Introduction
Coastal environments have long been favoured for human migration and settlement. For millennia, these locations have provided access to resources and well-connected places for exchange and defence (Dawson et al. Reference Dawson2020). Reflecting the maritime traffic that connected these places, an estimated three million shipwrecks lie on the floors of the world's oceans (UNESCO 2020). This underwater heritage affords a unique window into humanity's common past and collective memory. In a similar way, thousands of submerged prehistoric sites around the world—inundated by post-glacial sea-level rise of up to 130m—attest to earlier phases of human adaptation to climate change (Bailey et al. Reference Bailey, Harff and Sakellariou2017).
Successive reports published by the Intergovernmental Panel on Climate Change, or IPCC, have drawn attention to a growing range of interrelated climate phenomena affecting the world's oceans. The IPCC First Assessment Report (IPCC 1990) documented an international consensus that, by the end of the twenty-first century, sea levels were predicted to rise by approximately 1m, together with an increase in ocean surface temperatures (i.e. water to a depth of approximately 100m) of between 0.2 and 0.5°C. The IPCC Fourth Assessment Report (IPCC 2007) additionally recognised ocean acidification, with projections of a reduction in average global surface ocean pH of between 0.14 and 0.35 by the end of the century. The Fifth Assessment Report (IPCC 2014) identified the increased frequency of storm surges, resulting from sea-level rise and changing ocean circulation, as another effect of climate change. Most recently, the special report of 2019 has drawn attention to ocean deoxygenation and the increasing speed at which glaciers and ice sheets are melting as other major consequences of climate change (IPCC 2019).
The potential impacts of these changing climatic and environmental processes on the future preservation and management of coastal and underwater cultural heritage have been broadly discussed (e.g. Rowland Reference Rowland1992; Westley et al. Reference Westley, Bell, Renouf and Tarasov2011; Daire et al. Reference Daire2012; Rowland & Ulm Reference Rowland and Ulm2012; Hambrecht & Rockman Reference Hambrecht and Rockman2017; see references 1–15 in the online supplementary material (OSM)). As all these studies recognise, it is the complexity and interrelatedness of physical, chemical and biological processes—all exacerbated by human activity—that makes it difficult to determine direct links between climate change and the condition of maritime heritage. As a result, it is particularly challenging to understand the cumulative effects of these processes on archaeological materials and their immediate environments, and to differentiate the ‘signal’ from the ‘noise’ (Cheng & Gaskin Reference Cheng and Gaskin2011: 48). This situation stresses the need for more interdisciplinary work, with collaboration between heritage professionals and researchers from related disciplines. By integrating the material and natural sciences to monitor and quantify deterioration processes in coastal and marine environments, for example, it may be possible to implement sustainable management strategies. Currently, however, the archaeological community needs to do more to communicate with other researchers, as well as the public, about the fundamental importance of cultural heritage—both as a resource to be protected and as an important source of information about past climate change. For instance, in discussing the UN Decade of Ocean Science for Sustainable Development, Wisz and colleagues (Reference Wisz2020) do not include a single mention of cultural heritage. As well as better protecting maritime cultural heritage, addressing this situation brings benefits for a range of stakeholders. Integrating coastal and underwater cultural heritage with climate science has the potential to: contribute to the ocean or ‘blue’ economy (that is, the sustainable use of ocean resources for economic growth and improved livelihoods and jobs, while preserving the health of ocean ecosystems (World Bank 2017)); inform studies of the long-term durability of archaeological materials, which can provide insights into the biodeterioration and corrosion of modern materials; and offer direct and indirect information on past climate change and human adaptation (Landquist et al. Reference Landquist2013; see references 16–20 in the OSM).
As a contribution to this objective, the present article provides an overview of the literature specifically related to the effects of climate change on coastal and underwater cultural heritage, drawing on a range of international case studies. These examples are discussed within the wider context of current practices in archaeology and cultural resource management. In addition, we also discuss how natural threats to maritime heritage are inextricably intertwined with direct human (anthropogenic) impacts, both in coastal locations and on the seabed. Finally, examples drawn from other coastal and marine sciences relevant to the issues of deterioration, and hence preservation, are introduced in order to highlight the need for greater inter- and cross-disciplinary collaboration through which to support maritime cultural heritage management.
