Introduction
Since the late 19th century populations of large carnivores have been suppressed or extirpated throughout their geographical ranges by anthropogenic mortality (Linnell et al., Reference Linnell, Swenson and Anderson2001; Cardillo et al., Reference Cardillo, Purvis, Sechrest, Gittleman, Bielby and Mace2004; Ripple et al., Reference Ripple, Estes, Beschta, Wilmers, Ritchie and Hebblewhite2014). Large carnivores may be at greater risk of extinction globally compared to other groups of mammals because of inherent ecological characteristics such as large body size, reliance on large prey, social organization and restricted geographical ranges (Purvis et al., Reference Purvis, Gittleman, Cowlishaw and Mace2000; Cardillo et al., Reference Cardillo, Purvis, Sechrest, Gittleman, Bielby and Mace2004). Although large carnivores share similar ecological characteristics that may put them at risk, factors that affect carnivore populations at local and regional scales can vary significantly (Purvis et al., Reference Purvis, Gittleman, Cowlishaw and Mace2000; Ferguson & Larivière, Reference Ferguson and Larivière2002). As a result, conservation strategies developed to reduce anthropogenic pressure on populations of large carnivores vary among regions, reflecting diverse challenges to protecting and enhancing threatened populations; for example, in South Africa conservation fences are used to protect lions Panthera leo from persecution and anthropogenic habitat degradation (Hayward et al., Reference Hayward, Hayward, Druce and Kerley2009), and in the United States, Texas cougars Puma concolor were introduced into south-west Florida to assist in genetic restoration of the endangered Florida panther Puma concolor coryi population (Hedrick, Reference Hedrick1995; Johnson et al., Reference Johnson, Onorato, Roelke, Land, Cunningham and Belden2010). These examples demonstrate the importance of developing unique conservation strategies to address key threats to large carnivores.
In the eastern United States proactive conservation actions were necessary to prevent extinction of the Critically Endangered red wolf Canis rufus (Kelly et al., Reference Kelly, Beyer and Phillips2008; IUCN, 2014), which had been reduced to a single small, isolated population as a result of predator-control programmes and excessive hunting. The last known red wolf population was intentionally extirpated from the wild in the 1970s by the United States Fish and Wildlife Service (USFWS) when recovery in situ was deemed unlikely because of persecution, disease, poor habitat and hybridization with coyotes Canis latrans (USFWS, 1990; Hinton et al., Reference Hinton, Chamberlain and Rabon2013). During the late 1980s red wolves were reintroduced into eastern North Carolina, and the population currently comprises c. 100 individuals (USFWS, 2014). This represents the first successful reintroduction of a large predator species that was completely extirpated from the wild. Restoration of the red wolf also served as a model for subsequent reintroductions of the grey wolf Canis lupus to the Southwest and Greater Yellowstone Ecosystem (Phillips et al., Reference Phillips, Henry, Kelly, Mech and Boitani2003).
Red wolf recovery efforts in eastern North Carolina have occurred in the presence of two factors that facilitated the species’ decline and extirpation from the wild during the late 20th century: hybridization with coyotes and anthropogenic mortality. After several hybridization events occurred during the mid 1990s a population and habitat viability assessment was conducted to re-evaluate long-term management of red wolves and determine how to monitor hybridization effectively and limit it to acceptable levels (Kelly et al., Reference Kelly, Miller and Seal1999). A unique approach to limit hybridization was implemented whereby coyotes and hybrids were sterilized and used as placeholders on the landscape to suppress coyote reproduction and prevent coyotes that consort with red wolves from producing hybrids (Hinton et al., Reference Hinton, Chamberlain and Rabon2013; Gese & Terletzky, Reference Gese and Terletzky2014). By capturing, sterilizing and then releasing coyotes and hybrids back into the wild, the USFWS's Red Wolf Recovery Program (hereafter Recovery Program) could manipulate canid breeding pairs of non-red wolves to suppress hybridization and introgression.
