APEX) are crucial for predicting crop yields and assessing the impacts of climate change on agriculture. AquaCrop focuses on simulating crop water productivity under varying conditions, aiding in water management strategies (Steduto et al. Reference Steduto, Hsiao, Raes and Fereres2009). EPIC evaluates the long-term effects of climate and management on soil and crop performance, providing insights into environmental sustainability (Williams et al. Reference Williams, Dyke, Jones, William, Gaylord and Marshall1983). Both AquaCrop and EPIC are utilized at the farm scale, while APEX can be employed for large-scale watersheds (Feng et al. Reference Feng, Flanagan, Engel, Yang and Chen2020). APEX also extends EPIC’s capabilities by analyzing the effects of agricultural practices on large-scale watershed hydrology and crop production (Gassman et al. Reference Gassman, Williams, Wang, Saleh, Osei, Hauck, Izaurralde and Flowers2010). These tools facilitate informed decision-making by simulating future scenarios, thus supporting agricultural resilience against climate variability.
Irrigation water losses in agriculture
Approximately, 70% of the water utilized worldwide is used for agriculture, mostly for irrigation (World Economic Forum 2015). From about 40 million hectares in 1900 to more than 260 million hectares in 1999, the world’s irrigated acreage has expanded more than six times (Postel et al. Reference Postel, Polak, Gonzales and Keller2001). The 18% of cropland that is irrigated now provides 40% of the world’s food. According to Jensen (Reference Jensen1993), irrigated land grows by about 1% per year, and Rosegrant and Cai (Reference Rosegrant and Cai2002) predicted that the need for irrigation water will be increased by 13.6% by 2025. When irrigation is applied to any agricultural field, not the entire amount of the irrigated water can be used by crops as some of the water can be lost during distribution and application. For example, Chartzoulakis and Bertaki (Reference Chartzoulakis and Bertaki2015) reported that while applying irrigation water to a farm, a total of 35% of the water was lost during irrigation distribution, field application and farm distribution, and the remaining 65% of water was used by crops. Hence, it is necessary to ensure efficient irrigation practices to reduce losses of water during application to the field. Water use efficiency of crops can be heavily reliant on the methods of irrigation practices (Table 2). The depletion of aquifers and potential negative environmental externalities resulting from intensive groundwater pumping for irrigation have a detrimental economic impact on agriculture and related businesses.
Table 2. Country-wise different irrigation methods for optimum water use efficiency

Implications of water scarcity in agriculture – A global perspective
Water shortage is a threat to agriculture, globally (UNESCO World Water Assessment Programme 2018). Its consequences can be disastrous, resulting in failed crops, lower yields, food insecurity and the abandonment of lands. Over the past 40 years, water demand in agriculture has grown globally by about 1% per year due to a combination of this massive population expansion, socioeconomic development and changing consumption habits (Department of Economics and Social Affairs, Population Division 2017). A report revealed that municipal withdrawals rose by 3% between 2010 and 2018, while agricultural withdrawals rose by 5% (Food and Agriculture Organization 2020). The demand for water in global agriculture will increase by 60% by 2025 (Alexandratos and Bruinsma Reference Alexandratos and Bruinsma2012).