Key impacts to coastal archaeological sites
Sea-level rise
By 2100, sea-level rise is projected to be 0.29–1.10m across more than 95 per cent of the world's oceans, progressing at rates of 4–15mm/year, depending on atmospheric greenhouse gas concentrations (IPCC 2019) (Figure 1). Due to the unpredictability of ice-sheet dynamics (particularly in Antarctica), however, researchers advise that such predictions should be used with caution. Sea-level rise can increase the severity of extreme ocean events, such as exceptionally high tides, storm surges and unpredictable weather patterns, affecting both coastal and nearshore sites. In turn, tidal rivers can extend far inland the effects of coastal flooding caused by both the short-term combination of surges, tides and waves and long-term increases in sea level (Carmichael et al. Reference Carmichael2017). Recent research also highlights the role of sea-level rise in the greater frequency of extreme sea-level events (Vousdoukas et al. Reference Vousdoukas2018, Reference Vousdoukas2020), potentially driving accelerated coastal retreat in many parts of the world. The higher frequency of such events increases the likely occurrence of both short- and long-term flooding and coastal erosion.

Figure 1. Regional sea-level change for RCP2.6, RCP4.5 and RCP8.5 in metres for extreme sea-level (ESL) events. RCP = representative concentration pathway (after IPCC 2019: fig. 4.10).
Coastal erosion
Coastal erosion may destroy heritage sites gradually over decades or cause catastrophic loss during a single event (Dawson et al. Reference Dawson2020). Along the Libyan coast, for example, major settlements dating to the Greek, Roman and Byzantine periods are being damaged by both types of erosion (Figure 2), including parts of the World Heritage Site of Sabratha (Brooks et al. Reference Brooks, Clarke, Wambui Ngaruiya and Wangui2020), the ancient Greek city of Taucheira at Tocra, and the harbour of Apollonia in Cyrenaica, where the erosion of a protective natural reef has left the ancient harbour at risk (Bennett Reference Bennett2018). On the coast of East Africa, meanwhile, towns and villages associated with the Swahili Indian Ocean trade network are at severe risk. Many sites along the coasts of Kenya, Tanzania, Mozambique, Zanzibar and Madagascar were built in low-lying locations on coral or mud foreshores that are vulnerable to flooding and erosion caused by surges and long-term sea-level rise (Brooks et al. Reference Brooks, Clarke, Wambui Ngaruiya and Wangui2020). Similar coastal erosion is affecting archaeological sites in many other parts of the world, such as Cyprus (Andreou Reference Andreou2018) and the pre-Columbian ‘mud city’ of Chan Chan in Peru (Colosi et al. Reference Colosi2009; Pierdicca et al. Reference Pierdicca2016).

Figure 2. Major settlements dating to the Greek, Roman and Byzantine periods that are at risk along the eroding coastline of eastern Libya: A) Apollonia (photograph taken November 2019 by S. Buyadem); B) Tocra (photograph taken November 2019 by S. Alaurfi).
Severe storms occur in mid-latitude regions, contributing to much of the coastal erosion experienced along the Atlantic coast of North America (Greenland Reference Greenland and Schwartz2005). High winds can create enormous waves and storm surges that overflow natural and artificial coastal margins, leading to flooding and saltwater incursion. Severe storms can erode many tens of metres of land. In 2005, for example, a large part of a prehistoric settlement on the island of Baile Sear in the Outer Hebrides of Scotland was destroyed when the sandy coast retreated overnight by as much as 50m, leading to the collapse of structures, the exposure of archaeological deposits and the dispersal of cultural material along the beach (Dawson Reference Dawson2015). This storm was associated with a deep atmospheric depression, and the resultant low pressure led to an increase in sea level of 0.69m—enough to rise over the natural coastal protection afforded by an offshore rock platform and seaweed forest (Angus & Rennie Reference Angus and Rennie2014).
Hurricanes and typhoons
Tropical cyclones form, in part, as a result of high sea surface temperatures, usually inflicting the greatest damage in coastal areas and weakening if they move inland (Greenland Reference Greenland and Schwartz2005). In September 2017, Hurricanes Irma and Maria struck the Caribbean and the southern United States in quick succession. Several Caribbean islands were affected, including Barbuda and Puerto Rico, which suffered catastrophic damage to houses, infrastructure and heritage sites (Rivera-Collazo Reference Rivera-Collazo2020).
Strong winds can also cause coastal sands to move, burying some sites and creating deflation hollows within dune systems that damage the integrity of archaeological remains (Rick Reference Rick2002). Soils in coastal areas often have higher concentrations of salt, either carried inland on the wind or due to saltwater intrusion; this salinity can affect standing structures and buried remains, and can also damage coastal vegetation, all of which may have historical significance for Indigenous peoples (Sesana et al. Reference Sesana2021). There is also a growing risk to maritime heritage of pluvial flooding due to increased precipitation in both coastal and inland areas, especially during intense storms. This can be particularly problematic if the egress of river water is blocked at the coast by sea levels elevated by surges or tides; the catastrophic 1966 Venice flood, for example, was partly caused by intense rainfall inland across north-east Italy combined with a high tide and strong winds (Trincardi et al. Reference Trincardi2016).