Although sterilization has been effective at limiting hybridization and introgression to below 4% in the wild red wolf population (Gese et al., Reference Gese, Knowlton, Adams, Beck, Fuller and Murray2015), management strategies for the wild population were not designed to address excessive anthropogenic mortality, and no social scientists were assigned to the Red Wolf Implementation Team because social and public relations issues were left to non-profit organizations (the Red Wolf Coalition) and the USFWS to address (Stoskopf et al., Reference Stoskopf, Beck, Fazio, Fuller, Gese and Kelly2005). Red wolf deaths caused by gunshot have increased c. 275% since 2004, with most killings occurring during the North Carolina autumn and winter hunting seasons (Bartel & Rabon, Reference Bartel, Rabon and Soorae2013). Anthropogenic mortalities complicate management of Canis breeding units by disrupting resident breeding pairs and facilitating high turnover of territories; research suggests that anthropogenic mortality of both red wolves and eastern wolves Canis lycaon facilitates hybridization with coyotes by disrupting the population's social structure (Rutledge et al., Reference Rutledge, White, Row and Patterson2012; Bohling & Waits, Reference Bohling and Waits2015). Anthropogenic mortality was also found to have a negative effect on the annual preservation of red wolf breeding pairs (Sparkman et al., Reference Sparkman, Waits and Murray2011). Bohling & Waits (Reference Bohling and Waits2015) assessed 30 hybridization events between red wolves and coyotes, and reported that red wolves involved in hybridization were displaced from packs disrupted by anthropogenic mortality among the breeders. To further assess the effects of anthropogenic mortality on red wolf breeding units and successful management of red wolves, we evaluated the management and fate of Canis breeding pairs during a 22-year period. Specifically, we identified the primary causes of disbandment and described how anthropogenic mortality disrupts breeding units and territorial behaviour, and hinders conservation strategies designed to facilitate red wolf recovery. Our primary objective was to identify processes responsible for disbandment of red wolf breeding pairs and sterile placeholders. We also investigated if red wolves were replacing lost wolf breeders and sterile placeholders on the landscape following disbandment caused by natural and anthropogenic factors. Our study provides insight into causes of disbandment of red wolf breeding pairs, the subsequent formation of new breeding pairs, and the relationship between anthropogenic mortality and hybridization between red wolves and coyotes.
Study area
The Red Wolf Recovery Area (hereafter Recovery Area) consists of five counties (Beaufort, Dare, Hyde, Tyrrell and Washington) on the Albemarle Peninsula of eastern North Carolina (Fig. 1). The study area encompasses c. 6,000 km2 of federal, state and private lands consisting primarily of an agricultural–coastal bottomland matrix. Agricultural crops (i.e. corn, cotton, soybean and winter wheat) and managed pine Pinus spp. account for c. 30 and 15% of the land cover, respectively, and coastal bottomland forests and pocosin habitats account for c. 35%. The climate is typical of the mid-Atlantic: four distinct seasons, nearly equal in length, with annual precipitation of 1,220–1,320 mm. Summers are typically hot and humid, with daily temperatures of 27–38°C, and winters are relatively cool, with daily temperatures of −4–7°C.

Fig. 1 The location of the Red Wolf Recovery Area in North Carolina, USA.
Methods
Field data collection
As part of the Recovery Program's long-term monitoring and management, trapping was carried out annually during autumn and winter to capture red wolves, coyotes and hybrids, and fit them with mortality-sensitive VHF radio collars (Teleonics, Mesa, USA). Collared individuals were monitored twice per week from aircraft for the duration of their life. The canids were captured using padded foot-hold traps (Victor no.3 Softcatch, Woodstream Corporation, Lititz, USA), and coyotes and hybrids were taken to a local veterinary clinic and surgically sterilized by vasectomies and tubal ligations (Gese & Terletzky, Reference Gese and Terletzky2014). This process keeps hormonal systems intact to avoid disrupting breeding and territorial behaviour (Seidler & Gese, Reference Seidler and Gese2012).
Resident breeding pairs were identified as radio-collared individuals of breeding age (≥ 2 years old) who were temporally and spatially associated with one another and had been defending a territory for ≥ 6 months. Biologists confirmed breeding pair status during spring den work (March–May) by locating dens and daybeds of females to verify the presence of litters for each radio-collared breeding pair during 1991–1999. After 1999, dens were investigated more thoroughly; biologists sampled blood from pups each spring to verify parentage and construct a red wolf pedigree (Miller et al., Reference Miller, Adams and Waits2003; Beck et al., Reference Beck, Lucash and Stoskopf2009; Brzeski et al., Reference Brzeski, Rabon, Chamberlain, Waits and Taylor2014). We used the red wolf population pedigree in combination with a complete set of chronological and geographical data of Canis breeding pairs in the Recovery Area to identify when breeding pairs formed and disbanded. Red wolves paired with a sterilized non-wolf mate were confirmed as congeneric breeding pairs through field data and occasionally by den work if the non-wolf mate had not been sterilized. We defined two types of breeding pairs: red wolf (two red wolves) and congeneric (red wolf with a coyote or a hybrid).
Causes of breeding pair break-ups were categorized as natural causes (i.e. disease, intraspecific strife, malnutrition), management actions (i.e. removal of coyote or hybrid from congeneric pairs), anthropogenic (i.e. poison, trapping, suspected or confirmed gunshot, vehicle collision), or unknown. We separated management actions from anthropogenic causes because break-ups of breeding pairs through management are fundamentally different from break-ups caused by other human activities, such as illegal and incidental killing. Attempts to remove coyotes from congeneric pairs represent efforts to free individual red wolves as potential mates for dispersing wolves and can be eliminated by cessation of management protocols, whereas illegal and incidental killing are indiscriminate and more difficult to eliminate. Causes of mortalities were categorized as unknown in several situations: (1) there was not enough biomaterial present to determine the cause of death (e.g. skeletal remains, hair mat only), (2) necropsy analyses were inconclusive, or (3) multiple causes of death were suspected in pending cases and were confirmed by necropsy. Mortalities caused by management-related activities included deaths resulting from capture and handling and reactions to medical treatments. Mortalities caused by gunshot included cases where foul play was suspected (e.g. a cut or removed radio collar, bullet wounds), and confirmed cases where x-rays or necropsy examinations yielded evidence of bullet fragments. Instances of poisoning were confirmed by necropsy and toxicological analysis.
