Atherosclerotic CVD is the main cause of death worldwide( Reference Caleyachetty, Echouffo-Tcheugui and Tait 1 ). Over the last few decades, an increase in CVD risk factors such as excess body weight, insulin resistance or glucose intolerance among others has been observed in both children and adolescents( Reference Brambilla, Lissau and Flodmark 2 ). Such evidences support claims to independently assess the effect of different CVD risk factors in these age groups and promote strategies to prevent future events of atherosclerosis( Reference Garaiova, Muchova and Nagyova 3 ).
Cardiovascular risk factors including high serum concentrations of total cholesterol, TAG or LDL-cholesterol or low levels of HDL-cholesterol are associated with the development of arterial fatty streaks( Reference Berenson, Srinivasan and Bao 4 ) and increased total homocysteine (tHcy) concentrations. The latter is considered an independent predictor of cardiovascular mortality or of all-cause mortality( Reference Peng, Man and Xu 5 ) because of its role in oxidative stress, endothelium damage and thrombogenicity enhancement( Reference Lonn, Yusuf and Arnold 6 ). However, a debate exists about whether tHcy should be interpreted as a risk factor or a biomarker of cardiovascular events.
Results from large prospective studies suggest that elevated circulating concentrations of tHcy are associated with increased risk of CVD, regardless of other well-known risk factors such as smoking, high blood pressure, high serum lipids and obesity( Reference Wald, Law and Morris 7 ). At the same time, results of a recent meta-analysis showed that tHcy-lowering interventions did not reduce the occurrence of cardiovascular events( Reference Veeranna, Zalawadiya and Niraj 8 ). It could be explained by the fact that homozygous carriers of the 677C-T polymorphism on the methylenetetrahydrofolate reductase gene have higher CVD risk, which implies higher tHcy circulating levels( Reference Wald, Law and Morris 7 , Reference Lewis, Ebrahim and Davey Smith 9 ).
A recent systematic review examining the impact of homocysteine-lowering interventions via increased vitamins B6, folate or B12 supplement uptake on cardiovascular events failed to identify any relationship( Reference Marti-Carvajal, Sola and Lathyris 10 ). This fact set out the possibility that the positive associations between serum homocysteine and CVD risk may be confounded by other cardiovascular risk factors( Reference Rodionov and Lentz 11 ) such as low plasma (n-3) long-chain PUFA levels. The main (n-3) long-chain PUFA such as EPA and DHA are suggested to act as cardioprotective elements by lowering blood pressure and heart rate, reducing serum TAG, thrombotic tendency, inflammation and arrhythmias and by improving endothelial function, insulin sensitivity, paraoxonase concentrations and plaque stability( Reference Hooper, Thompson and Harrison 12 ). Previous animal( Reference Durand, Prost and Blache 13 – Reference van Wijk, Watkins and Hageman 15 ) and human( Reference Crowe, Skeaff and McMahon 16 – Reference Zhao, Lamers and Ralat 18 ) studies have reported that some changes in plasma fatty acid (FA) concentrations might be explained by B-vitamin biomarkers because of their role as cofactors in the methylation of phosphatidylethanolamine (PE) to phosphatidylcholine (PC), crucial in the transport of PUFA in the blood. The latter could explain the controversial results observed regarding the association between tHcy and CVD risk. However, results obtained in such studies were also inconsistent among them( Reference Durand, Prost and Blache 13 – Reference Zhao, Lamers and Ralat 18 ).
Assumptions that B-vitamin biomarkers involved in tHcy regulation are linked to serum phospholipid (PL) FA profiles have not been studied in young populations yet. The aim of this study was to examine the association between vitamin B6, folate and vitamin B12 biomarkers (including tHcy) and fasting lipid profile and serum PL FA profile (focusing on n-3 FA) in healthy European adolescents.
Methods
Study design
The Healthy Lifestyle in Europe by Nutrition in Adolescence Cross-Sectional Study (HELENA-CSS) obtained standardised, reliable and comparable data on relevant nutrition and health-related parameters from a large sample of European adolescents( Reference Moreno, De Henauw and Gonzalez-Gross 19 ). Fieldwork took place from November 2006 to December 2007 in ten cities (Vienna, Ghent, Lille, Dortmund, Athens, Heraklion, Pecs, Rome, Zaragoza and Stockholm) of nine European countries (two in Greece).
