Milk contains numerous bioactive substances including immunoglobulins, cytokines, growth factors and components possessing antibiotic and prebiotic activity(Reference Kelleher and Lönnerdal1, Reference Field2). Little is known about the biological effects of individual milk bioactives, despite the fact that natural milk improves intestinal function and development of a stable and beneficial microbiota in the gastrointestinal tract(Reference Edwards and Parrett3) relative to milk formula. The anti-microbial and anti-inflammatory properties of milk are most pronounced for the first milk (colostrum), although most known milk bioactives can be found throughout lactation. Milk components with preliminary evidence of positive effects on the intestinal immune system and microbiota include osteopontin (OPN), gangliosides (Gang) and sialic acids (SL)(Reference Kelleher and Lönnerdal1, Reference Ashkar, Weber and Panoutsakopoulou4–Reference Wang and Brand-Miller10). Characterisation of the biological effect of such components is important for optimal production of infant milk formulas to be used when mother's milk is not available. Furthermore, these compounds could be particularly important for nutrition of sensitive newborns, such as preterm infants.
OPN is a phosphorylated acidic glycoprotein expressed by a number of different immune and non-immune cells and tissues, and it is also known as early T cell activation 1 protein(Reference Ashkar, Weber and Panoutsakopoulou4, Reference Denhardt, Noda and O'Regan11, Reference Sodek, Ganss and McKee12). Secreted OPN has cytokine-like properties. It enhances interferon-γ-producing T helper 1 cell responses by inducing production of IL-12 and suppressing production of IL-10(Reference Ashkar, Weber and Panoutsakopoulou4, Reference Sato, Nakai and Tamura13, Reference Sodek, Batista Da Silva and Zohar14). Hence, expression of OPN has been shown to be important in the efficient development of the T helper 1 immune response, and OPN is recognised as a key cytokine involved in T helper 1 cytokine expression at sites of inflammation. Many studies have reported increased production of OPN during various inflammatory processes(Reference Ashkar, Weber and Panoutsakopoulou4, Reference Sato, Nakai and Tamura13–Reference Scatena, Liaw and Giachelli16). Overall, OPN can be categorised as an important regulator of processes involved in inflammation and tissue repair; in particular, those responses characterised by the presence of macrophages and T cells. Besides lymphocyte activation and involvement in a number of other steps of cell-mediated immunity, OPN is also involved in cell survival and wound repair(Reference Denhardt, Noda and O'Regan11, Reference Sodek, Batista Da Silva and Zohar14). Due to the multifunctional immunoregulatory cytokine properties of OPN, and since it is present in such high amounts especially during early lactation, it is very likely that OPN plays a significant role in the early immunological development of infants.
Gang are complex glycosphingolipids comprising a hydrophobic ceramide tail attached to a hydrophilic oligosaccharide with at least one SL molecule. They are widely distributed in vertebrate tissues, attached to cell surfaces or secreted to plasma and other body fluids(Reference Bergelson17–Reference Takamizawa, Iwamori and Mutai20). Breast milk contains Gang, and milk from different species has specific Gang profiles with contents varying during lactation(Reference Bronnum, Seested and Hellgren18, Reference Rueda, Maldonado and Narbona19, Reference Martin-Sosa, Martin and Garcia-Pardo21–Reference Rueda23). Certain glycosylated milk Gang may serve as false receptor analogues for bacterial adhesins. Thus, they may be able to modify the intestinal microbiota in the neonate(Reference Martin, Martin-Sosa and Alonso7, Reference Rueda, Maldonado and Narbona19). In vitro, Gang exhibit immunosuppressive effects on a number of immunocompetent cells, and these effects are dependent on Gang structure, concentration and the type of immune cell affected(Reference Bergelson17, Reference Bronnum, Seested and Hellgren18, Reference Ladisch, Becker and Ulsh24).
SL comprise a diverse group of acidic sugars with a nine-carbon backbone. Typically, these are found on the terminal ends of glycan chains attached to lipids and proteins covering surfaces of all cells and a variety of secreted proteins in vertebrates. Here, they serve as components of binding sites for pathogens and toxins that recognise the SL unit in combination with different underlying carbohydrate chains of the cell surface glycans(Reference Wang and Brand-Miller10, Reference Varki25). SL moieties on, for example, Gang can therefore inhibit attachment of pathogenic bacteria species such as Vibrio cholerae and enterotoxic Escherichia coli by acting as decoy receptors(Reference Laegreid, Otnaess and Fuglesang26).
Dendritic cells (DC) reside in the Peyer's patches and lamina propria of the intestine and are important antigen-presenting cells of the gut-associated immune system. We have previously shown that murine bone marrow-derived DC respond strongly to bacterial stimuli(Reference Christensen, Frokiar and Pestka27), and this response can be modulated with various milk components(Reference Bronnum, Seested and Hellgren18, Reference Mikkelsen, Bakman and Sorensen28). Hence, the DC assay can be used as a screening tool to study the immunomodulatory effects of various milk bioactives.
In preterm pigs, maternal colostrum protects against necrotising enterocolitis (NEC) in the small intestine (SI)(Reference Bjornvad, Thymann and Deutz29–Reference Van Haver, Oste and Thymann31), while infant formula leads to digestive and immune dysfunction and NEC lesions. We hypothesised that these diet differences are explained partly by the lack of milk bioactives like OPN, Gang and SL in commercial milk formulas, relative to mother's milk, or a diet like bovine colostrum. We therefore tested the in vivo intestinal response to milk formulas enriched with OPN, Gang or SL in preterm pigs. Furthermore, the immunomodulatory activity of the enriched milk fractions was investigated in vitro using DC stimulated with gut-associated bacteria. Both the overall bacterial load and specific groups (such as Clostridia) are suspected to play a role in NEC aetiology(Reference De la Cochetiere, Piloquet and Des Robert32).