In California, United States, the ongoing drought has had a significant impact on agriculture. California is a leading agricultural state in the United States and the crop production relies heavily on irrigation. However, the drought has reduced the availability of water for irrigation, leading to significant losses in agricultural production. According to a report by the University of California, the drought in 2015 resulted in a $1.84 billion loss in agricultural revenue and the loss of 10,100 seasonal jobs (Kerlin Reference Kerlin2015). In addition, the farmers in California have had to reduce their planting area or leave farmland fallow due to the limited availability of water for irrigation. It also emphasized how water shortage is a problem in many of the world’s key agricultural regions and that it will probably persist in the years to come. Water shortages and climate change might cause a 23% drop in global food output by the year 2,100 (Schewe et al. Reference Schewe, Heinke, Gerten, Haddeland, Arnell, Clark, Dankers, Eisner, Fekete, Colón-González F, Gosling, Kim, Liu, Masaki, Portmann, Satoh, Stacke, Tang, Wada and Kabat2014). The study also highlighted that water scarcity will be the primary driver of food production loss in many regions across the world, including the Middle East, North Africa, South Asia and Southern Europe. The UN (UNESCO 2022) predicted that by 2030, water scarcity could reduce crop yields by 20% globally causing a ‘significant threat’ to food security. By 2040, water shortage may threaten up to 30% of the world’s food production (Zhang et al. Reference Zhang, Wang, Chen, Wang and Wu2021). In the Mediterranean area, water shortage might threaten up to 40% of the region’s overall crop revenue by 2040 (Mekonnen et al. Reference Mekonnen, Hoekstra, Alemu and Ghezehei2020). Wheat and maize production might decrease by 10–20% because of water constraints by 2050 in Southeast Asia (Gupta et al. Reference Gupta, Narain, Arora and Singh2021). In Pakistan’s Indus River Basin, it was reported that lack of water has significantly reduced agricultural productivity and compelled farmers to switch from water-exhaustive crops like rice to less water-intensive crops like wheat (Shah et al. Reference Shah, Ul-Hassan, Khattak and Ahmad2019). Due to the lack of access to irrigation technology and low financial resources, smallholder farmers in developing and underdeveloped countries are especially exposed to the consequences of water shortage (Djaman et al. Reference Djaman, O’Neill, Owen, Smeal, Koudahe, West, Allen, Lombard and Irmak2018). In sub-Saharan Africa, 95% of smallholder farmers rely on seasonal rainwater for crop production (Giller et al. Reference Giller, Corbeels, Nyamangara, Triomphe, Affholder, Scopel and Tittonell2011).
India, with over 600 million farmers of a total of 1.4 billion population, stands as the second-largest wheat and rice producer globally, contributing to 10% of the world’s crop production (Erickson Reference Erickson2021). However, extensive usage of groundwater has made India one of the largest consumers of water resources in the world, facing high water stress and having particularly fragile water resources (Carrington Reference Carrington2016). The excessive pumping of groundwater through tube wells has enabled Indian farmers to increase their food production significantly since the 1960s, even during dry seasons which has resulted in ‘critically low groundwater availability’ in the northwest and south regions of the country (Jain Reference Jain2018). The severe impact of water scarcity was noticeable in early 2016 when 300 million Indians encountered acute water scarcity due to two consecutive seasons of weak monsoons, resulting in a significant decline in farm production (Bera Reference Bera2017). Due to the same reasons, India experienced a decline in sugar production from a peak of 28.3 million tons in 2013–2014 to 25.1 million tons in 2015–2016, and a seven-year low of 21.3 million tons during 2016–2017 (Bera Reference Bera2017). Due to increasing water scarcity, many farmers are switching crops, for example, in the state of Tamil Nadu, farmers have switched to cultivating sesame instead of groundnut due to its low water requirement (Haq Reference Haq2023). Some studies also indicated that if farmers in areas where groundwater is being excessively extracted were to lose access to it entirely without having any other alternative sources of irrigation water to compensate. This could lead to a 20% decline in winter harvests nationwide, with the most severely impacted regions experiencing a 68% reduction (Yeung et al. Reference Yeung, Gupta and Kann2021). By the year 2030, 74% of the total wheat-cultivated land and 65% of the rice-cultivated land in India will encounter notable water scarcity (NITI; Aayog 2019). A 36-years age farmer possessing 17 acres (7 ha) of agricultural land in the state of Punjab in India, had told in an interview that farmers in his region were able to produce only 8 quintals (800 kg), compared to the typical yield potential of 18 quintals (1,800 kg) per acre of wheat cultivation. As a cause of this drastic fall in wheat production, he accused water scarcity arising out of rising temperatures and erratic rainfall (Guram Reference Guram2022). To tackle this critical situation, many young farmers are adopting millet farming in Punjab as a profitable substitute for rice and/or wheat due to its low water requirement (Bhogal et al. Reference Bhogal, Gree, Petrie and Dixit2022).
