Introduction
Glioblastoma (GBM) is the most common primary malignant brain tumor in adults, and also carries the most dire prognosis, with a median survival of just over 14 months with current therapy.Reference Omuro and DeAngelis1,Reference Stupp, Mason and van den Bent2 Treatment typically consists of maximal safe surgical resection, followed by fractionated radiation to a total dose of 60 Gy in 30 fractions with concomitant temozolomide.Reference Stupp, Mason and van den Bent2 During treatment and thereafter, patients are followed closely with frequent magnetic resonance imaging (MRI) scans to assess for disease progression. Imaging evidence of recurrence typically dictates a change in therapy, whether with repeat surgery or additional drug therapy, such as with bevacizumab.Reference Omuro and DeAngelis1 However, treatment-related changes due to radiation and chemotherapy frequently mimic true disease progression, with increased T2 signal change and enhancement on MRI (Figure 1). This change can be transient and occurs early following the completion of therapy (so-called “pseudoprogression”), or take the form of delayed radiation necrosis that may occur many months after completion of radiation (these nonprogressive changes will be subsequently collectively referred to as “treatment effect” or “treatment-related changes”).Reference Wen, Macdonald and Reardon3–Reference Hygino da Cruz, Rodriguez, Domingues, Gasparetto and Sorensen5
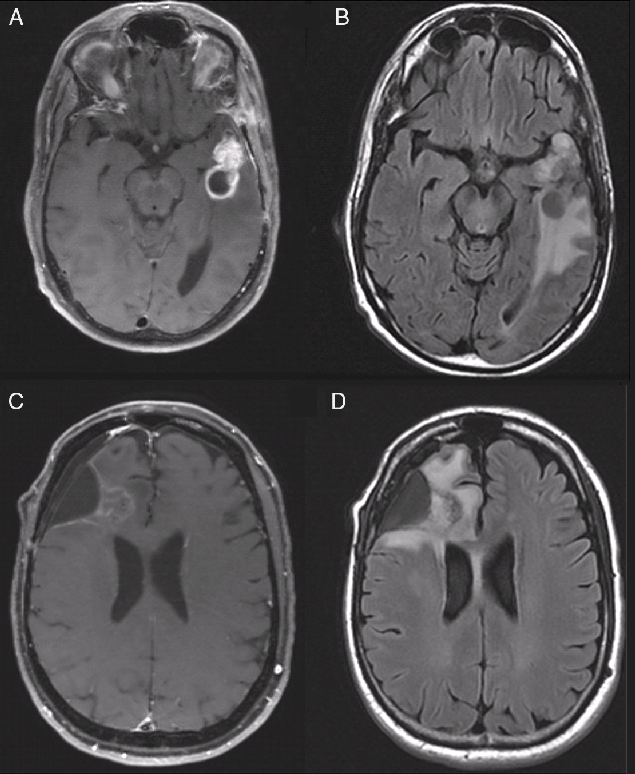
Figure 1: Representative images of treatment effect versus radiation necrosis in recurrent glioblastoma. (A and B) T1-weight post-contrast (A) and FLAIR (B) images of an asymptomatic 57-year-old man with progressive disease 8 months after completion of radiation. (C and D) T1 with contrast (C) and FLAIR (D) images of a, asymptomatic 52-year-old man more than a year after completion of radiation; pathology demonstrated only treatment-related changes. Images are from patients included in this series.
A number of strategies have been put forth to evaluate MRI imaging to differentiate treatment effect from true disease progression. The MacDonald, Response Assessment in Neuro-Oncology (RANO) and Response Evaluation Criteria in Solid Tumors (RECIST) criteria have been employed with varying reported degrees of success.Reference Wen, Macdonald and Reardon3,Reference Eisenhauer, Therasse and Bogaerts6,Reference Macdonald, Cascino, Schold and Cairncross7 However, such systems are widely acknowledged to have significant flaws, and the need for improved diagnostic techniques and assessment of factors correlated with pseudoprogression remains critical.Reference Ellingson, Chung, Pope, Boxerman and Kaufmann8 Of note, many series report rates of pseudoprogression or radiation necrosis diagnosis based purely on radiographic evidence, with patient survival frequently used as a retrospective surrogate for diagnosis in some series.Reference Yun, Park and Kim9 This has led to some speculation that treatment-related changes may be correlated with longer overall survival (OS) in GBM patients – usually without supporting pathologic evidence.Reference Hygino da Cruz, Rodriguez, Domingues, Gasparetto and Sorensen5,Reference Brandsma, Stalpers, Taal, Sminia and van den Bent10 In contrast, others have reported that histopathology distinguishing recurrent tumor from radiation necrosis at resection for GBM recurrence has no prognostic value.Reference Tihan, Barletta, Parney, Lamborn, Sneed and Chang11
In an effort to better characterize the clinical, radiographic, and treatment history of patients who demonstrate treatment-related changes during follow-up imaging, we sought to assess patients with pathology-proven evidence of treatment-related changes. To that end, we reviewed all patients at a single institution undergoing surgery (either biopsy or resection) for a presumed first recurrence of GBM.