Materials and methods
Animals, surgery and nutrition protocols
Forty-seven piglets (Large White × Danish Landrace) from three litters were delivered by caesarean section at 105 d of gestation as previously described(Reference Sangild, Siggers and Schmidt30). While still anaesthetised from delivery, each piglet was fitted with an orogastric feeding tube (infant feeding tube 6F; Portex, Kent, UK) and a vascular catheter (4F; Portex) in the dorsal aorta via the transected umbilical cord(Reference Bjornvad, Thymann and Deutz29). Within 6 h of delivery, all the piglets were administered total parenteral nutrition (TPN), continuously infused through the arterial catheter for 48 h. Initially, the rate was 4 ml/kg body weight per h advancing to 6 ml/kg body weight per h after 12 h. The TPN solution was prepared aseptically as previously described and adjusted to meet the nutritional requirements of the preterm pig(Reference Bjornvad, Thymann and Deutz29, Reference Sangild, Bjornvad and Petersen33, Reference Siggers, Siggers and Boye34). All the pigs were immunised with three gradually increasing doses of porcine plasma obtained from their mother(Reference Siggers, Thymann and Jensen35).
Enteral milk formulas used as control diets were prepared from commercially available formula products (Pepdite, Maxipro, Liquigen; SHS International, Liverpool, UK) and adjusted to match the energy and protein concentrations in porcine milk during lactation. The three enriched milk formulas were prepared by the addition of pure bovine OPN or Gang- or SL-enriched bovine milk fractions (all from Arla Foods Ingredients, Viby J, Denmark) as described later. The protein, lipid and carbohydrate contents were adjusted according to the additional nutrients added to each formula to obtain similar compositions for all formulas within the same experiment. As the Gang- and SL-enriched milk products were not completely pure, like the OPN product, these were tested in a separate experiment where the control formula was adjusted to match the composition of the Gang and SL formulas. Hence, the formula control diets of the two experiments were slightly different. The final composition of the applied formulas is shown in Table 1. Bovine colostrum was collected and pooled from two to three Holstein cows from the first milking after calving and stored at − 20°C until use. The content of OPN, SL and Gang in bovine milk has been documented elsewhere(Reference Martin-Sosa, Martin and Garcia-Pardo21, Reference Pan and Izumi22, Reference Schack, Lange and Kelsen36).
Table 1 Composition of enriched (osteopontin (OPN), gangliosides (Gang) and sialic acids (SL)) and control formulas (per litre formula)
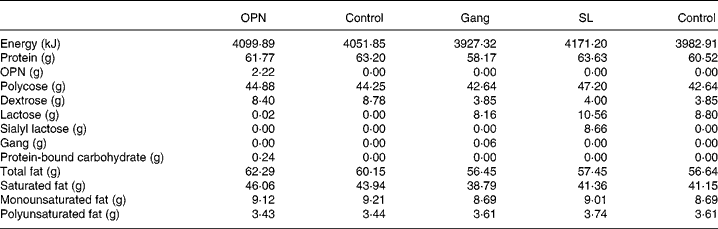
After the TPN period, the pigs were switched to enteral nutrition. Every 3 h, all the pigs were manually given oral boluses of 15 ml/kg of their respective diets, and the pigs were monitored every 3 h for potential clinical signs of NEC (feeding intolerance, abdominal distension, diarrhoea and respiratory stress). The pigs were euthanised for sample collection 1·5 d after initiation of enteral feeding unless serious clinical NEC symptoms developed beforehand. All animal protocols and procedures were approved by the Danish National Committee on Animal Experimentation.
Expt 1: osteopontin-enriched formula to preterm pigs
Twenty-six preterm pigs derived from three litters were randomly assigned to two treatment groups. During the 2 d PN period, one group was given minimal enteral doses of 5 ml/kg body weight per 3 h of pure OPN diluted in sterile deionised water to a concentration of 2 g/l (OPN, n 13), while the controls (n 13) were given the same volume of deionised water. After the PN period, both the groups were switched to total enteral nutrition and fed either the standard milk formula (controls) or the same formula enriched with 2·2 g/l of OPN (OPN group).
Expt 2: colostrum or gangliosides- or sialic acids-enriched formulas to preterm pigs
Twenty-one pigs were obtained from three separate litters and administered TPN as described earlier. Following TPN, the pigs were assigned to a bovine colostrum group (n 5) and three formula groups. The formula groups were given either standard milk formula (control, n 4), formula with an SL-enriched bovine milk fraction (60 g/l corresponding to 8·7 g/l of sialyl lactose in the final solution, SL, n 6) or formula with Gang-enriched bovine milk fraction (3 g/l, final concentration of Gang was 0·06 g/l formula, Gang, n 6). The number of animals allocated to the treatment groups in Expt 2 (n 4–6) was kept relatively small because the studies quickly documented high NEC severity scores in all three formula groups, with marked differences relative to bovine colostrum. The concentration of SL used in this experiment corresponded to the concentration in human colostrum(Reference Martin, Martin-Sosa and Alonso7, Reference Gill, Doull and Rutherfurd37), and immunomodulatory effects of milk proteins containing SL moieties have been documented in vitro and in vivo (Reference Varki9, Reference Varki25, Reference Mikkelsen, Bakman and Sorensen28, Reference Gill, Doull and Rutherfurd37, Reference Varki and Varki38).