Data analyses
We divided the study period into three phases: I (1991–1998), II (1999–2005) and III (2006–2013). Phase I represents when the USFWS originally focused efforts to establish a wild red wolf population (Phillips et al., Reference Phillips, Henry, Kelly, Mech and Boitani2003). Phase II represents when the USFWS adopted and implemented an adaptive management plan to detect, monitor and manage hybridization as the Recovery Area was colonized by coyotes (Stoskopf et al., Reference Stoskopf, Beck, Fazio, Fuller, Gese and Kelly2005). Phase III represents when the USFWS reported stagnant population growth and increased rates of red wolf mortality as a result of anthropogenic causes (USFWS, 2007; Bartel & Rabon, Reference Bartel, Rabon and Soorae2013).
We used trapping data to estimate relative abundances of red wolves, coyotes and their hybrids and to evaluate their ratio before and after breeding pairs disbanded, by calculating the number of red wolves, coyotes and hybrids captured each year. We analysed 22 years (1991–2013) of trapping data compiled by the Recovery Program, to calculate annual ratios of red wolf to coyote and red wolf to hybrid. Most trapping was conducted in known and suspected territories of red wolf breeding pairs, to capture and fit pups with radio collars or to target coyotes or hybrids suspected of pair-bonding with red wolves. However, trapping also focused on capturing resident coyotes and hybrids to use as placeholders and, to a lesser extent, to address complaints from the public regarding the presence of red wolves and coyotes on private lands. Recovery Program biologists also coordinated with private fur trappers to opportunistically capture red wolves, coyotes and hybrids throughout the Albemarle Peninsula. Although trapping was used to accomplish multiple objectives and could not be standardized temporally or spatially, we believe trapping efforts that were part of the large-scale, long-term monitoring efforts conducted across the five-county Recovery Area provided the only reasonable proxy for abundances of Canis species in eastern North Carolina (Keane et al., Reference Keane, Jones and Milner-Gulland2011; Stephens et al., Reference Stephens, Pettorelli, Barlow, Whittingham and Cadotte2015). We used ANOVA to determine differences in the numbers of red wolves, coyotes and hybrids captured during each of the three phases and to determine variations in ratios of red wolf to coyote and red wolf to hybrid during each of the three phases. We used linear regression to assess if these ratios were correlated with the percentage of breeders lost to anthropogenic mortality.
We calculated several metrics of pair preservation, replacement and disbandment to evaluate how Canis breeding pairs changed during each phase. Because we categorized canids that were temporally and spatially associated with a potential mate for ≥ 6 months as breeding pairs, we calculated duration of Canis breeding pairs in half-year increments. The annual preservation rate of Canis breeding pairs was calculated as the number of Canis breeding pairs preserved (maintained from the previous year) divided by the total number of Canis breeding pairs present in a given year. We calculated annual replacement rates of red wolves as the number of Canis breeding pairs disbanded (not maintained from the previous year) and replaced by red wolves divided by the total number of Canis breeding pairs disbanded for each given year. We calculated annual disbandment rate as the number of Canis breeding pairs disbanded as a result of anthropogenic causes divided by the total number of Canis breeding pairs disbanded in a given year. Because annual preservation replacement and displacement rates were reported as proportion data, we logit transformed these variables for analyses (Warton & Hui, Reference Warton and Hui2011).
We used linear regression to assess increasing or decreasing trends in annual preservation replacement and displacement rates of Canis breeding pairs. We also used ANOVA to determine variation among these rates across the three phases. We described the primary causes of break-up of Canis breeding pairs using contingency tables to compare observed frequencies of breeding pair disbandment to expected frequencies for each of the four primary causes of disbandment. We also used contingency tables to determine if disbanded Canis breeding pairs were replaced more often than expected by a red wolf or congeneric breeding pair. Similarly, we assessed whether the mate of a disbanded breeding pair accepted a red wolf or coyote as a new mate more often than expected. We defined acceptance of a new mate as when individual canids from disbanded breeding pairs were observed to be temporally and spatially associated with a new canid for ≥ 6 months.
Results
During 1991–2013 1,144 canids were captured: 510 red wolves (44.6%), 416 coyotes (36.4%) and 218 genetically identified hybrids (19.0%). Captures of red wolves did not vary across the three phases (Table 1; F 2,20 = 2.58, P = 0.101), whereas coyote captures were highest during Phase III (F 2,20 = 29.50, P < 0.001) and hybrid captures were lowest during Phase I (Table 1; F 2,20 = 10.60, P < 0.001). Ratios of red wolf to coyote declined from Phase I to Phase III (F 2,20 = 11.90, P < 0.001) but were similar between Phase I and Phase II, whereas ratios of red wolf to hybrid were similar between Phase II and III but significantly higher during Phase I (Table 1; F 2,20 = 4.85, P = 0.019).