Prospects to adopt viable approaches to managing agricultural water
The time has come to consider the adaptation of existing water harvesting technologies to circumvent the situation of grave water scarcity arising out of the indiscriminate use of underground water to enhance crop productivity. The strategic factor for the selection of a particular water-conserving technique, the most essential criterion is to consider the ‘efficiency’ parameter, both in terms of utilization and conservation of each of the available techniques (Figure 7).

Figure 7. Components of irrigation scheduling for increasing water usage efficiency using marginal waters for irrigation in agriculture.
Evaluating prices and markets of water in agricultural water use for sustainable development
Prices and markets for water, along with institutional frameworks, play a critical role in ensuring the sustainable development of agricultural water use by promoting efficiency, equitable access and conservation. Hence, it is important to evaluate prices and markets of water. Water pricing mechanisms, such as volumetric pricing or tiered tariffs, can encourage farmers to use water more efficiently, reducing waste and over-extraction from aquifers. Several studies highlight the crucial role of water pricing and market mechanisms in promoting sustainable agricultural water use. Water pricing policies, when designed effectively, can incentivize farmers to adopt more water-efficient practices, thus improving water productivity. For example, In Iran, the implementation of such policies led to a notable shift toward optimizing water use due to the economic pressures associated with higher water costs. Markets for water can also enable more efficient allocation, allowing scarce water resources to be used where they generate the highest agricultural returns (Zamani et al. Reference Zamani, Azadi, Mortazavi, Balali, Moghaddam and Jurík2020). Furthermore, In Brazil’s semi-arid areas, the implementation of water pricing policies can significantly reduce agricultural water use by incentivizing farmers to adopt more efficient irrigation methods, shift to less water-intensive crops and invest in water-saving technologies. This approach helps alleviate the effects of water scarcity, particularly during droughts (Marengo et al. Reference Marengo, Galdos, Challinor, Cunha, Marin, Vianna, Alvala, Alves, Moraes and Bender2022). However, it is essential to carefully design pricing structures to maintain fair access for small-scale farmers and prevent adverse economic effects on vulnerable communities. Similarly, water markets, which allow for the trading of water rights, help in allocating water resources where they are most valued, increasing overall economic efficiency. Australia’s Murray-Darling Basin provides a notable example where water trading facilitated more efficient use, resulting in higher agricultural productivity and improved environmental outcomes (Grafton et al. Reference Grafton, Libecap, Edwards, O’Brien and Landry2012). Institutional frameworks, including legal and regulatory systems, also play a crucial role. Effective water governance, such as establishing clear water rights and regulatory oversight, ensures that water allocation aligns with long-term sustainability goals. For example, the European Union’s Water Framework Directive mandates sustainable water use across sectors, promoting the protection of water ecosystems while supporting agricultural needs (European Commission 2019). These mechanisms – pricing, markets and governance – work in synergy to balance water demand and supply, fostering sustainable agricultural practices.
Improved irrigation systems
Water resources management is the planning, development and administration of water resources concerning water quantity and quality across all water uses (Figure 8). It is made up of institutions, schemes for financial assistance, information systems and groups that support and guide water management. Some of the components of an improved water management practice are discussed below and depicted in Figure 8.

Figure 8. Improved management of water resources concerning water quantity and quality across all water users.