In vivo carbohydrate absorption
The test was performed twice by the end of the PN period and 1 d after initiation of enteral nutrition. Boluses of 15 ml/kg body weight of 2 % mannitol and 5 % galactose were administered orally, and arterial blood samples were collected at intervals (0, 20, 40 and 60 min) after the two boluses. Concentrations of mannitol and galactose in plasma were analysed spectrophotometrically as previously described(Reference Kurz, Wallenfels and Bergmeyer39, Reference Thymann, Burrin and Tappenden40).
Necrotising enterocolitis evaluation and tissue collection
When clinical symptoms of NEC were observed in several pigs in a litter or no later than 1·5 d after initiation of enteral nutrition, the pigs were anaesthetised and subsequently killed with an intra-cardiac injection of sodium pentobarbitone (60 mg/kg). The entire gastrointestinal tract was removed, and the SI, colon and stomach were weighed without contents. SI length was measured in a relaxed state, and the intestine was divided into three equal segments designated proximal, middle and distal SI. The wet mass of lungs, liver, spleen, heart and kidneys was recorded, and the lung volume was determined by immersion in water. Samples from the three SI regions were snap frozen in liquid N2 and stored at − 80°C before analysis of enzymatic activity. Another set of samples was fixed in 4 % neutral-buffered paraformaldehyde for villus morphology as described previously(Reference Bjornvad, Thymann and Deutz29, Reference Sangild, Bjornvad and Petersen33).
Each SI segment, stomach and colon were photographed and given a macroscopic pathologic lesion score on a scale from 1 to 6. Here, 1 indicates the absence of any NEC-associated symptoms; 2 indicates local hyperaemia, inflammation and oedema; 3 indicates hyperaemia, extensive oedema and local haemorrhage; 4 indicates extensive haemorrhage; 5 indicates local necrosis and pneumatosis intestinalis; and 6 indicates extensive necrosis and pneumatosis intestinalis. A pig having a score of 3 or more in any region was considered a case of NEC. An average NEC severity score across all five regions was calculated for each pig, and an average score for each treatment group was calculated from these average scores. The proportion of dry weight of mucosa was determined as previously described(Reference Sangild, Siggers and Schmidt30).
Intestinal mucosal morphology and enzyme activities
Fixed SI samples were embedded in paraffin, sectioned (3 μm), and mounted on glass slides and stained with haematoxylin and eosin. Villus heights of ten intact villi from one representative cross-section from each pig were measured on scanned images of the tissue sections using the software SoftWoRx Explorer version 1.1 (Applied Precision, Issaquah, Washington, DC, USA).
Homogenates of frozen samples from the three SI segments from each pig were assayed spectrophotometrically for activities of lactase, sucrase, maltase, dipeptidyl-peptidase IV, aminopeptidase N and aminopeptidase A. Lactose, sucrose, maltose, glycyl-l-prolin-4-nitroanilide, l-alanine-4-nitroanilide and α-l-glutamic acid 4-nitroanilide were used, respectively, as substrates, in accordance with a previously established protocol(Reference Sangild, Sjostrom and Noren41). The hydrolytic release of 1 μmol substrate per min at 37°C was considered to represent one unit (U) of enzyme activity, which was expressed per gram of wet intestine.
Expt 3: in vitro immunomodulatory activity of milk bioactives
Preparation of bacterial strains
Clostridium perfringens NEC A20 (type A isolate from a premature piglet with NEC) was grown anaerobically overnight at 37°C in tryptone soya broth (Oxoid A/S, Greve, Denmark). E. coli Nissle 1917 (O6:K5:H1, isolate from human faeces) was cultured aerobically in de Man, Rogosa and Sharpe broth (Merck, Darmstadt, Germany) overnight at 37°C. Bacterial cultures were harvested by centrifugation at 2700 g for 15 min, washed twice in sterile PBS (pH 7·2) and resuspended in PBS. Bacterial cells were killed by 1 h exposure to UV light and then stored at − 80°C. Bacterial concentrations were determined from dry mass of freeze-dried samples and corrected for buffer salt concentration.
Preparation of dendritic cells and co-culturing with milk bioactives and bacteria
Bone marrow cells were flushed with PBS from the dissected femur and tibia from C57BL/6 mice. The cells were washed twice in PBS and resuspended in Roswell Park Memorial Institute-1640 supplemented with 2 mm-l-glutamine, 10 % (v/v) heat-inactivated foetal calf serum, 100 U/ml penicillin, 100 μg/ml streptomycin, 50 μm-2-mercaptoethanol (culture medium, all components from Lonza, Basel, Switzerland) and 15 ng/ml murine granulocyte-macrophage colony-stimulating factor (supernatant from a granulocyte-macrophage colony-stimulating factor transfected Ag8·653 myeloma cell line). The cells were incubated for 8 d in petri dishes at 37°C and 5 % CO2. On days 3 and 6 of incubation, the cells were supplied with fresh culture medium containing 15 ng/ml granulocyte-macrophage colony-stimulating factor. On day 8, non-adherent cells were harvested and resuspended in culture medium with 10 ng/ml granulocyte-macrophage colony-stimulating factor. A total of 2 × 106 cells/ml were seeded in forty-eight-well tissue culture plates, and 10 μg/ml bacteria were added together with different concentrations of OPN, SL, Gang, bovine colostrum or colostrum whey diluted appropriately in culture medium. Colostrum whey was produced by coagulation with 3 μl/ml bovine chymosin (Chr. Hansen, Horsholm, Denmark) for 30 min at 37°C and subsequent centrifugation for 10 min at 280 g. The final volume in each well was 600 μl. The cells were stimulated for 18–20 h at 37°C and 5 % CO2 before collection of cell culture supernatants, which were stored at − 20°C until cytokine analysis. The concentration of cytokines IL-12p70, IL-10, IL-6 and TNF-α was determined using commercially available ELISA kits (R&D Systems, Minneapolis, MN, USA) according to the manufacturer's instructions.