Subjects
Adolescents aged 12·5–17·5 years were randomly recruited from selected schools. The HELENA-CSS study population consisted of 3528 adolescents (response rate 61·3 %)( Reference Beghin, Huybrechts and Vicente-Rodriguez 20 ). Of those, a subsample of 1089 adolescents was randomly selected for blood sampling. For the purposes of this study, only adolescents with available information on B-vitamin biomarkers, lipid profile, serum PL FA, BMI and Diet Quality Index (DQI) were included, resulting in 674 cases (43 % males). Sampling selection procedures can be observed in Fig. 1.
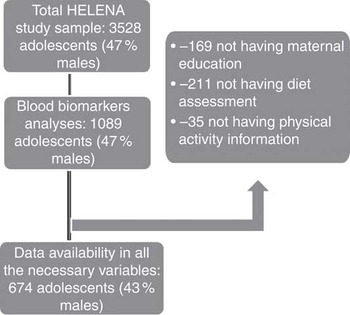
Fig. 1 Flow chart of the selection process from the Healthy Lifestyle in Europe by Nutrition in Adolescence (HELENA) study initial sample.
The present study was performed following the ethical guidelines of the Declaration of Helsinki, the Good Clinical Practice rules and the legislation about clinical research in human subjects of the participating countries. The Ethics Committees of the involved centres approved the study protocol and procedures, and all subjects and their parents or legal guardians signed an informed written consent form( Reference Beghin, Castera and Manios 21 ).
Assessment of vitamin B6, folate and vitamin B12 biomarker concentrations as well as serum phospholipid fatty acids
Assessment and analysis of biological samples in the study have already been described elsewhere( Reference Gonzalez-Gross, Benser and Breidenassel 22 , Reference Gonzalez-Gross, Breidenassel and Gomez-Martinez 23 ). In brief, fasting blood samples were collected by venepuncture at school. Plasma folate (PF), serum vitamin B12, tHcy and red blood cell folate (RBC-folate) were measured by competitive immunoassay (Immulite 2000; DPC Biermann GmbH) (CV for PF=5·4 %, RBC-folate=10·7 %, serum vitamin B12=5·0 %). Pyridoxal 5'-phosphate (PLP) was measured by HPLC (Varian Deutschland GmbH; CV=1 %). Serum holotranscobalamin (HoloTC) was assayed by microparticle enzyme immunoassay (Active B12; Axis-Shield Ltd, CV=5·1 %) using AxSYM (Abbott Diagnostics)( Reference Gonzalez-Gross, Benser and Breidenassel 22 ).
TAG as well as HDL- and LDL-cholesterol concentrations were enzymatically assayed by the Dimension RxL clinical chemistry system (Dade Behring).
Serum FA composition from PL was determined by capillary GC (model 3900; Varian GmbH) after extraction performed by TLC using the standard 1,2 Dipentadecanoyl-sn-glycero-3-phosphocholine. The total amount of FA was obtained as a result of the percentage area by integrating the area under the peak and dividing it by the total area for all FA. The CV was 4·4 % for all serum PL FA analyses( Reference Dumont, Huybrechts and Spinneker 24 ). The headings ‘saturated fatty acids’, ‘MUFA’, PUFA’, ‘trans’, ‘ω3’ and ‘ω6’ include all types of these FA identified during blood analyses.
Diet Quality Index for adolescents
DQI was computed from data obtained from two non-consecutive, 24-h recalls performed via a computer-based, self-reported tool called the HELENA Dietary Assessment Tool( Reference Vereecken, Covents and Sichert-Hellert 25 ). This tool was based on a previous version developed for Flemish adolescents, the Young Adolescents’ Nutrition Assessment on Computer valid for twenty-nine food groups aggregated according to the European Food Groups classification system( Reference Ireland, van Erp-Baart and Charrondiere 26 ) and for several nutrients apart from energy, including macronutrients, fibre, Ca, Fe and vitamin C( Reference Vereecken, Covents and Sichert-Hellert 25 ). Total daily food intake was distributed into six meal occasions. For each occasion, the user was invited to select all the consumed food items and beverages from a standardised food list. If any food or beverage was not included in the list, they could be added manually at any time. Household measurements or pictures of portion sizes were used to obtain information on quantities. The DQI used in this study was originally developed for preschool-aged children( Reference Huybrechts, Vereecken and De Bacquer 27 ), and was later adapted and validated for use in adolescents( Reference Vyncke, Cruz Fernandez and Fajo-Pascual 28 ) and consists of three components: dietary quality, dietary diversity and dietary equilibrium. This index is used as an indicator of compliance with the Flemish Food Based Dietary Guidelines, which are very similar to dietary guidelines in other countries including the WHO CINDI (Countrywide Integrated Non-communicable Disease Intervention programme) pyramid, making it applicable for a European population( Reference Vyncke, Cruz Fernandez and Fajo-Pascual 28 ).