Table 1 Numbers and ratios of red wolves Canis rufus, coyotes Canis latrans and hybrids captured in eastern North Carolina during 1991–2013.

During 1991–2013 we identified 174 Canis breeding pairs: 109 red wolf (62.6%) and 65 congeneric (37.4%) pairs (Fig. 2). The percentage of Canis breeding pairs disbanded by anthropogenic mortality increased during this period (R 2 = 0.52, P > 0.001) and the annual preservation rates of Canis breeding pairs declined steadily (F 2,20 = 10.23, P = 0.004). The annual replacement rates of Canis breeding pairs by red wolves were lowest during Phase III (F 2,20 = 4.08, P = 0.033), whereas the annual rates of disbandment from anthropogenic causes were highest during Phase III (F 2,20 = 8.65, P = 0.002). Ratios of red wolf to coyote were negatively correlated with anthropogenic disbandment (Fig. 3; R 2 = 0.39, P < 0.001), and were lowest during Phase III, when breeder loss as a result of anthropogenic mortality was highest (Table 2). We observed no correlation between human-caused disbandment and observed ratios of red wolf to hybrid (R 2 = 0.001, P = 0.874).
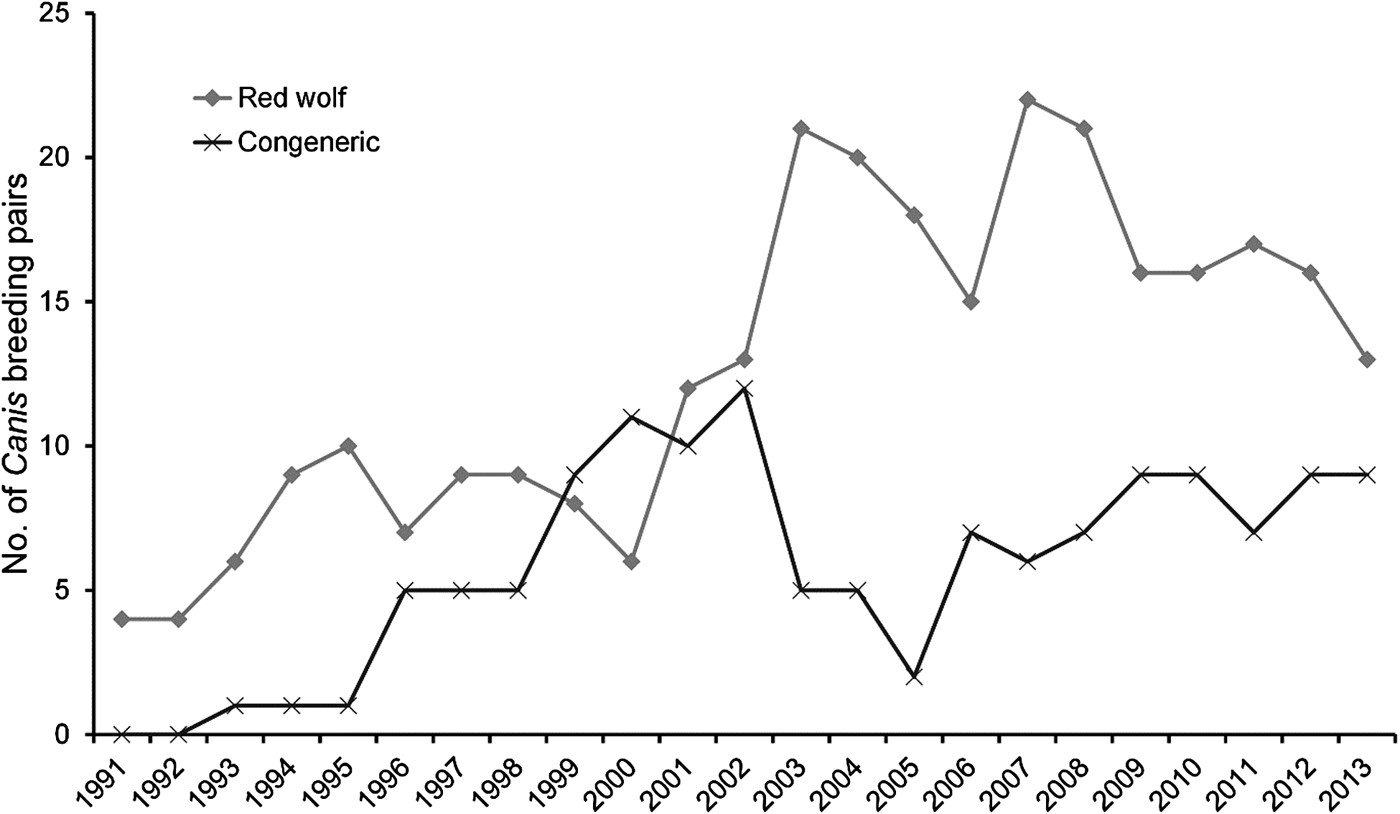
Fig. 2 Number of red wolf Canis rufus and congeneric Canis breeding pairs monitored in the Red Wolf Recovery Area (Fig. 1) during 1991–2013. In Phase I (1991–1998) the U.S. Fish and Wildlife Service focused efforts to establish a wild red wolf population, in Phase II (1999–2005) they focused efforts to detect, monitor and manage hybridization in the Recovery Area, and in Phase III (2006–2013) they reported increasing rates of red wolf mortality as a result of anthropogenic causes.