Key impacts to underwater cultural heritage
Seabed erosion
Shipwrecks and submerged prehistoric sites and landscapes are also susceptible to erosion caused by tidal currents and wave action, the latter being affected by changing storm and weather patterns. Research has been directed predominantly towards the use of marine geophysical techniques for mapping and monitoring changes to the seabed around archaeological sites, principally shipwrecks (Quinn Reference Quinn2006; see references 21–25 in the OSM) (Figure 3). Many underwater sites, however, have not been subject to such geophysical investigation, and thus they lack a baseline record against which to measure change. This situation makes it difficult to disentangle natural seabed dynamics from climate change-induced variations. A potential way forward is to model the complex physical processes affecting underwater sites by using computational fluid dynamics, as exemplified in recent work on shipwreck sites (Smyth & Quinn Reference Smyth and Quinn2014; see references 26–27 in the OSM). While modelling, however, can be used to demonstrate the impact on the seabed of changing winds and currents, and the influence of bathymetry and coastal configurations on local hydrodynamics, projections of future storm patterns often have low levels of confidence and strong regional variability (IPCC 2019). As with the coastline, human modification of the seabed (by, for example, offshore infrastructure, such as wind turbine foundations) can also cause disruption to local sediment dynamics (e.g. Vanhellemont & Ruddick Reference Vanhellemont and Ruddick2014).

Figure 3. Sediment dynamics at different timescales around the wreck of the SS WM Barkley (torpedoed and sunk October 1917 in the Irish Sea): a) bathymetry showing the wreck lying in a scour pit amidst a field of sand waves; b–d) difference model created from MBES surveys: b) one week apart in 2019, c) nine years apart (2010 and 2019) and d) one year apart (2015 and 2016). DoD = difference in seabed elevation between surveys (image created by J. Majcher, Ulster University).
Temperature
Global sea temperatures rose between 0.5 and 1°C during the twentieth century and, over the last three decades, the world's oceans have reached their highest-ever recorded temperatures. Furthermore, the most recent IPCC report indicates not only an impact on surface ocean temperatures but also on the deep ocean, with increases of 0.6–2.0°C in the top 100m of the water column and 0.3–0.6°C at depths of 1000m by the end of this century (IPCC 2019). Such temperature changes will affect physical processes in both the atmosphere and the oceans. Warmer seas lead to more energetic climate conditions expressed as changing weather patterns, including shifts in rainfall, air temperatures and wind systems. These changes reflect the highly complex interaction of physical, chemical, biological and anthropogenic processes, which complicate evaluation of direct and indirect impacts on coastal and underwater cultural heritage.
As a rule of thumb, chemical reaction rates double for every 10°C increase in temperature. If we consider iron corrosion in the marine environment, however, a rise of 30°C is required to double the corrosion rate (Melchers Reference Melchers2002), yet this higher resistance to corrosion is complicated by other factors. Researchers at the Western Australian Museum have pioneered the monitoring of metal wreck sites (Macleod Reference Macleod2006; see referenecs 28–30 in the OSM). Their research indicates that the average long-term corrosion rate for iron in aerobic seawater is approximately 0.1mm per year. Based on this, and using the current worst-case models for temperature increase (2°C) over the next 100 years, we may expect only a relatively small increase in corrosion rates. Temperature change, however, is only one part of a complex process; other physical, chemical and biological properties of seawater affect the corrosion rates of different metals, including: the ratios of the concentrations of the major constituents; the presence of chloride; high electrical conductivity; pH; solubility of gases, especially oxygen and carbon dioxide (CO2); and the types of bacteria present and their resulting effect on the corrosion rate (Salazar & Little Reference Salazar and Little2017).