Physical examination
Weight and height were measured with an electronic scale (SECA 861; Seca Ltd) and with a telescopic stadiometer (SECA 225; Seca Ltd) to the nearest 0·1 kg and to the nearest 0·1 cm, respectively( Reference Nagy, Vicente-Rodriguez and Manios 29 ). BMI was calculated as body weight( Reference Neveus, Eggert and Evans 30 ) divided by the square of height (m). In addition, BMI was adjusted for age and sex providing a BMI standard deviation score using the British 1990 growth reference data from the Child Growth Foundation( Reference Cole, Freeman and Preece 31 ).
Socio-economic status and self-reported physical activity
Maternal education was used as proxy for socio-economic status obtained via a self-administered questionnaire. This variable was assessed using four categories (elementary, lower secondary, higher secondary or tertiary education)( Reference Iliescu, Beghin and Maes 32 ).
The level of physical activity was obtained using the validated International Physical Activity Questionnaire for Adolescents (IPAQ-A), adapted and validated for the HELENA study( Reference Hagstromer, Bergman and De Bourdeaudhuij 33 ). The IPAQ-A covers four physical activity domains during the whole week: school-related physical activity (including activity during physical education classes and breaks), transportation, housework and activities during leisure time. Activities were later classified into low, moderate and vigorous activities on the basis of guidelines for data processing and analyses of the IPAQ-A( 34 ). Total time spent on moderate-to-vigorous activity was summed and truncated to avoid overestimation( Reference Haerens, Deforche and Maes 35 ).
Statistical analyses
Population characteristics are presented as means and standard deviations unless otherwise stated. All analyses were stratified by sex. Baseline characteristics were compared between sexes using Student’s t test or the Mann–Whitney U test for continuous variables and by Pearson’s χ 2 test for categorical variables. Tests for normality were performed using the Kolmogorov–Smirnov test. B-vitamin biomarkers, fasting lipids and serum PL FA-related variables were logarithmically transformed to obtain a normal distribution.
Associations between either fasting lipid profile or serum PL FA and B-vitamin biomarkers (vitamin B6, folate, vitamin B12 and tHcy as independent variables) were tested with linear mixed-effects models to control for study design (clustering of cases within cities), including z-scores of BMI, DQI, maternal education and physical activity. Associations are presented in terms of change in 10 units of the independent variables (B-vitamin biomarkers) to ease interpretation and to present biologically relevant results. A coefficient lower than 10 implies a negative association, whereas a coefficient higher than 10 implies a positive one. In addition, single univariate tests controlling for the false discovery rate, using the widely agreed 0·25 cut-off point( Reference McDonald 36 ) with the Benjamini–Hochberg method( Reference Benjamini and Hochberg 37 ), were performed to control for the type I error inflation associated with multiple testing. Significance was established according to the new P values obtained after applying the Benjamini–Hochberg method. PASW 20.0 for Windows (IBM SPSS Inc.) was used to run the analyses.
Results
Several significant differences in terms of descriptive characteristics were observed between included and excluded participants (data not shown). In the whole HELENA study sample, males represented 48 %, whereas for the sample included in this analysis males represent 43 %. For instance, included males had significantly lower BMI and higher DQI scores, and there were significant differences in terms of maternal education (P<0·001) as compared with those excluded. No differences were observed in females. Included males had lower levels of DHA (P<0·05), whereas included females had higher levels of palmitoleic acid and palmitoleic:palmitic ratio (P<0·05). Both male and female participants had higher levels of α-linolenic fatty acid compared with adolescents excluded from the analysis. No differences were found between included and excluded adolescents regarding B-vitamin biomarkers.