In arid and semi-arid areas, the rising demands for water from municipal and industrial sectors continuously diminish the water allotment for agriculture (Mirdashtvan et al. Reference Mirdashtvan, Najafinejad, Malekian and Sa’doddin2021). Consequently, water availability is generally restricted, which inevitably leads to sub-optimal crop yields. To optimize the use of water resources, improved irrigation techniques that do not rely on meeting the full CWRs need to be implemented. These include deficit irrigation, partial root drying and sub-surface irrigation. It is also essential for improving the performance and sustainability of irrigation systems. In order to decide when to irrigate and how to irrigate, an understanding of crop water needs, weather and soil characteristics is necessary. The proficiency of the farmer significantly impacts the efficacy of irrigation scheduling at the field level. A suitable irrigation schedule has the potential to hinder deep percolation and the movement of fertilizers and agro-chemicals outside the root zone, avoid waterlogging, save water and energy and result in higher yields and better-quality crops (Chartzoulakis and Bertaki Reference Chartzoulakis and Bertaki2015). In addition, some common and widely adopted irrigation practices such as micro-irrigation and fertigation are useful for managing agricultural water use.
Micro-irrigation
Micro-irrigation is a method of applying water to plants at a slow and targeted rate, typically using low pressure. Sub-surface drip, trickle irrigation, micro-sprinklers and bubblers are some of the most common methods used in a micro-irrigation system. The system consists of small-diameter tubing that is placed either on the surface or underground in the field, with small water application devices attached to the tube to supply water directly to the plants at low pressure (Evans et al. Reference Evans, Wu, Smajstrala, Hoffman, Evans, Jensen, Martin and Elliott2007). This technique is essential for conserving water while achieving optimal plant growth. Micro-irrigation systems are particularly useful for widely spaced trees, vine crops and high-value vegetable crops. However, one of the main drawbacks of micro-irrigation is its high installation cost, as well as the increased risk of clogging, particularly when low-quality water is used (Barragan et al. Reference Barragan, Cots, Monserrat, Lopez and Wu2010).
Fertigation
Fertigation is a popular technique in modern agriculture where fertilizers are applied through the irrigation system. It is well-suited for localized irrigation systems that are highly efficient for water application (Shukla et al. Reference Shukla, Sadhu, Chinchmalatpure, Prasad, Kumar and Camus2018). Fertigation involves the direct application of soluble fertilizers to the wetted volume of the soil at recommended concentrations for crops through the irrigation system. However, there are some potential drawbacks to fertigation. These include non-uniform distribution of chemicals when irrigation design or operation is inefficient, fertilization above actual crop needs and excessive utilization of soluble fertilizers.
Modern water-saving agricultural practices
Conservation tillage
Conservation tillage (CT) is a farming practice that involves leaving crop residues on the field after harvest to retain moisture and prevent soil erosion. This method can reduce water use by up to 50% compared to conventional tillage (Kaur and Jat Reference Kaur and Jat2017). The CT can also improve soil health and reduce soil degradation caused by excessive water use. Additionally, it improves soil structure, reduces soil compaction and increases soil organic matter, which can contribute to more efficient water use and improved crop yields (Jat et al. Reference Jat, Sahrawat Kassam, Friedrich, Jat, Sahrawat and Kassam2013). This type of tillage also helps to increase soil moisture retention and improve crop yield in dryland farming systems (Govaerts et al. Reference Govaerts, Verhulst, Castellanos-Navarrete, Sayre, Dixon and Dendooven2009). It is a promising agricultural practice for efficient water use in India, where water resources are limited, and soil degradation is a major concern. It reduced water use by up to 40% compared to conventional tillage practices in Haryana (Singh et al. Reference Singh, Mishra, Singh, Singh, Sharma and Verma2017) whereas in the western state of India, mainly in Gujrat, water use was reduced by 30% with an increased yield of 20% due to this special type of practice (Gajri et al. Reference Gajri, Arora, Chaudhary and Kumar2013). Conservation tillage (Reduced tillage and Zero tillage) also improves bio-meteorological parameters including leaf area index, photosynthetically active radiation use efficiency (PARUE) along with biological and grain yield compared to conventional tillage in the case of maize during rabi season (Pal et al. Reference Pal, Mondal, Banerjee, Saha and Pramanick2022). It is also a less labor-intensive as well as less capital-intensive technique but plays a vital role in enhancing productivity. A study that took place in the cotton-growing community of Refugio County, Texas, showed that every 1% adaption of CT could turn a county-wide benefit of approximately USD $16,000 (Cusser et al. Reference Cusser, Jha, Lonsdorf and Ricketts2023 ).