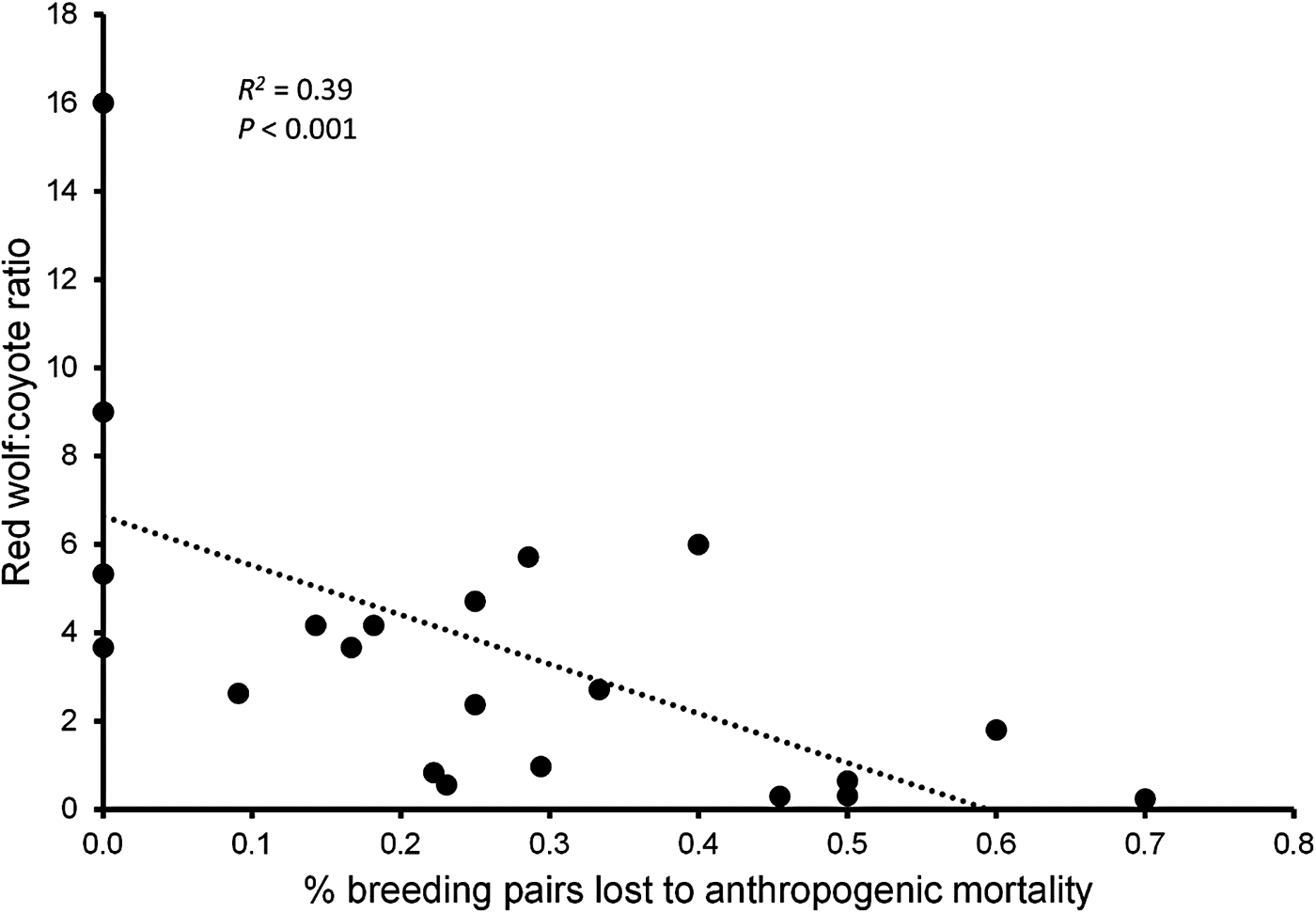
Fig. 3 Annual ratios of red wolves to coyotes Canis latrans among canids captured by the Red Wolf Recovery Program regressed against the annual percentage of Canis breeding pairs disbanded because of anthropogenic mortality in eastern North Carolina (Fig. 1) during 1991–2013.
Table 2 Cause of break-up of Canis breeding pairs, with the number of break-ups attributed to each cause, and the percentage of pairs replaced by red wolves or coyotes/hybrids, or not replaced < 12 months after breeding pairs disbanded in eastern North Carolina during 1991–2013.