Table 1 presents adolescents’ characteristics stratified by sex. Females had significantly higher DQI scores and lipid parameters as opposed to males. Males had significantly higher weekly moderate-to-vigorous physical activity, lower levels of serum vitamin B12, and higher PLP and tHcy levels (P<0·01). The mean palmitoleic:palmitic acid ratio was identical for both sexes.
Table 1 Characteristics of the study participants (Mean values and standard deviations)

DQI, Diet Quality Index; PLP, pyridoxal 5'-phosphate; PF, plasma folate; HoloTC, holotranscobalamin; tHcy, total homocysteine; PL, phospholipids; ALA, α-linolenic fatty acid.
* Values between brackets following the B-vitamin biomarkers are the number of males and females, respectively, with available information for these biomarkers and the rest of variables used in the analyses.
† P value based on t test. All other P values refer to the Mann–Whitney U test.
‡ DQI was calculated following the formula previously described( Reference Vyncke, Cruz Fernandez and Fajo-Pascual 28 ).
§ P values based on the χ 2 test.
Associations presented in Table 2 reflect changes in 10 units of the independent variables (B-vitamin biomarkers). A coefficient lesser than 10 implies a negative association. Overall, more significant associations were found between folate and vitamin B12 biomarkers and fasting lipid profile and serum PL FA status as compared with PLP or tHcy concentrations. All significant associations found with tHcy were negative, except for the oleic:stearic ratio where a positive significant association was observed in males with an increase of 10 µmol in tHcy concentrations, resulting in a decrease in the PL FA in serum. The associations found between either fasting lipid profile or serum PL FA and B-vitamin biomarkers were always in the opposite direction as those found with tHcy. Moreover, the association found between TAG and serum vitamin B12 was positive for males and negative for females. Most of the significant associations were observed between B-vitamin biomarkers and DHA, n-3 FA and n-3:n-6 ratio as the dependent variables.
Table 2 Changes in fatty acid profiles according to changes in 10 units of B-vitamin biomarkersFootnote † in HELENA adolescent males and females (controlled for the influence of the centre as random effect) (β-Coefficients with their standard errors)

PLP, pyridoxal 5'-phosphate; PF, plasma folate; HoloTC, holotranscobalamin; tHcy, total homocysteine; ALA, α-linolenic fatty acid.
* Coefficients are statistically significant based on the raw P values and using the Benjamini–Hochberg procedure with a false discovery rate of 0·25.
† Coefficients are the result of multiplying by 10 the real coefficients provided by the linear mixed model analysis including random effects for centre and represent the change resulting from an increase of 10 nmol/l or pmol/l of indicated B-vitamin biomarker. Analyses are adjusted by z-scores of BMI, Diet Quality Index, physical activity and maternal education level and false discovery rate control by the Benjamini–Hochberg method (refs).
Discussion
To our knowledge, this is the first study investigating the associations between B-vitamin biomarkers and serum PL FA in a large sample of adolescents and one of the few available in humans. Our results primarily showed that folate and vitamin B12 biomarkers and homocysteine concentrations were associated with serum PL FA, mainly with the n-3 pathway. Evidence of associations in the literature is unclear and often debatable. Some studies have reported associations between B-vitamin biomarkers and PUFA both in animals( Reference van Wijk, Watkins and Hageman 15 , Reference Cabrini, Bochicchio and Bordoni 38 ) and in humans( Reference van Wijk, Watkins and Hageman 15 , Reference Zhao, Lamers and Ralat 18 , Reference Bertrandt, Klos and Debski 39 ), whereas others have failed to observe any associations in elderly and pregnant women( Reference Crowe, Skeaff and McMahon 16 , Reference Dullemeijer, Durga and Brouwer 40 ). However, this is the first time that such associations are explored in a population of healthy adolescents. It is of great importance to further investigate such associations in future studies because of their role in CVD. It would also be relevant to evaluate the link between both B-vitamins and FA and DNA methylation and future conditions such as cancer or other metabolic diseases( Reference de la Rocha, Pérez-Mojica and León 41 ).
Another HELENA-based subanalysis by Vyncke et al.( Reference Vyncke, Huybrechts and Van Winckel 42 ) showed that food intake could explain a limited variance of serum PL FA, being maximal for the n-3 FA (14·2 %). We have also observed that the highest variance of B-vitamin concentrations explained by the habitual combination of foods consumed by a subject or a population group, referring to the dietary patterns( Reference Willett 43 ), consists of only 17 % (unpublished results).