Methods
Institutional Review Board (IRB) approval was obtained prior to initiation of the study (IRB #15-009656). A departmentally maintained database of operative case logs at a single institution was reviewed for patients with a primary diagnosis of GBM who underwent surgical resection or biopsy for a presumed first recurrence between 2005 and 2015. Pathology records were reviewed to confirm a primary diagnosis of GBM and at recurrence for a diagnosis of recurrent GBM or treatment-related changes. In cases where the pathology report at recurrence was ambiguous, clinical records were reviewed to determine whether patients were treated as a true recurrence or pseudoprogression and categorized accordingly. All pathology reports were reviewed by a neuropathologist at our institution (AR) in order to ensure consistency in categorization, with this review providing definitive categorization. Clinical notes were reviewed to confirm tumor location, recurrence location, and clinical symptoms.
In cases where radiation plans were available (either for patients receiving radiation at our institution or for whom detailed radiation planning records were imported (53 of 123 patients), plans were reviewed for volume treated, maximum total dose, maximum point dose, and volumes receiving >105% of the prescribed dose. The type of radiation plan (3D conformal vs. intensity-modulated radiotherapy (IMRT)) was also recorded.
Statistical analysis was performed using GraphPad Prism8, Microsoft Excel 2010, and MedCalc. Kaplan-Meier curves were generated for comparing progression and OS rates, with surviving patients or those lost to follow-up censored, and time-to-event significance assessed via the Gehan–Breslow–Wilcoxon test, with p-values below 0.05 considered significant. Univariate analysis using odds ratios (ORs) was used to assess differences between treatment effect and true progression groups, with p-values below 0.05 reported as significant. Continuous variables reported as means were assessed via Student’s t-test with p-values below 0.05 reported as significant.
Results
Clinical Characteristics of Treatment-Related Changes
Over a ten-year period (2005–2015) at a single institution, 123 cases of patients undergoing resection or biopsy for a presumed first recurrence of GBM were identified. Median age was 55 (21–78) in both groups and did not differ significantly between the two groups (age 55 in progression vs. 56 in treatment effect, p = 0.48, Student’s t-test). There were 35 female patients in the progression group (35%) versus 8 patients in the treatment-effect group (40%, p = 0.616, Fisher’s Exact Test). One-hundred-sixteen patients (94%) underwent resection and 7 (6%) underwent biopsy. Twenty patients (16%) demonstrated treatment-related changes on final pathology (data summarized in Table 1).
Table 1: Demographic data

Bold indicates p<0.05
Patients more commonly underwent biopsy at recurrence in the treatment-effect group (3/20 cases, 15%) versus those with true disease progression (4/103 cases, 4%); however, this trend did not reach statistical significance (OR 4.37, 95% CI 0.89–21.28, p = 0.068, Table 2). A symptomatic presentation at recurrence was not associated with a specific diagnosis (OR 1.36, 95% CI 0.51–3.61, p = 0.535). However, preoperative steroid use (OR 3.9, 95% CI 1.07–14.16, p = 0.0.038), or repeat surgery within 90 days following completion of radiation therapy, was associated with treatment-related changes on final pathology (OR 8.25, 95% CI 1.99–34.22, p = 0.0036). Overall time from diagnosis to first recurrence was also significantly shorter in the treatment-effect group (median survival 8.4 vs. 14.1 months, p = 0.0003, Gehan–Breslow–Wilcoxon test). Tumor location according to lobe (defined as either frontal, temporal, parietal, occipital, or multiple) was not associated with final pathologic diagnosis (Chi square 3.04, p = 0.695). O6-methylguanine-DNA methyltransferase (MGMT) promotor methylation status was available in 56/123 cases (11/20 treatment-effect cases and 45/103 true progression cases). MGMT promotor methylation was not associated with a diagnosis of treatment-related changes (OR 0.73, 95% CI 0.19–2.74, p = 0.64).
Table 2: Clinical characteristics associated with a diagnosis of treatment effect

Bold indicates p<0.05
* Results reported for 56 of 123 patients.
The RANO criteria for progressive disease consist of any of the following: an at least 25% increase in the area of T1 gadolinium enhancement, significant new T2 or fluid-attenuated inversion recovery (FLAIR) signal, the presence of a new lesion, or clinical decline. Of the 20 patients with pathologic evidence of treatment effect, 100% met at least one criterion for progressive disease as follows: significant T2/FLAIR signal increase (15/20 cases), clinical decline (12/20 cases), and increased enhancement (11/20 cases). A single criterion was met in 7 of 20 (35%) cases while no patient met all criteria. A new lesion was detected in 3 of 20 cases. These data are summarized in Table 3.