Crop shifting
Crop shifting involves planting crops that require less water during dry seasons or in water-limited areas. This can help to reduce the amount of irrigation water needed and prevent soil degradation caused by excessive water use (Acharjee et al. Reference Acharjee, Van Halsema, Ludwig, Hellegers and Supit2019). For example, in the lower Indo-Gangetic plain, traditionally, Boro rice and potato are cultivated, but these crops are highly irrigation intensive causing a steady reduction of groundwater level. Farmers are being recommended to pick maize over rice in order to avert this catastrophe because it uses just one-fifth as much water to cultivate as rice, yields more and offers greater returns to farmers (Kapuria and Banerjee Reference Kapuria and Banerjee2022).
Crop rotation
Crop rotation is a practice where different crops are grown in sequence in a year on the same piece of land. Certain crops like legumes can fix nitrogen in the soil, reduce the need for fertilizers, and therefore reduce water use. As an example, rice-based cropping is the conventional system in lower Indo-Gangetic plains in India, but in recent times, legumes like cowpea, Lathyrus, Bengalgram, Greengram and Blackgram have been incorporated (Lauren et al. Reference Lauren, Shrestha, Sattar and Yadav2008). For example, lentils and chickpeas were successfully cultivated during the rabi season of 2018–2019 and 2019–2020 with residual soil moisture after monsoon rice at the B.C.K.V., Kalyani, West Bengal (Mukherjee Reference Mukherjee2022). Irrigation facility was not applied for these two winter legumes cultivation which not only saved water application as compared to boro rice cost but also generated additional income. Thus, such kind of crop rotations can be beneficial in the current water-scarce scenario.
Mulching
Mulching is an approach that involves covering the soil around the plants with organic materials, such as leaves or straw. In dryland conditions, mulching is an effective method for conserving water, as it helps to maintain soil moisture, lower soil evaporation and control temperature (Kader et al. Reference Kader, Senge, Mojid and Ito2017). It is also extensively used in rain-fed farming systems to save water (Chakraborty et al. Reference Chakraborty, Nagarajan, Aggarwal, Gupta, Tomar, Garg, Sahoo, Sarkar, Chopra, Sarma and Kalra2008; Zribi et al. Reference Zribi, Aragüés, Medina and Faci2015). Plastic sheet mulching is more effective in conserving soil water than wheat straw mulch (Li et al. Reference Li, Hou, Jia, Han, Ren and Yang2013). The primary benefit of mulching is its ability to retain soil moisture by preventing soil erosion and reducing surface evaporation (Qin et al. Reference Qin, Li, Kang, Du, Tong and Ding2016). This, in turn, reduces the need for irrigation during crop cultivation seasons, making mulching a cost-effective and sustainable method for conserving water (Kader et al. Reference Kader, Senge, Mojid and Ito2017).
Use of drought-tolerant crops
Planting drought-tolerant crops can reduce water use and increase crop yields under water-limited conditions (Das et al. Reference Das, Christopher, Choudhury, Apan, Chapman, Menzies and Dang2022). These crops have been developed through breeding programs to survive and produce in dry conditions, making them an ideal choice for areas with low water availability. Different varieties (like Huhan-3, Huhan-7, water-saving and draught-resistant rice (WDR) 48, etc.) of WDR are popular and acceptable among farmers in different parts of China (Luo et al. Reference Luo, Mei, Yu, Xia, Chen, Liu and Li2019).