Statistical analysis
ELISA data from the DC experiments were evaluated by one-way ANOVA with Dunnett's multiple comparison post hoc test using Graph Pad Prism (version 5.01; Graph Pad Software Inc., La Jolla, CA, USA). Villi heights were compared with one-way ANOVA and Tukey's post hoc test (Expt 2) or Student's t test (Expt 1) using Graph Pad Prism. Enzyme activity and NEC severity score data were analysed by two-way ANOVA for the two in vivo experiments separately using the mixed procedure of Statistical Analysis Systems (SAS/STAT version 9.1; SAS Institute, Cary, NC, USA). Treatment and intestinal region were fixed effects, while pig and litter were regarded as random variables. Sugar absorption data were analysed with repeated-measures analysis using Statistical Analysis Systems. NEC incidence was analysed using Fisher's exact procedure test with subsequent pairwise comparisons of treatment groups. Data are presented as means with their standard errors or means and standard deviations in all statistical analyses; P values < 0·05 were considered significant.
Results
Expt 1 and 2: in vivo studies on enriched bovine milk fractions and colostrum
In vivo carbohydrate absorption
The in vivo response to oral boluses of galactose and mannitol by the end of the TPN period and 1 d after the initiation of enteral nutrition showed no significant difference between OPN and controls (repeated-measures analyses of graphs in Fig. 1). However, 60 min after the mannitol bolus, 2-d-old piglets supplemented with OPN during the TPN period had significantly elevated plasma mannitol levels, compared with controls (P < 0·05, Fig. 1(B)). Both the groups had much lower plasma increments of galactose and mannitol during enteral feeding than during the TPN period.
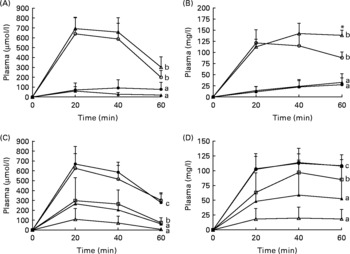
Fig. 1 Absorption of galactose ((A) and (C)) and mannitol ((B) and (D)) by the end of the 2 d total parenteral nutrition (TPN) period and 1 d after start of enteral feeding. Values represent increments in plasma galactose and mannitol at 20, 40 and 60 min (means with their standard errors). Enriched formulas are compared with colostrum and control formulas, respectively, and the entire response was analysed by repeated measures. a,b,c Mean values with unlike superscript letters assigned to the curves indicate galactose and mannitol responses that differ significantly (P < 0·05). * Mean values for the TPN–osteopontin (OPN) and OPN groups differ significantly specifically at 60 min in (B) (P < 0·05, by t test). ((A) and (C)) –△–, TPN–OPN; –○–, TPN control; –▲–, OPN; –●–, control. ((B) and (D)) –○–, TPN all pigs; –▲–, sialic acids; –△–, gangliosides; –●–, colostrums; –□–, control.
In Expt 2 after initiation of enteral nutrition, Gang and SL lowered the galactose and mannitol absorption relative to controls, while the absorption capacity was higher in colostrum pigs compared with all formula-fed pigs (Fig. 1, P < 0·01).
Necrotising enterocolitis incidence and severity
OPN supplementation (Expt 1) did not reduce the incidence of NEC (54 and 77 % in the OPN and control groups, respectively, Table 2). However, the mean NEC severity score was significantly reduced in the OPN group (2·5 (sem 0·3) v. 3·7 (sem 0·3), P < 0·01, Table 2). In Expt 2, all the three formula groups showed high NEC incidence (83–100 %), while only 40 % (2/5) of the pigs in the colostrum group developed NEC (Table 2). Only mild signs of NEC (grades 2 and 3) were observed in the colon and distal SI of two pigs fed colostrum. Hence, the NEC severity score of the colostrum group was significantly lower (P < 0·0001) than all three formula-fed groups, which did not differ. In both the experiments, there were no significant differences in organ weights, intestinal dimensions and mucosal proportions among treated groups and their respective control groups (data not shown).
Table 2 Necrotising enterocolitis (NEC) incidence and severity and small intestine structural and functional indices
(Mean values with their standard errors)
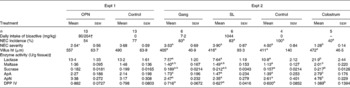
OPN, osteopontin; Gang, gangliosides; SL, sialic acids; ApA, aminopeptidase A; ApN, aminopeptidase N; DPP IV, dipeptidyl-peptidase IV.
a,b Means values within the same row with unlike superscripts letters were significantly different (P < 0·05) for Expt 2.
* Mean values were significantly different for OPN compared to controls in Expt 1 (P < 0·05).
† OPN intake during total parenteral nutrition–minimum enteral nutrition and enteral feeding period, respectively.
‡ The hydrolytic release of 1 μmol substrate per min at 37°C represents one unit of enzyme activity, expressed per g wet intestine.
Intestinal mucosal morphology and enzyme activities
Microscopic examination of haematoxylin and eosin-stained sections of the distal SI revealed obvious pathological differences between pigs macroscopically diagnosed with NEC compared to those pigs diagnosed as healthy. The NEC pigs had extensive bleeding and mucosal atrophy, and in very severe cases (NEC severity score of 5–6), complete loss and disintegration of the mucosa, submucosa and muscularis layers were observed (Fig. 2). Measurement of villus height showed no significant differences between the OPN and control groups in Expt 1 or among the four groups in Expt 2 (Table 2).