Mortality, pooled across all causes, was responsible for most disbandment in Canis breeding pairs (73.6%). Primary causes of mortality responsible for disbandment of Canis breeders corresponded with those reported to affect red wolf survival (J.W. Hinton et al., unpubl. data). Anthropogenic causes accounted for 40.6% of deaths, whereas natural causes and management actions accounted for 33.6 and 25.8%, respectively. Mortalities caused by gunshots were responsible for 71.2% of disbandment as a result of anthropogenic causes, and 21.3% of all disbandment. In comparison to red wolf breeding pairs, congeneric breeding pairs were disrupted more by management actions (40.0 vs 6.4%) and less by natural (27.7 vs 51.4%) and anthropogenic causes (21.5 vs 34.9%; χ3 2 = 32.45, P < 0.001). Anthropogenic and unknown causes of Canis breeding pair break-ups were highest in Phase III (Fig. 4; χ6 2 = 28.01, P < 0.001). Assessing only red wolf breeding pairs across all Phases, anthropogenic causes of break-ups increased (15.0, 25.7 and 48.2% during Phase I, II and III, respectively; χ6 2 = 26.44, P < 0.001). Similarly, anthropogenic causes in congeneric pair disbandment increased across all Phases (0, 11.5 and 32.4% during Phase I, II and III, respectively; χ6 2 = 13.19, P = 0.040). Overall, red wolves were more likely to replace Canis breeding pairs when disbandment was caused by natural causes and management actions (Table 2; χ4 2 = 19.27, P = 0.004).
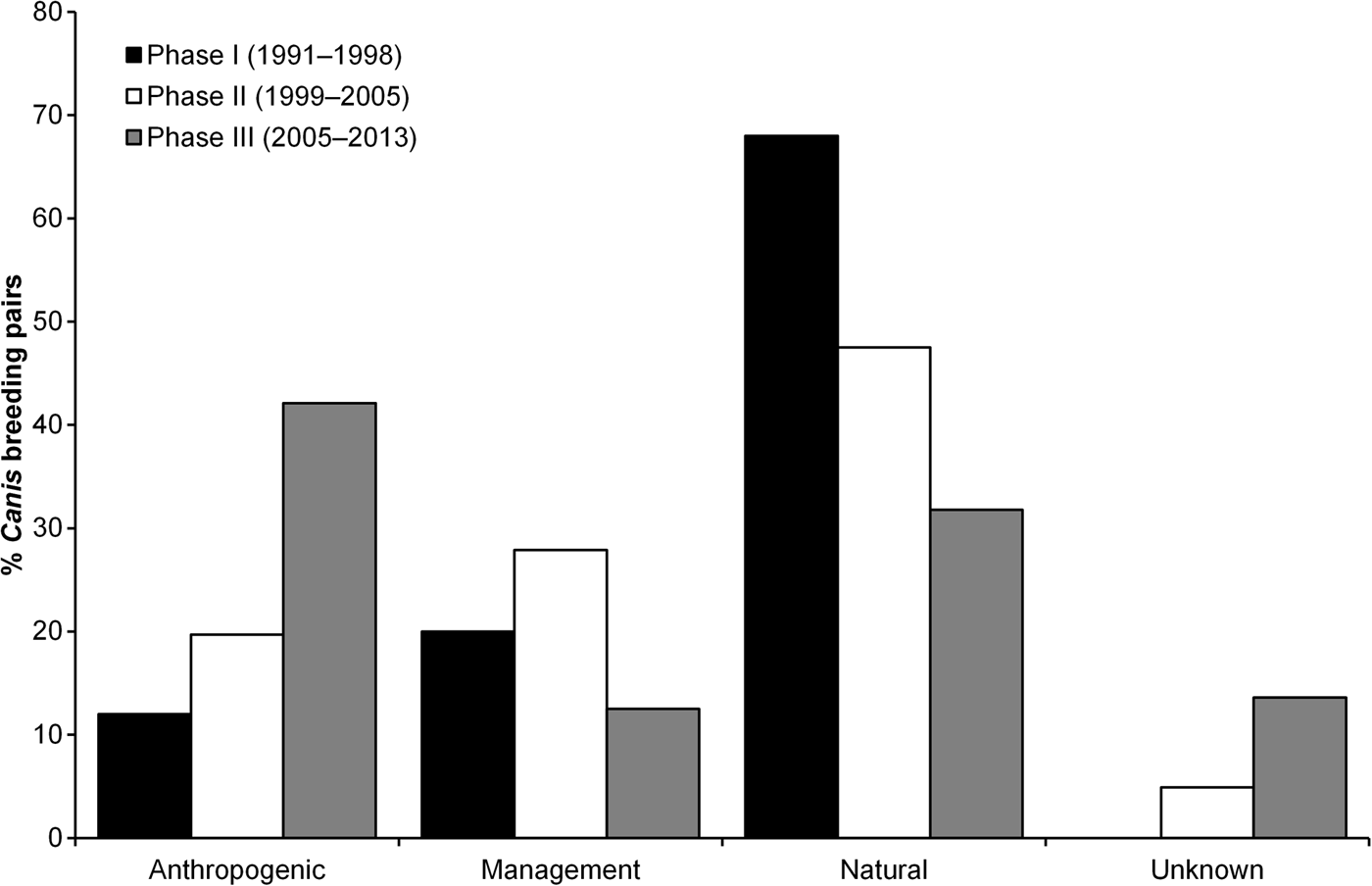
Fig. 4 Percentage of Canis breeding pairs that were disbanded as a result of anthropogenic causes, management actions, natural causes, and unknown causes in eastern North Carolina (Fig. 1) during 1991–2013. Phases represent changes in conservation strategies to mitigate environmental factors that affected red wolf recovery efforts in the wild population. The total number of Canis breeding pairs was 25 in Phase I, 61 in Phase II, and 88 in Phase III.