Other demand-side practices
While the review primarily focuses on water scarcity and its relation to agricultural water management practices as the agricultural sector is the major contributor to the global water crisis, other demand side practices, such as permits, pricing electricity, well spacing requirements and water markets could also be considerable factors, as well. For instance, water pricing mechanisms, such as volumetric pricing or tiered pricing strategies, have been shown to effectively promote water conservation in agriculture by incentivizing farmers to adopt water-saving technologies and practices only when water prices reflect their scarcity (Loch and Adamson Reference Loch and Adamson2015). Moreover, institutional measures like well-spacing regulations can directly impact groundwater sustainability, preventing over-extraction and ensuring equitable water distribution among users (Molle and Closas Reference Molle and Closas2020). Likewise, the establishment of water markets, which allow the trading of water rights, has been successfully implemented in regions like Australia’s Murray-Darling Basin, where such markets enable more efficient water allocation between agricultural and non-agricultural users during periods of scarcity (Grafton and Horne Reference Grafton and Horne2014). These examples highlight the importance of combining agricultural interventions with policy and market-driven measures to optimize water use. Furthermore, it is also necessary to consider how the timing, geographic context and socio-economic conditions determine the adoption of both agricultural and institutional practices. For instance, while precision irrigation technologies, such as micro-irrigation and fertigation, may be critical in areas with high-value crops, institutional solutions like water permits and electricity pricing could be more effective in regions with over-reliance on groundwater or where energy subsidies distort water use behavior (Ma et al. Reference Ma, Zhang, Song and Yu2022). Integrating these considerations would provide a more comprehensive roadmap for achieving sustainable agriculture and water use.
Furthermore, Table 4 summarizes a critical analysis of country-wise water-use efficient techniques, their prevalent conditions, costs and benefits, advantages and limitations.
Agricultural water management – Technological innovations and adoption
Use of super-absorbent polymer hydrogels
Hydrogel is a type of gel in which the liquid constituent is water. Being a hydrophilic substance, they can absorb and retain aqueous solutions that can be up to a hundred times their weight (Buchholz and Graham Reference Buchholz and Graham1998). They have various positive effects on soil, including a better infiltration rate (Helalita and Letey Reference Helalita and Letey1989). They also act as soil conditioners by reducing evaporation rate and moisture stress (Gehring and Lewis Reference Gehring and Lewis1980; Teyel and El-Hady Reference Teyel and El-Hady1981). In floricultural crops, they enhance the ability to retain water in pot soil (Eikhof et al. Reference Eikhof, King and Koevn1994). They also regulate the discharge of pesticides (Rudzinski et al. Reference Rudzinski, Dave, Vaishnav, Kumbar, Kulkarni and Aminabhavi2002), along with water conservation (Nnadi and Brave Reference Nnadi and Brave2011), and protection against soil erosion (Sojka and Lentz Reference Sojka and Lentz1997), which makes it an ideal component for various water-saving applications in agriculture.
Precision agriculture
Precision agriculture is the science and technology that uses data-driven approaches from ultra-high resolution ground-based and aerial sensors and analytical tools, which improve crop productivity and increase the cost-effectiveness of resource management including irrigation management, fertilizer management and pesticide application (Friedl Reference Friedl and Liang2018). It is an innovative approach where farmers can improve productivity, quality and quantity of yield by supplying optimized inputs such as water and fertilizer. Advancements in technology, particularly the accessibility of high-quality satellite images and the swift progress of unmanned aerial vehicle technology, indicate that the utilization of remote sensing technology and the use of big data in precision agriculture is expected to see a rapid upsurge in the next few years (Friedl Reference Friedl and Liang2018).
Rainwater harvesting
Rainwater from roofs, rooftop terraces and impermeable surfaces is collected, stored, treated and distributed for on-site consumption through a rainwater harvesting system (Campisano et al. Reference Campisano, Butler, Ward, Burns, Friedler, DeBusk, Fisher-Jeffes, Ghisi, Rahman, Furumai and Han2017). In developing countries, this system remains the ultimate source of water supply for millions of people (Kim et al. Reference Kim, Han, Kabubi, Sohn and Nguyen2016). However, usage of rainwater harvesting is highly encouraged even in developed countries by laws, as a sustainable way to increase the resilience of the water supply. There are multiple types of rainwater harvesting methods available with various merits and demerits (Table 3).
Table 3. Description of different types of rainwater harvesting systems

Adopted from (Varma et al. Reference Varma, Nandanan, VishakhRaja, Soundharajan, Pérez M, SidharthK and Ramesh2021).