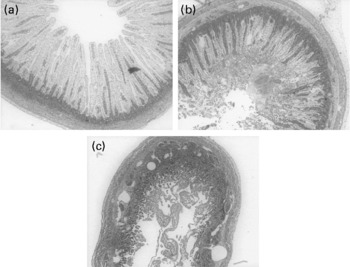
Fig. 2 Cross-sections of distal intestinal samples from sick and healthy pigs representing different degrees of necrotising enterocolitis (NEC) evaluated macroscopically at tissue collection and given a macroscopic pathologic lesion score on a scale from 1 to 6: 1 indicates the absence of any NEC-associated symptoms; 2 indicates local hyperaemia, inflammation and oedema; 3 indicates hyperaemia, extensive oedema and local haemorrhage; 4 indicates extensive haemorrhage; 5 indicates local necrosis and pneumatosis intestinalis; and 6 indicates extensive necrosis and pneumatosis intestinalis. (a) Control formula-fed pig, NEC score 1; (b) pig fed gangliosides-enriched formula, NEC score 4; (c) control formula-fed pig, NEC score 6.
There was no statistical difference in the activity of any of the measured enzymes between OPN and control pigs (Expt 1) and among the Gang, SL and control formula groups in Expt 2 (Table 2). Pigs fed bovine colostrum had significantly higher activities of all measured enzymes relative to the control formula group. Compared with the Gang and SL groups, all the enzyme activities except for sucrase and maltase were also significantly improved with bovine colostrum, most pronounced for lactase (approximately a twofold activity increase, Expt 2, Table 2).
Expt 3: in vitro immunomodulatory activity of milk fractions
For all four measured cytokines (IL-10, IL-12, IL-6 and TNF-α), there were no significant differences between the bacteria-stimulated DC and the DC co-stimulated with bacteria and 0·1, 0·5, 1 and 5 μg/ml of OPN (data not shown). The Gang-enriched milk product added to DC alone did not induce a cytokine response from the cells (Fig. 3(a) and (b)). High doses of the Gang-enriched milk product co-incubated with DC and bacteria resulted in reduced production of IL-10 and IL-12, with strongest inhibition of E. coli Nissle-induced cytokine production (Fig. 3(a) and (b)). There were no effects of Gang on the TNF-α and IL-6 responses (data not shown). When the SL-enriched milk product was added to the DC alone, it induced production of all four cytokines (Fig. 3(c) and (d)). Upon co-stimulation with bacteria, no effect on cytokine production of the SL-enriched milk product was observed for doses lower than 250 μg/ml. Conversely, higher concentrations resulted in a general increase in cytokine production independently of bacterial stimulation (Fig. 3(c) and (d), IL-6 and TNF-α data are not shown).
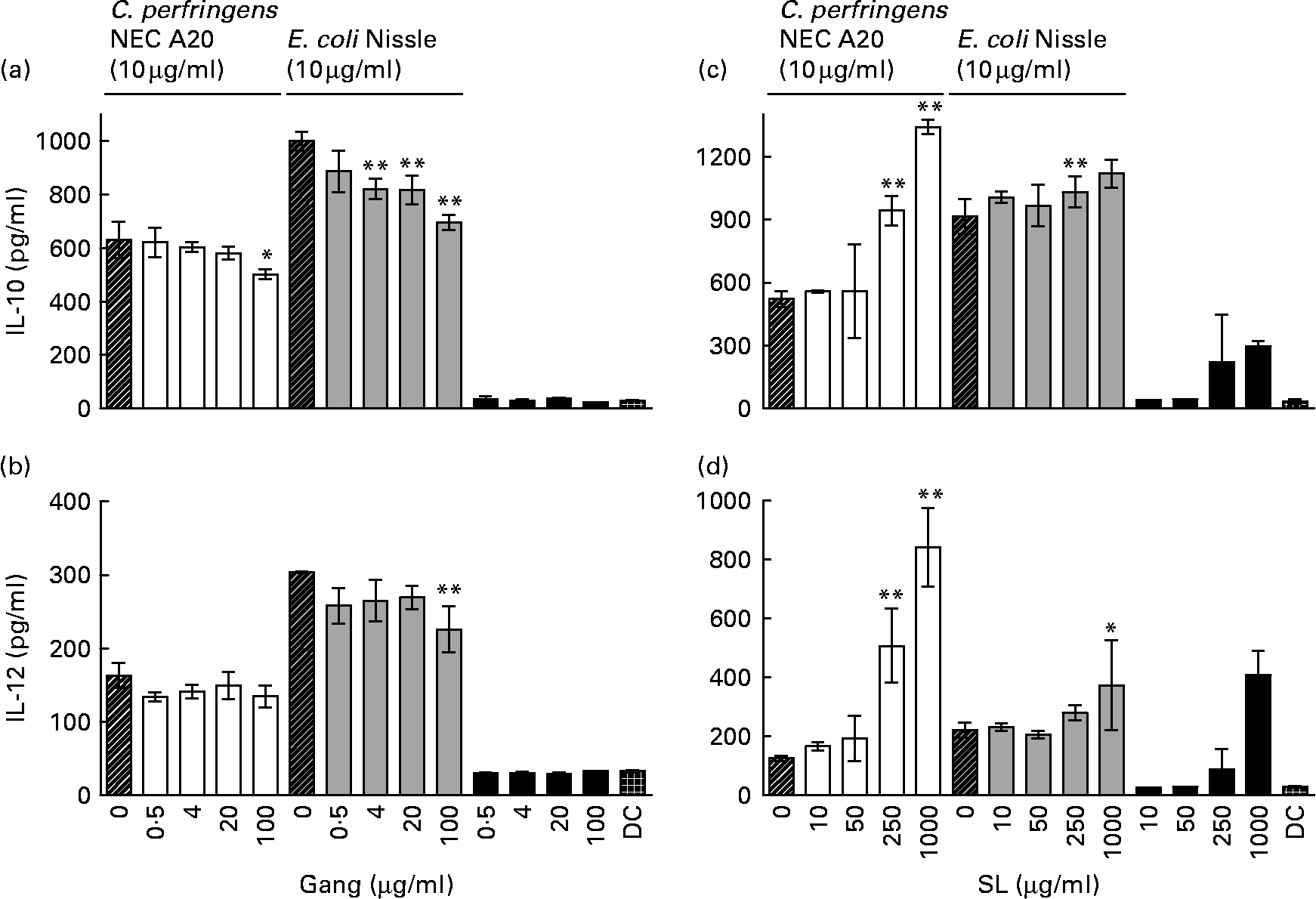
Fig. 3 IL-10 ((a) and (c)) and IL-12 ((b) and (d)) expression in dendritic cells (DC) co-incubated with 10 μg/ml Clostridium perfringens NEC A20 or Escherichia coli Nissle 1917 and different concentrations of sialic acids (SL) and gangliosides (Gang). The results are means and standard deviations of three replicates from one experiment, representative of two separate experiments. Values are compared to their respective controls without SL and Gang (striped bars). Mean values were significantly different: *P < 0·05, ** P < 0·01.