Table 3: RANO criteria in treatment effect cases

Survival Analysis
Patients with treatment-related changes on final pathology demonstrated prolonged median OS (from the time of surgery for presumed recurrence) relative to patients with true disease progression (16.5 vs. 10.3 months, p = 0.026, 16 of 132 cases censored due to ongoing survival or loss to follow-up Figure 2A). Median progression-free survival (PFS) was also longer in the treatment-effect group (6.5 vs. 4.3 months, p = 0.017, 16 of 132 cases censored Figure 2B). However, when measured from the time of original disease diagnosis, statistical significance was lost, with median OS of 28.7 versus 28.1 months in the treatment effect versus true progression groups, respectively (p = 0.97, 16 of 132 cases censored Figure 2C).

Figure 2: Survival analysis in recurrent glioblastoma. (A) Overall survival from the time of repeat resection for presumed GBM recurrence. (B) Progression-free survival from the time of repeat resection as defined by subsequent radiographic progression. (C) Overall survival from time of initial GBM diagnosis. In all instances, blue lines represent patients with treatment-related changes on pathology, red lines represent true disease progression. Tables below each figure indicate the number of patients at risk at each given timepoint. p-values reported from Gehan–Breslow–Wilcoxon test.
Radiation Planning
Fifty-three patients in the series underwent radiation therapy at our institution or had radiation planning records imported into our institution’s planning software. The median radiation dose was 60 Gy in 30 fractions (range 39–76 Gy). The area of presumed recurrence occurred within the planned treatment volume (PTV) in all but two cases, one diagnosed as true progression and one as radiation necrosis. Detailed volumetric data were not able to be extracted in all cases, but where possible, we evaluated the conformity of the treatment plan. The mean target volume receiving 105% of the prescribed dose (50.4cc vs. 16.2cc, p = 0.013) and the average maximum dose (69.9 vs. 64.2 Gy, p = 0.008) were significantly higher in patients with treatment-related changes on final pathology (data available for 7/20 treatment-effect cases for 105% of prescribed dose and 9/20 for maximum point dose, with data available for 39/103 true progression cases in both cases). The volume of tissue receiving prescription dose was not associated with prediction of treatment effect (177cc vs. 193.4cc in cases of true progression, p = 0.689). Patients treated with IMRT had a higher rate of treatment-related changes than those treated with 3D conformal plans (OR 7.25, 95% CI 1.32–39.5, p = 0.022). However, these patients were more likely to have a volume of 105% prescription dose and a higher maximum point dose than patients receiving 3D conformal plans (40.5 vs. 9.1cc, p = 0.001 and 68 vs. 63.4 Gy, p = 0.007, respectively). A number of patients with IMRT plans were also enrolled in dose-escalation studies where the total prescribed dose could be as high as 76 Gy. These data are summarized in Table 4.
Table 4: Radiotherapy planning factors*

Bold indicates p<0.05
* Values reported as mean (standard deviation).
Discussion
Here we describe a series of patients undergoing resection for a presumed first recurrence of GBM. We sought to assess the rate of pathology-proven treatment effect among patients that proceed with repeat surgery and to associate these findings with treatment-related variables. Many series on this subject report rates of pseudoprogression based solely on radiographic and clinical observation, rather than tissue diagnoses, which limits the assessment of patient’s actual disease rate.Reference Yun, Park and Kim9,Reference Kucharczyk, Parpia, Whitton and Greenspoon12 In our series, the rate of treatment-related changes on final pathology was 16%, similar to that reported in several other series.Reference Ellingson, Chung, Pope, Boxerman and Kaufmann8,Reference Kucharczyk, Parpia, Whitton and Greenspoon12,Reference Chamberlain, Glantz, Chalmers, Van Horn and Sloan13 Prior studies have also reported a higher rate of treatment-related changes in cases close to the completion of radiation, with one study reporting over 50% of patients undergoing repeat surgery within six months of completion of radiation demonstrating radiation necrosis.Reference Chamberlain, Glantz, Chalmers, Van Horn and Sloan13 The true rate of treatment-related changes masquerading as progression remains unclear; however, as most series on the subject are small and use varied criteria for defining treatment-related changes.Reference Ellingson, Wen, van den Bent and Cloughesy14 Furthermore, many patients with new enhancement on MRI after radiation and temozolomide will not have surgery to obtain tissue prior to making treatment decisions. Therefore, we anticipate that the actual rate of treatment-related changes is likely substantially higher than commonly reported and likely higher than we report here, as we only identified patients who underwent a second surgery.