Table 4. A summary of the country-wise adapted water-use efficient techniques

Solar-powered water pumping systems
The purpose of a solar water pumping system is to minimize the use of coal-based electricity and diesel fuel photovoltaic (PV) pumping is one of the most adopted solar energy-operated water pumping systems, in rural villages in many developing countries. The size of the PV array and the amount of solar radiation determines the flow rate of pumped water. When compared to a traditional pumping system, a well-designed PV system yields considerable long-term cost savings with a minimum water application rate (Kumar et al. Reference Kumar, Bibin, Akash, Aravindan, Kishore and Magesh2020).
Cloud-based irrigation system
A cloud-based irrigation system consists of soil moisture and humidity sensors, Arduino Uno, central cloud storage and mobile apps. Soil moisture and humidity sensors are used to continuously gather data on soil moisture and humidity. The Arduino Uno gadget receives this information which is delivered to a centralized cloud. Mobile applications are connected to this cloud. Farmers can use mobile applications to set the values for moisture and humidity. Furthermore, a smart irrigation system will activate sprinklers or water pumps if the farmer’s specified range for soil moisture and humidity is met (Kaur et al. Reference Kaur, Bhatt and Raja2024).
Conclusions
Water scarcity can be caused either due to man-made incidences or natural events, but it has disastrous effects on the well-being and prosperity of billions of people, globally. In the coming years, numerous regions around the world, including India, will need greater freshwater resources to fulfill the enormous demands of water and food in the face of the uncertainties of climate fluctuations and other environmental constraints. Various studies have highlighted the common practices of over-exploiting natural resources in India, especially affecting soil health and groundwater table. As 80% of total water consumption goes to the Agricultural sector in India, it has a significant role in averting the aggravation of this critical situation. With almost half of the Indian population being engaged in this sector, a decline in the growth rate by a huge drop in the gross domestic product will be a negative consequence due to water-related losses. In order to address this globally critical issue, the UN has included it as one of the primary objectives of Sustainable Development Goal-6 (SDG 6), which states ‘to ensure availability and sustainable management of water and sanitation for all by 2030’. A total of eight targets come under SDG 6 which takes into consideration – safe and affordable drinking water by 2030 (target 6.1), services for sanitation and hygiene (target 6.2), treatment and reuse of wastewater, improvement of water quality (target 6.3), increasing water use efficiency and freshwater supplies (target 6.4), implementation of integrated water resources management (target 6.5), protection and restoration of water-related ecosystems (target 6.6), international support and capacity building support (target 6A) and lastly, support and strengthen the participation of communities in water and sanitation management (target 6B) (https://sdgs.un.org/goals). To achieve this target, specific adaptation strategies for water management should be followed that have been discussed thoroughly in this study including evaluating water pricing and markets, adaptability of various water-use efficient techniques and their costs and benefits across different regions, improved irrigation systems, such as micro-irrigation, fertigation, deficit irrigation and so on, modern water-saving agricultural practices like conservation tillage, crop shifting, crop rotation, mulching, use of drought tolerant crops. Additionally, innovative technologies for water management approaches like the use of super-absorbent polymer hydrogels, biochar and precision agricultural practices are also discussed to provide a thorough understanding.
Overall, this review enhances our existing knowledge by synthesizing the current research, case studies and policy frameworks. The paper consolidates insights on the complex interactions between water scarcity and agricultural demands, highlighting how water shortages exacerbate food insecurity, increase vulnerability of livelihoods and obstruct progress toward the UN SDGs. By critically evaluating the effectiveness of adaptation strategies including technological innovations, water management policies and sustainable agricultural practices, this review equips researchers with a comprehensive understanding of the science and gaps in current research. Furthermore, practitioners and policymakers will find valuable information and recommendations to guide water-efficient agricultural practices, inform equitable water distribution policies and develop actionable plans to counter the global water crisis. This synthesis of impacts and solutions is crucial for fostering resilience in water-scarce regions and promoting global sustainability in the face of growing environmental pressures.