Defatted colostrum was associated with high mortality of DC, and only 0·9 % colostrum was tolerated by the cells resulting in similar effects as for low concentrations of whey (data are not shown). Therefore, cytokine production was measured after co-incubation with 10 μg/ml C. perfringens NEC A20 or E. coli Nissle 1917 and 8·3, 2·8 and 0·9 % (v/v) of bovine colostrum whey, which was not lethal to the DC. Colostrum whey alone induced some production of all four cytokines except IL-12 (Fig. 4). The whey fraction caused a bacteria-dependent modulation of the cytokine response from the stimulated DC. For E. coli-stimulated cells, whey caused a dose-dependent decrease in production of all cytokines (Fig. 4(a)–(d), P < 0·01 and 0·001), while inhibiting effects on cytokine production were modest after C. perfringens stimulation. Production of IL-10 was induced with whey only after C. perfringens stimulation (Fig. 4(a), P < 0·05 and 0·01).
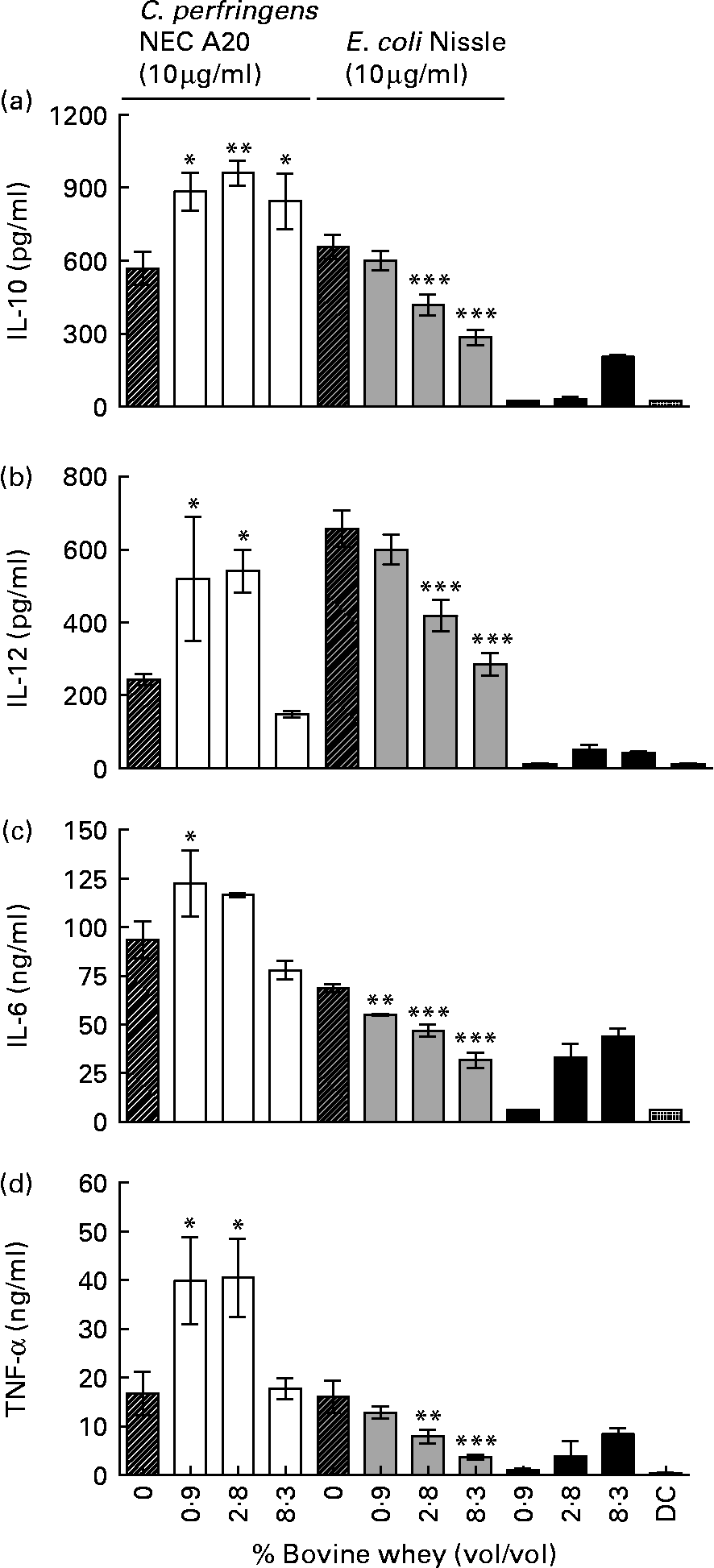
Fig. 4 IL-10 (a), IL-12 (b), IL-6 (c) and TNF-α (d) expression in dendritic cells (DC) co-incubated with 10 μg/ml Clostridium perfringens NEC A20 or Escherichia coli Nissle 1917 and different concentrations of a whey fraction from bovine colostrum. The results are means and standard deviations of three replicates from one experiment, representative of two separate experiments. Values are compared to their respective controls without whey (striped bars). Mean values were significantly different: *P < 0·05, **P < 0·01, ***P < 0·001.