In previous studies, the DQI showed positive associations with HoloTC and with n-3 FA( Reference Vyncke, Cruz Fernandez and Fajo-Pascual 28 ). In our study, associations between B-vitamin biomarkers, FA and lipids fraction were adjusted for DQI scores to mitigate the effect of diet in observed associations. However, we cannot preclude that some of the observed associations may be due to the combination of both B-vitamins and FA contained in the same food items. For instance, previous analyses showed that fish products are important contributors of both DHA( Reference Vyncke, Libuda and De Vriendt 44 ) and folate( Reference Iglesia, Mouratidou and González-Gross 45 ), and such products were found to be positively associated with the DQI, whereas less healthy foods showed negative associations. In this sense, it is important to consider the DQI dietary equilibrium component as it might influence negatively the total score if a food group is consumed in excess( Reference Vyncke, Cruz Fernandez and Fajo-Pascual 28 ).
Two physiological pathways that may explain such associations have been described( Reference van Wijk, Watkins and Hageman 15 , Reference Zhao, Lamers and Ralat 18 ). However, despite the plausibility of both mechanisms, the specific metabolic pathway linking both B-vitamins and FA has not been clearly established. First, B-vitamins act as cofactors in the methylation process as described in Fig. 2. The methylation of PE to PC by phosphatidylethanolamine methyltransferase (PEMT) requires the methyl donor S-adenosylmethionine (SAM). This reaction is inhibited by S-adenosyl-l-homocysteine (SAH). SAH is hydrolysed to homocysteine throughout a reversible reaction: homocysteine will result in increased SAH, thereby inhibiting PEMT( Reference van Wijk, Watkins and Hageman 15 ). In the liver, PC is crucial for the transport of PUFA in the blood, particularly DHA.

Fig. 2 One-carbon cycle: interactions between B-vitamins and DHA (adapted from Kulkarni et al.( Reference Kulkarni, Dangat and Kale 46 )).
Nevertheless, in a randomised controlled trial (RCT) of 253 elderly participants( Reference Crowe, Skeaff and McMahon 16 ), results did not support this mechanism, as the proportion of EPA, docosapentaenoic acid and DHA acids in plasma PC did not differ between the B-vitamin supplementation and the placebo groups after 2 years of intervention. This lack of agreement in the results could be due to differences in the study design (RCT against cross-sectional) and population group (elderly against adolescents), as several studies have suggested that n-3 PUFA metabolism changes with age, provided that Δ6 desaturase (a PLP-dependent enzyme) decreases with age( Reference Watson and De Meester 47 ). This fact would affect the second proposed mechanism.
The second plausible mechanism is based on the impairment of hepatic Δ6 desaturase activity. The Δ6 desaturase is a PLP-dependent enzyme, required for the interconversion of high-unsaturated fatty acids (HUFA)( Reference Zhao, Lamers and Ralat 18 ) into PUFA such as arachidonic acid (20 : 4 n-6) and DHA (22 : 6 n-3). This was also observed in one in vitro study with cultured cells( Reference Zhao, Ralat and da Silva 48 ) in which a reduction in vitamin B6 levels led to changes in the n-3 and n-6 long-chain PUFA profile in parallel with reductions in the rate of the desaturation processes. They also found that concentrations of total FA and PL species were higher in vitamin B6-deficient cells, as it was also observed in some studies in rats( Reference Audet and Lupien 49 , Reference Sabo, Francesconi and Gershoff 50 ). This might be because of the reduction in FA oxidation rates and their accumulation into cells because of the impairment of carnitine whose synthesis might be affected in case of vitamin B6 deficiency( Reference Cho and Leklem 51 ).
In view of the observed associations, this second pathway is less likely to explain our results, as more consistent associations were found for folate and vitamin B12 biomarkers than for PLP (vitamin B6 biomarker). However, it is suggested elsewhere( Reference Zhao, Ralat and da Silva 48 ) that this pathway is more probable than the first one as SAM concentrations were higher, but SAH was lower in the cultured cells with vitamin B6 restriction. However, the study did not consider the interaction between folate and vitamin B12.