Overall, the percentage of red wolf breeding pairs vs congeneric breeding pairs did not change among phases (80.0, 57.4 and 61.4% for Phase I, II and III, respectively; χ2
2 = 4.31, P = 0.116). However, the percentage of congeneric breeding pairs including coyotes was highest during Phase III (40.0, 34.6 and 79.4% for Phase I, II and III, respectively; χ2
2 = 13.39, P < 0.001). Congeneric pairs typically contained more female than male red wolves (69.2 vs 30.8%; χ1
2 = 14.44, P < 0.001). There was no variation observed in the duration of red wolf breeding pairs over time (
$\bar x$
= 1.7 ± SE 0.4, 1.8 ± SE 0.3 and 2.0 ± SE 0.2 years in Phases I, II and III, respectively; F
2,102 = 0.38, P = 0.685) but the duration of congeneric breeding pairs declined steadily (
$\bar x$
= 2.3 ± SE 0.5, 1.7 ± SE 0.2 and 1.1 ± SE 0.2 years in Phases I, II and III, respectively; F
2,62 = 4.28 P = 0.018). Overall, the duration of red wolf breeding pairs was longer than that of congeneric pairs (
$\bar x$
= 1.9 ± SE 0.2 vs
$\bar x$
= 1.4 ± 0.2 years; F
1,172 = 4.84, P = 0.029).
Discussion
Early in red wolf recovery efforts, when there were fewer coyotes and anthropogenic mortalities, wolves replaced wolf breeders. However, since the early 2000s the coyote population and the number of red wolf mortalities caused by gunshots have increased, with coyotes typically replacing red wolves following deaths caused by humans, specifically those caused by gunshot. Coyote populations have increased in North Carolina (DeBow et al., Reference DeBow, Webster and Sumner1998) and the Recovery Area, which is evident from our trapping data: the number of coyote captures was highest during 2006–2013. Since the mid 2000s the North Carolina Wildlife Resources Commission has increased opportunities for coyote hunting in an attempt to reduce the abundance of coyotes. However, red wolves are often mistaken for coyotes by hunters, and, by proxy, are subject to excessive hunting pressure; this trend is evident from the fact that most gunshot mortalities occur during the autumn and winter hunting season (15 October–31 December; Bartel & Rabon, Reference Bartel, Rabon and Soorae2013). Since 1991 there has been a significant increase in the break-up of red wolf breeding pairs as a result of anthropogenic mortalities, in which gunshot mortalities were a primary reason for disbandment, prevented long-term pairings and facilitated the increase in congeneric breeding pairs observed during 2006–2013. Replacement by coyotes in red wolf breeding pairs disbanded as a result of gunshot mortalities has increased significantly since the early 2000s. This pattern has profound effects on red wolf demography and population increase because each breeding pair disbanded represents a lost reproductive opportunity. This loss is compounded by an increase in coyotes replacing disbanded pairs, further reducing the ability of surviving wolves to find conspecific mates. Similarly to red wolf breeding pairs, congeneric breeding pairs containing sterile placeholders were also disbanded at higher rates as a result of anthropogenic mortality. This trend is problematic for controlling hybridization if sterile coyotes and hybrids paired with red wolves are killed and replaced by fertile coyotes.
Rutledge et al. (Reference Rutledge, Patterson, Mills, Loveless, Murray and White2010) reported that intense harvest outside Algonquin Provincial Park disrupted pack structure and social patterns in which culling of eastern wolves during the 1960s was linked to past introgression from coyotes within the Park (Rutledge et al., Reference Rutledge, White, Row and Patterson2012). Benson et al. (Reference Benson, Patterson and Wheeldon2012) also suggested that levels of harvest in areas adjacent to Algonquin Provincial Park may influence hybridization dynamics between eastern wolves and coyotes. Similarly, Bohling & Waits (Reference Bohling and Waits2015) found that most hybridization events in the Recovery Area occurred after red wolves lost mates to gunshots, and suggested that the disruption to the wolves’ social structure and stability facilitated hybridization. Our results complement the findings of these studies and offer unique insights regarding how Canis breeding pairs are affected by the anthropogenic factors responsible for disbandment. In addition to negatively affecting red wolf survival, anthropogenic mortality disrupts pack dynamics by reducing the mean life expectancy. The mean lifespan of a red wolf in the wild is 3.2 years (J.W. Hinton et al., unpubl. data) and our findings indicate that the mean duration of red wolf breeding pairs is 2 years. The short duration of breeding pairs is a consequence of low life expectancy and prevents the long-term pairing required for developing the social structure and stability necessary to maintain pack dynamics. The average time for red wolves to replace lost mates is unknown, but selecting a new mate may occur over a period of several days to several months, as in other canids (Asa & Valdespino, Reference Asa and Valdespino1998; Bekoff & Gese, Reference Bekoff, Gese, Feldhamer, Thompson and Chapman2003; Borg et al., Reference Borg, Brainerd, Meier and Prugh2015). When resident red wolves lose mates they permit transients of the opposite sex into their territories to facilitate selection of a new mate. The excessive turnover of Canis breeding pairs resulting from anthropogenic mortality is likely to erode strong territorial behaviour in both wolves and coyotes, and increases mate-selection behaviours. This promotes coyote encroachment into areas where red wolf breeding pairs have been disbanded by anthropogenic causes, facilitating an increase in congeneric pairing between red wolves and coyotes. It may also create opportunities for coyotes to outnumber red wolves and usurp their territories.