Discussion
The results show that enteral feeding with bovine colostrum reduces NEC lesions in preterm pigs, relative to formula, as shown previously for porcine colostrum(Reference Bjornvad, Thymann and Deutz29, Reference Sangild, Bjornvad and Petersen33–Reference Siggers, Thymann and Jensen35, Reference Thymann, Burrin and Tappenden40, Reference Oste, Van Ginneken and Van Haver42, Reference Sangild, Petersen and Schmidt43). It now remains to be investigated whether bovine colostrum, or fractions thereof, might also prevent gut inflammation and NEC in preterm infants lacking access to mother's milk. Formulas enriched with Gang or SL failed to improve resistance in pigs, while OPN supplementation was associated with a modest decrease in NEC without any consistent effects on gut structural and functional indices. The potential immune-modulating activity of bovine colostrum was supported by the effects of colostrum whey on DC stimulated in vitro with either E. coli Nissle 1917 (a commensal probiotic bacteria) or C. perfringens NEC A20 (an opportunistic NEC pathogen). In contrast, the enriched milk fractions showed no consistent effects, except that high concentration of Gang reduced the IL-10 and IL-12 production in bacteria-stimulated DC.
In vivo, bovine colostrum improved several gut functional indices (galactose and mannitol absorption, dipeptidyl-peptidase IV, aminopeptidase A, aminopeptidase N and lactase activities), while the effects of enriched formulas were limited or absent, at least for the doses chosen in the present study. The activities of six intestinal brush-border enzymes were elevated in pigs fed bovine colostrum, relative to formula, confirming previous findings with porcine colostrum(Reference Bjornvad, Thymann and Deutz29, Reference Sangild, Bjornvad and Petersen33, Reference Siggers, Siggers and Boye34, Reference Thymann, Burrin and Tappenden40). Digestive enzyme activities and nutrient absorptive capacity are sensitive parameters to detect digestive dysfunction, and they often correlate well with gut integrity and degree of inflammation(Reference Buddington, Bering and Thymann44). In the present study, both the galactose and mannitol absorptions were reduced 1 d after initiation of enteral formula feeding. Other recent studies have indicated that this formula-induced decrease in nutrient absorptive capacity may take place a few hours after the start of feeding(Reference Thymann, Moller and Stoll45), coinciding with a very rapid formula-induced increase in tissue inflammatory markers such as IL-1β(Reference Van Haver, Oste and Thymann31), but before structural changes. In the present study, colostrum did not improve mucosal function by stimulating structural integrity, since we found no significant treatment differences for villus height, mucosa proportion or intestinal weight in the two experiments. Mean villus height in the bovine colostrum group was relatively low (472 μm) compared with preterm pigs fed porcine or bovine colostrum starting from the time of birth, without a TPN period (approximately 600 μm)(Reference Sangild, Siggers and Schmidt30, Reference Siggers, Siggers and Boye34). Colostrum was not fully protective, as two of the five colostrum pigs showed mild NEC lesions in the distal intestine and colon. In agreement with our earlier observations(Reference Bjornvad, Thymann and Deutz29), a few days of TPN after birth may predispose preterm pigs to moderate inflammatory lesions, even when a near-optimal diet (porcine or bovine colostrum and OPN-enriched formula) is used as an enteral diet after TPN. Furthermore, we have also previously observed that minimal enteral doses of bovine colostrum during TPN followed by full enteral nutrition with bovine colostrum fully protect against NEC(Reference Cilieborg, Boye and Thymann46). These previous findings with bovine colostrum indicate that it is only fully protective against NEC in the preterm pig model if given immediately after birth as it seems to counteract the detrimental effects of initial TPN.
In vitro, bovine colostrum whey caused a bacteria-dependent modulation of cytokine responses from stimulated DC. In recent studies with the DC model, we have found similar modulatory effects of porcine and human whey, indicating conserved immunomodulatory effects between different species (HK Møller, unpublished results). In contrast, the enriched milk fractions showed no consistent effects, except high concentration of Gang, which reduced the IL-10 and IL-12 production in bacteria-stimulated DC.