In our study, the pro-inflammatory index n-6:n-3 PUFA ratio was significantly lower in adolescents with higher concentrations of B-vitamin biomarkers; however, the n-6:n-3 PUFA ratio was significantly higher in those with higher tHcy concentrations. PLP concentrations were also reported to be inversely associated with C-reactive protein among other pro-inflammatory markers, independent of plasma homocysteine concentrations( Reference Sakakeeny, Roubenoff and Obin 52 ). This association has been speculated to reflect the mobilisation of this coenzyme into inflammatory sites. Nevertheless, the underlying mechanisms remain unclear.
It is worth noticing that we observed an inverse association between PF concentrations and trans-FA. Trans-FA can inhibit the activity of Δ6 desaturase in the liver and subsequently decrease the efficacy of HUFA interconversion( Reference Zhao, Lamers and Ralat 18 ) into PUFA. Trans-FA are a special type of unsaturated fatty acids with one double bond in the trans position and they can be naturally or industrially present in food products. Their presence in food products is highly variable (from 1 to 50 % industrially, to 2 to 9 % naturally)( Reference Moratidou, Saborido and Wollgast 53 ). Because of the established association between trans-FA and CVD( Reference Martin-Saborido, Mouratidou and Livaniou 54 ), the food industry has drastically decreased the content of these trans-FA in their products in the last decade, but there are still some European countries, especially in Eastern Europe, where levels are still elevated( Reference Moratidou, Saborido and Wollgast 53 ). Our study was performed in 2006; at the time, the vast majority of trans-FA intakes came from industrial products and their level in blood was already low. The levels of these FA would be even lower, and it is very likely that such an association would not have been found if our study was reproduced today.
The ratios product:precursor of 18 : 1n-9cis:18 : 0 (oleic:stearic) (oleoyl-CoA desaturase activity) and 16 : 1n-7cis:16 : 0 (palmitoleic:palmitic) (stearoyl-CoA desaturase activity) measured in plasma PL are integrated markers of SFA intake( Reference Hernández Rodríguez and Gallego 55 ). These ratios are relevant as they are associated with increasing risks of obesity, diabetes or cancer( Reference Caron-Jobin, Mauvoisin and Michaud 56 , Reference Mosconi, Agradi and Gambetta 57 ). We found positive associations of oleic:stearic acid with homocysteine and negative associations with PF and the biomarkers of vitamin B12 in males. This might be because of the fact that B-vitamins play a role as cofactors in the metabolism of sulphur-containing amino acids such as homocysteine and these are related with lipid metabolism as reported in several studies( Reference Poloni, Blom and Schwartz 58 ); however, this explanation needs to be confirmed. Recently, several studies have suggested that sulphur-containing amino acids modulate the expression of stearoyl-CoA desaturase-1, a key enzyme in the hepatic synthesis of MUFA( Reference Hodson and Fielding 59 ).
Considerations of the study
This study has several strengths including the use of harmonised, standardised and validated tools and procedures in a large sample of European adolescents( Reference Hooper, Thompson and Harrison 12 ). Another important strength is the availability of B-vitamin biomarkers, accepted in the literature (PLP, PF, RBC-folate, HoloTC, tHcy) or widely used (serum B12)( Reference Gonzalez-Gross, Benser and Breidenassel 22 ), which accurately reflect B-vitamins’ status and complement dietary assessment methods. Moreover, all these biomarkers were centrally analysed, increasing the accuracy of the laboratory results( Reference Beghin, Castera and Manios 21 ). Finally, the HELENA study sample size was calculated on the basis of the variance of BMI, which is a variable with the greatest variability in studies assessing the nutritional status in adolescents, which reinforces the representativeness of the data. Besides, different geographical areas across Europe and different socio-demographic groups were recruited. Sexual maturation of this sample of European adolescents has been previously described( Reference Beghin, Huybrechts and Vicente-Rodriguez 20 ), and the distribution of Tanner( Reference Tanner and Whitehouse 60 ) categories, both in males and females, was between the third and the fifth stage. Therefore, our results could be extrapolated to similar groups of healthy adolescents living in European cities.
On the other hand, the present study has several limitations. The sources of trans-FA isomers (natural or industrial) were not analysed in this study, and therefore we can only consider trans-FA in total, and the authors assume that the majority came from industrial sources. Similarly, choline, which might also influence our results, was not measured.