While many patients without pathologic evidence of disease progression were symptomatic on presentation (55%) and may have benefited from a repeat surgical debulking regardless of the underlying pathology, our findings underscore the difficulty in distinguishing true progression from treatment effects based on radiographic and clinical criteria alone. Challenges in developing criteria for GBM progression based on imaging are widely reported, and multiple rubrics to assess disease progression have been put forth, including the MacDonald, RANO, and RECIST criteria.Reference Wen, Macdonald and Reardon3,Reference Eisenhauer, Therasse and Bogaerts6,Reference Macdonald, Cascino, Schold and Cairncross7 Our hope in reviewing cases with pathologic diagnosis is to reduce ambiguity and potentially explore additional points of information that may provide valuable insight into the likelihood of pseudoprogression.
To that end we closely reviewed available dose–volume histograms of radiation treatment plans for a subset of cases. We observed that heterogeneous plans with large volumes exceeding prescription dose were more likely to have treatment-related changes than plans that were more homogenous. The volume of tissue receiving doses greater than the prescription dose was a more important predictor of treatment effect than the total target volume receiving prescription dose. Further, only two patients were observed to have treatment-effect changes outside of the high dose target volume, indicating a dependency of radiation dose and volume on the development of pseudoprogression.
It has been suggested that pseudoprogression or treatment-related changes may correlate with increased survival in GBM.Reference Hygino da Cruz, Rodriguez, Domingues, Gasparetto and Sorensen5,Reference Brandsma, Stalpers, Taal, Sminia and van den Bent10,Reference Sanghera, Perry and Sahgal15 Indeed, when taken from the time of surgery for presumed recurrence, patients demonstrating treatment-related changes on pathology have longer overall and PFS times (Figure 2A and B). However, when the time of initial diagnosis of GBM is taken into account, this benefit vanishes, consistent with the finding that patients with pseudoprogression tend to have presumed recurrence closer to the completion of radiation, reported by others and confirmed in our series.Reference Chamberlain, Glantz, Chalmers, Van Horn and Sloan13,Reference Sanghera, Perry and Sahgal15 That treatment effect does not appear to confer a survival benefit is important clinically, as this indicates that, where possible, measures should be taken to minimize the risk of treatment-related imaging changes in order to reduce the difficulty in assessing postoperative imaging, and in order to reduce the risk of unnecessary surgical interventions.
These findings underscore the need to delve further into the development of improved techniques for determining the likelihood of treatment-related changes. Efforts have been made to use MRI perfusion studies looking at increased relative cerebral blood volumes (rCBV) as a surrogate marker for disease progression with varying levels of efficacy.Reference Kong, Kim and Kim16–Reference Young, Gupta and Shah18 This technique was used intermittently in our series, but was not used as a major factor in determining whether patients had disease progression. Positron-emission tomography (PET) studies have also been used to determine the likelihood of treatment effect, but significant logistical and cost constraints prevent this from being widely used.Reference Galldiks, Dunkl and Stoffels19
This study is limited by its relatively small size, the retrospective nature of the review, and by variability in the imaging modalities and criteria applied to each patient in the preoperative determination of likely disease progression. Further, a number of patients in this cohort remained censored in the survival analysis (16 total, 6 in the treatment-effect group with regard to OS since recurrence). The inability to follow all patients until mortality could potentially mask a survival benefit in the treatment-effect group. Additionally, while the express purpose of our study was to examine the rate of treatment-related changes in patients who proceed with repeat surgery, the generalizability to all patients with a primary diagnosis of GBM may be limited due to selection bias inherent in including patients that were healthy enough to undergo repeat surgery.
The need for improved characterization of postoperative imaging will only increase as the field moves forward, with the development of novel therapeutics including tumor vaccines and other immune-mediated approaches (several patients in our study ultimately received such therapies, but not prior to the first recurrence discussed here). Attempts have been made to take these treatments into account when assessing progression in the form of the iRANO criteria.Reference Okada, Weller and Huang20 Such treatments are frequently associated with significant radiographic treatment-related changes, which can make assessment of a patient’s disease status incredibly difficult, especially in the context of assessing an investigational therapy, and ongoing efforts are necessary to better characterize this process and determine its implications for the clinical course of patients.
Conflict of Interest
The authors have no conflicts of interest to disclose.
Statement of Authorship
All authors contributed meaningfully to the development of this study and manuscript. Conception and design – BTH, AA, KWM, IFP. Data collection – BTH, AA, KWM, MG, AGB. Data analysis – BTH. Review of pathology findings – AR. Interpretation of data – all authors. Drafting and critical revision of manuscript – all authors.
Ethical Standards
IRB approval was received prior to initiation of the study (IRB #15-009656). This study is a retrospective review requiring review of the medical record only.