In vitro, it has recently been shown that a bovine colostrum protein concentrate is able to modulate cytokine production from lipopolysaccharide-stimulated human peripheral blood mononuclear cells by increasing release of IL-2 while suppressing early production of TNF, IL-6 and IL-4(Reference Shing, Peake and Suzuki47). The same study found an increase in IL-10 and IL-2 production and inhibition of interferon-γ and TNF, when cells were stimulated with phytohaemagglutinin in the presence of colostrum(Reference Shing, Peake and Suzuki47). Another study found that bovine colostrum was able to modulate interferon-γ production in stimulated human peripheral blood mononuclear cells depending on the strength and type of antigenic stimulation(Reference Biswas, Vecchi and Mantegani48). Our findings using murine DC extend these properties to cell types other than peripheral blood mononuclear cells. As the present results were obtained using the whey fraction of colostrum, they, together with the Shing et al. study(Reference Shing, Peake and Suzuki47), indicate that the immunomodulatory properties may be due to bioactive whey proteins. Previously, we have found immunosuppressive activity of sialylated milk proteins on the DC cytokine response to lipopolysaccharide, which again supports the notion that the proteins of colostrum play an important role in preventing inflammation(Reference Mikkelsen, Bakman and Sorensen28). It must also be noted that the in vitro data indicate a stimulus dependency of the effects of colostrum, which may be an important regulatory mechanism behind the protective effects of colostrum against inflammation in vivo.
The present study is the first to test luminal effects of OPN in a porcine model of gut immaturity and inflammation. Based on the immune-modulating effects of OPN documented by others under other conditions(Reference Ashkar, Weber and Panoutsakopoulou4, Reference Begum, Umemura and Kon5, Reference Renkl, Wussler and Ahrens8, Reference Denhardt, Noda and O'Regan11, Reference Sato, Nakai and Tamura13, Reference Sodek, Batista Da Silva and Zohar14, Reference Giachelli, Lombardi and Johnson49–Reference Xanthou, Alissafi and Semitekolou51), we hypothesised that milk-derived OPN played a role in the control of inflammatory responses in neonates. OPN exhibits pro-survival and anti-apoptotic properties, and thus aids cell survival(Reference Khan, Lopez-Chua and Zhang50), wound healing and matrix formation(Reference Denhardt, Noda and O'Regan11, Reference Sodek, Ganss and McKee12, Reference Da Silva, Pollett and Rittling52), supporting the observed tendency to positive clinical effects in the present study, where OPN may positively affect rebuilding of necrotic mucosa and tissue or through anti-apoptotic signals promoting epithelial cell survival. Further studies on OPN concentrations and mode of administration (enteral v. parenteral) are warranted. Although OPN significantly reduced NEC severity, the clinical effects of OPN on gut function parameters using the present treatment regimen were minimal. It is possible that OPN effects are developmentally dependent and that the gut-associated lymphoid tissue is too immature in preterm pigs to be able to fully respond to OPN.
Developmental immaturity may also contribute to the lacking effects of SL- and Gang-enriched formulas. Furthermore, the concentrations of these bioactives in the formulas may have been too low to provide beneficial effects in vivo. The finding that SL only affected DC function at very high concentrations could indicate that a DC-stimulating contaminant, such as lipopolysaccharide, may have been present in the enriched formula. Different structures of Gang have been shown to exhibit immunosuppressive effects on various immunocompetent cell types in vitro, and these effects seem to be dependent on the Gang structure and type of immune cell affected(Reference Bergelson17, Reference Bronnum, Seested and Hellgren18, Reference Ladisch, Becker and Ulsh24). Clinically, a Gang-enriched formula has been found to stimulate the development of a beneficial gut microbiota in preterm infants, relative to control formula(Reference Rueda, Maldonado and Narbona19). Milk Gang and conjugated sialylated carbohydrates (glycoproteins and glycolipids) may also serve as false receptor analogues for bacterial adhesins, and thus be able to modify the intestinal microbiota in the neonate serving as a beneficial prebiotic.
As in human milk, the predominant Gang of bovine milk are disialganglioside 3 (GD3) and monosialganglioside 3 (GM3), but in general, human milk contains higher amounts of Gang than bovine milk(Reference Martin, Martin-Sosa and Alonso7, Reference Rueda, Maldonado and Narbona19). Hence, the Gang-rich milk product employed in the present study most likely contains GD3 and GM3, and concentrations approached those in human milk. We observed a dose-dependent suppression of E. coli Nissle 1917-induced IL-10 and IL-12 cytokine production, probably due to the effects of GD3 and GM3. This slight immunosuppressive effect of the Gang milk product on the DC cytokine response confirms our previous findings with pure Gang(Reference Bronnum, Seested and Hellgren18). In conclusion, bovine colostrum was superior to milk formulas enriched with milk OPN, Gang or SL in protecting against NEC in preterm piglets. OPN tended to reduce NEC as indicated by a clearly reduced severity score but without any consistent improvement of structural and functional indices. The modest effect of milk bioactives may be explained by the immature state of the gut immune system in preterm pigs. Equally well, the effects of the enriched formulas may just be minimal compared with that of the synergistic actions of many bioactives present in intact bovine colostrum. These synergistic actions of colostral bioactives appear to include inhibitory effects on bacteria-stimulated DC cytokine release.
Acknowledgements
We gratefully acknowledge the technical assistance from Elin Skytte, Bente Synnestvedt and Anni Mehlsen. The work was supported by the FOSU-DSF programme by the Danish Research Councils, the Technical University of Denmark and Arla Foods Ingredients. One of the co-authors, A. S. K., is employed by Arla. Although she took part in the design of the studies, and initial interpretation of the results, final responsibility for the manuscript is with the remaining authors who had no potential conflicts of interest. P. T. S., T. T., L. N. F., H. F. and A. S. K. designed the research; H. K. M., P. T. S. and T. T. conducted the research; A. S. K. provided essential reagents; H. K. M. and T. T. analysed data; H. K. M., T. T., L. N. F., H. F. and P. T. S. wrote the paper. H. K. M. had primary responsibility for the final content. All authors read and approved the final manuscript.