In addition, the reduction of the sample from the original 1089 adolescents with blood samples, due to the unavailability of the variables of interest for this analysis, poses a study limitation. Besides, the cross-sectional design of the study does not allow us to establish cause–consequence relationships from the variables of interest.
Only as a consideration, previous reports based on the HELENA study have shown that the association between FA intake and plasma FA cannot be assumed, except for several long-chain n-3 PUFA( Reference Vyncke, Huybrechts and Van Winckel 42 ). In fact, it seems that plasma FA concentrations in adolescents could be seldom explained by diet, being maximal at 14·2 % for the n-3 FA. This lack of association between FA intake and biomarkers may be explained by the different routes followed by FA once metabolised in the human body (oxidation, elongation and desaturation, to be incorporated in cell membranes, or stored in the adipose tissue( Reference Vyncke, Huybrechts and Van Winckel 42 )). This is why we have used the DQI as a covariate to control for diet instead of FA intake. In addition, a better overall dietary quality, assessed with the DQI, was associated with a higher total fat intake (expressed as % energy) in males and females in a previous study( Reference Vyncke, Huybrechts and Dallongeville 61 ).
Conclusion
B-vitamin-related biomarkers, especially those corresponding to folate and vitamin B12, were positively associated with serum PL FA, mainly with the n-3 pathway, whereas these associations were negative with homocysteine levels. Among the possible mechanisms that might explain our results, the influence of these vitamins in the conversion of PE to PC, which is the main membrane PL in transporting actively the PUFA, seems to be the most likely route. However, additional experiments are required in which additional parameters should be measured – for example, plasma and tissue levels of SAM or SAH.
To conclude, our findings suggest that higher B-vitamin biomarker concentrations might be related to higher PUFA concentrations (mainly n-3 series). Besides, the pro-inflammation marker n-6:n-3 PUFA ratio decreased with increasing values of B-vitamin biomarkers, as with trans-FA (probably because of different dietary sources in this case) and the oleic:stearic acid ratio. Consequently, assuring a good status of B-vitamin biomarkers is very important as it might influence cardiovascular health by lowering homocysteine levels, but also by increasing circulating n-3 PUFA and decreasing the pro-inflammatory marker n-6:n-3 PUFA ratio.
Acknowledgements
The authors acknowledge all the adolescents who made possible the HELENA study with their participation. Many thanks to Petra Pickert, Anke Carstensen, Rosa Torres, Ulrike Albers and Andre Spinneker for their contribution to laboratory work.
The HELENA study received funding from the European Union’s Sixth RTD Framework Programme (contract FOODCT-2005-007034). Additional support was obtained from the Spanish Ministry of Education (AGL2007-29784-E/ALI), Axis-Shield Diagnostics Ltd (Oslo, Norway), Abbot Científica S.A. (Spain). This analysis was also supported by the Spanish Ministry of Science and Innovation (JCI-2010-07055) with the contribution of the European Regional Development Fund (FEDER). The first author is supported by a grant from the Spanish Carlos III Health Institute: RD08/0072/0025 (Red SAMID: Maternal, Child Health and Development Research Network).All the authors listed have contributed to the study, and all authors have read, approved and agreed to submit the manuscript to Br J Nutr. Besides, all the authors have contributed substantially to this study either in formulating the research question (RQ), design of the study (DS), carrying it out (C), analysing the data (AD) or writing the article (WA) as follows: I. I. (RQ, C, AD WA), I. H. (C, AD, WA), M. G.-G. (DS, C, WA), T. M. (WA), J. S. (AD, WA), V. C. (RQ, AD), E. M. G.-G. (AD, WA), J. Y. P. (RQ, AD), S. B.-S. (AD, WA), M. C.-G. (AD, WA), M. C. (DS, C, WA), M. K. (DS, C, WA), K. W. (DS, C, WA), S. D. H. (DS, C, WA), M. S. (DS, C, WA), F. G. (DS, C, WA), D. M. (DS, C, WA), Y. M. (DS, C, WA), A. K. (DS, C, WA), M. F. (C, WA), P. S. (DS, C, WA), A. M. (DS, C, WA), F. J. S.-M., F. J. (RQ, AD, WA), and L. A. M. (RQ, DS, C, AD, WA).
The authors declare that there are no conflicts of interest.
Supplementary material
For supplementary material/s referred to in this article, please visit https://doi.org/10.1017/S0007114516004414