Open peer review
To view the open peer review materials for this article, please visit http://doi.org/10.1017/wat.2024.16.
Data availability statement
No new data were created in this study. Data is included in the manuscript. Data sharing does not apply to this article.
Acknowledgments
The corresponding author would like to extend his sincere thanks to all the co-authors who contributed to preparing the manuscript.
Author contribution
A. B.: Conceptualization, Methodology, Formal analysis, Literature review, Data curation and Writing – original draft; S. S.: Conceptualization, Methodology, Formal analysis, Literature review, Data curation and Writing – original draft; S. Da.*: Conceptualization, Methodology, Formal analysis, Supervision, Resources, Software, Validation, Visualization, Writing – original draft and Writing – review and editing; S. Du.: Software, Visualization and Writing – review and editing; M. R. C.: Conceptualization, Methodology, Resources, Visualization and Writing – review and editing; A. G.: Data curation, Validation and Writing – review and editing; B. B.: Supervision and Writing – review and editing; K. B.: Writing – review and editing; B. M.: Writing – review and editing; K. B.: Writing – review and editing; D. G.: Writing – review and editing; D. P.: Writing – review and editing;
Financial support
The work did not receive any external funding.
Competing interest
The authors declare that they have no known competing financial interests or personal relationships that could have appeared to influence the work reported in this paper.
Ethics statement
This manuscript does not include human or animal research. All authors have read and approved the final manuscript.
Comments
15 October 2023
To,
The Editor(s)-in-chief,
Cambridge Prisms: Water
Prof. Fenner / Savic,
I would like to submit the manuscript entitled “Water Scarcity: A global hindrance to sustainable development and agricultural production - Impacts and Adaptation strategies” by Auindrila Biswas, Sourakanti Sarkar, Suman Dutta, Anmol Giri, Bimal Bera, Koushik Bag, Bishal Mukherjee, Koushik Banerjee, Debaditya Gupta, Debasish Paul, Malini Roy Choudhury, and Sumanta Das, to be considered for publication as an ‘Overview Review’ in the journal “Cambridge Prisms: Water”.
Manuscript at a glance:
Water is essential for sustaining life and required for carrying out basic daily activities. Even though water covers the vast majority of the earth’s surface, the availability of fresh water, which is necessary to maintain human activities is limited, making it a scarce resource. Climate change, overexploitation of groundwater, and population growth are all putting significant pressure on natural water sources, which pose a serious threat to various sectors of society, especially in agriculture. Future projections of freshwater availability indicate agriculture production will suffer a significant shock globally, including in India, leading to a threat to food security and sustainability. To ensure the sustainability of this vital resource, it is crucial to use water sensibly. Moreover, it is essential to adopt certain strategies to manage agricultural water use effectively. This includes adopting various water-efficient techniques such as ‘micro-irrigation’, ‘irrigation scheduling’, ‘conservation agriculture’, ‘crop switching’ etc. In this review, firstly, we discuss water scarcity and its types, causes, crisis for water shortages, and hindrance to sustainable development from a global perspective emphasizing the Indian scenario. Secondly, we elaborated our discussion on water scarcity in agriculture including the impacts of water scarcity on agricultural production and its connection to climate change, population growth, and overexploitation of natural resources globally focusing on the Indian scenario. In addition, water management practices and adaptation strategies to manage agricultural water use, constraints, and the need for further research are also covered. It is anticipated that this review will benefit researchers and policymakers by providing useful information on the impacts of water limitation in agriculture and adoption strategies.
We declare that this manuscript is original, has not been published previously, and/or is not being considered for publication elsewhere. We also declare that there are no conflicts of interest associated with this publication. As the corresponding author, I confirm that the manuscript has been read and approved for submission by all the authors as stated above.
We hope you will find our manuscript suitable for publication in your journal.
Thank you in anticipation.
Sincerely,
Sumanta Das, Ph.D.