Bohling & Waits (Reference Bohling and Waits2015) reported a strong bias of female red wolves producing hybrid litters but asserted that hybrid litters were easier to find when they involved radio-collared female wolves rather than female coyotes and hybrids without radio collars. Similarly, our results show that c. 70% of all congeneric breeding pairs included a female red wolf, and we believe the greater occurrence of female wolves producing hybrid litters reported by Bohling & Waits (Reference Bohling and Waits2015) was not a result of monitoring bias. It appears female red wolves are more likely than males to form congeneric pairs and, as red wolf pairs and packs are disrupted by excessive anthropogenic mortality, surviving female wolves pair-bond with encroaching coyotes when compatible wolf mates are unavailable. The loss of female red wolves to encroaching coyotes is problematic because it disrupts wolf natality that is necessary to buffer the effects of mortality. Natality has the greatest influence on a population's ability to increase (Vandermeer & Goldberg, Reference Vandermeer and Goldberg2004), and congeneric pairing disrupts the red wolf's potential to compensate for the loss of breeders through reproduction.
Current approaches to coyote management often focus on demographic consequences of population control methods, with little consideration of accidental or intentional killing of other Canis species. Previous hunting regulations (no closed season, artificial lights permitted, and no bag limits) increased the likelihood of red wolves being mistaken for coyotes and shot by hunters. In 2014 a court-approved settlement between several conservation groups and the North Carolina Wildlife Resources Commission banned night-time hunting of coyotes, and permitting and reporting are now required for coyote hunting during the day in the Recovery Area (Boyle, Reference Boyle2014). Regulating the take of coyotes will have considerable potential for reducing the effects of incidental killing of red wolves. Nevertheless, the USFWS is responsible for developing recovery plans to address key threats to the survival of threatened and endangered species (Hoekstra et al., Reference Hoekstra, Clark, Fagan and Boersma2002). Anthropogenic mortality and hybridization were identified as the two primary threats to red wolves, but although it has taken measures to address hybridization, the USFWS has not addressed threats of anthropogenic mortality.
Fundamental to the conservation of any imperilled species is identifying how anthropogenic factors lead to population declines. Key life histories are known to affect population viability, and in large carnivores mating and social structure have a significant influence on population dynamics (Ferguson & Larivière, Reference Ferguson and Larivière2002; Borg et al., Reference Borg, Brainerd, Meier and Prugh2015); for example, when dominant males are hunted this can increase offspring mortality as a result of sexually selected infanticide following male turnover (Milner et al, Reference Milner, Nilsen and Andreassen2007; Caro et al., Reference Caro, Young, Cauldwell and Brown2009). Conserving red wolves and other imperilled carnivores will therefore require the development of new conservation strategies that account for the potential effects of social behaviours on population dynamics, and will ultimately depend on governing organizations recognizing and responding to threats such as anthropogenic pressures that negatively affect life-history traits important to survival.
Acknowledgements
Data for this research were provided by the U.S. Fish and Wildlife Service Red Wolf Recovery Program, and the research was funded by the Warnell School of Forestry and Natural Resources at the University of Georgia. We particularly appreciate the support of the Red Wolf Recovery Program, specifically A. Beyer, M. Butts, R. Harrison, C. Lucash, F. Mauney, M. Morse and R. Nordsven. We also thank J. Benson for comments on the manuscript. The findings and conclusions in this article are those of the authors and do not necessarily represent the views of the U.S. Fish and Wildlife Service. Any use of trade, product or firm names is for descriptive purposes only and does not imply endorsement.
Biographical sketches
Joseph Hinton's research interests lie in understanding how selective pressures operating on key traits influence the abundance and distribution of wildlife populations. Kristin Brzeski's research interests include assessing associations between genetic variation and fitness, and the genetic structure of threatened species. David Rabon is a conservation biologist interested in the restoration and management of threatened species, particularly wild canids and other carnivores. Michael Chamberlain conducts research on a variety of wildlife species, with a keen focus on canids.