Wood-boring bivalves
Temperature change also has an indirect effect on the habitat and ecology of marine flora and fauna. Of particular concern is the spread of wood-degrading organisms due to increasing temperature. A recent study of the diversity, environmental requirements and biogeography of wood-boring bivalves in European coastal waters identified a total of nine species of teredinid molluscs, commonly known as shipworms, with various distributions (Borges et al. Reference Borges, Merckelbach, Sampaio and Cragg2014). Of these nine species, Nototeredo norvagica was found to have the widest distribution in European waters. Teredo navalis, or the naval shipworm, was reported to be present as far as the eastern Baltic but only sporadically along the Atlantic coast of Southern Europe. In the latter area, the Atlantic lineage of the blacktip shipworm (Lyrodus pedicellatus), which has been a species of particular concern in relation to underwater cultural heritage over the last decade, was found to be the dominant teredinid. Meanwhile, in the Mediterranean, the study found six sympatric (or geographically overlapping) teredinid species, three of which were also found in the Black Sea. Borges and colleagues (Reference Borges, Merckelbach, Sampaio and Cragg2014) concluded that, of these nine species, T. navalis and L. pedicellatus pose the greatest hazard to wooden maritime structures in European coastal areas (Figure 4).

Figure 4. X-ray of modern wooden pine blocks after submergence for one year in the Atlantic estuarine waters of the Bou Regreg River, Morocco, showing tunnelling by Teredo navalis (large tunnels) and Lyrodus pedicellatus (smaller and more abundant tunnelling) (photographs by M. Taube, National Museum of Denmark).
Dissolved oxygen
The concentration of dissolved oxygen in seawater is a key parameter influencing both corrosion rates and the species of marine flora and fauna present, including all wood-boring organisms. Dissolved oxygen in coastal waters is declining globally; since the mid twentieth century, 1–2 per cent of the global ocean oxygen inventory has been lost and over 700 coastal regions worldwide have now reported reductions in oxygen concentrations (Limburg et al. Reference Limburg, Breitburg, Swaney and Jacinto2020). Hypoxia, or low oxygen levels, occurs naturally in many marine and freshwater systems that are characterised by high microbial productivity and stratification of the water column. In general, climate warming decreases oxygen solubility due to higher water temperature, which, in turn, increases stratification and enhances the respiration processes of anaerobic bacteria. In addition, human activities, including intensive agricultural practices, land-use changes and point-source nutrient loading (e.g. via sewage outlets), have caused the frequency, magnitude and extent of coastal hypoxia to increase. Higher terrestrial nutrient inputs stimulate the growth and export of organic material to deeper waters, thereby disrupting the natural balance between oxygen supply from physical processes and oxygen demand from the consumption of organic material. As such, hypoxia would undoubtedly benefit underwater cultural heritage by limiting the growth of wood-boring organisms and by decreasing metal corrosion rates. This irony is one of the challenges we face: what may be advantageous for the sustainable preservation of underwater cultural heritage is unsustainable for the environment as a whole.
Acidification
Ocean acidification, resulting from dissolution of atmospheric anthropogenic CO2 in seawater, represents an additional emerging global threat to the health of marine ecosystems. Coastal ocean waters undergo natural pH fluctuations on daily, seasonal and inter-annual scales. Higher levels of atmospheric CO2, however, have led to increases in the ocean surface CO2 concentrations, resulting in lower, or more acidic pH levels (Dupont & Pörtner Reference Dupont and Pörtner2013). Since the Industrial Revolution, the acidity of the surface ocean has increased by 30 per cent, with a resulting decrease of 0.1 pH units. An additional doubling or tripling in acidity may occur by 2100 as the oceans absorb more of the CO2 released by anthropogenic activities driven by population growth and rising living standards. The direct effects of a decrease in ocean pH (i.e. increased acidity) include the potentially accelerated corrosion rates of metal shipwreck sites. Indirectly, however, lower pH levels affect a wide range of biological processes associated with the growth, reproduction and survival of organisms that can both colonise and degrade underwater cultural heritage (Boyd Reference Boyd2011; see references 31–33 in the OSM).
Caution should be exercised, however, as it is the “interrelatedness of everything” (Sesana et al. Reference Sesana2021: 10) that influences deterioration processes. Taking metal corrosion rates as an example, the influence of one parameter on the overall rate is interdependent on the concentration of other variables. Chloride ion concentrations increase corrosion rates, but increases in carbonate ion concentrations assist in the formation of semi-permeable calcareous deposits on metal surfaces, hence reducing overall corrosion rates (MacLeod Reference Macleod2006). Moreover, archaeological iron alloy artefacts in the marine environment are not only affected by changes in water chemistry and biology. The impact of the 2015 super typhoon Soudelor on Second World War vehicles submerged around the coast of Saipan in the Mariana Islands, provides a good example. Here, corrosion monitoring of two Daihatsu landing craft located 100m apart at similar depths demonstrates that the landing craft with very little surviving metal (Figure 5) was much more badly affected by the typhoon than the better-preserved landing craft, which remained essentially intact.
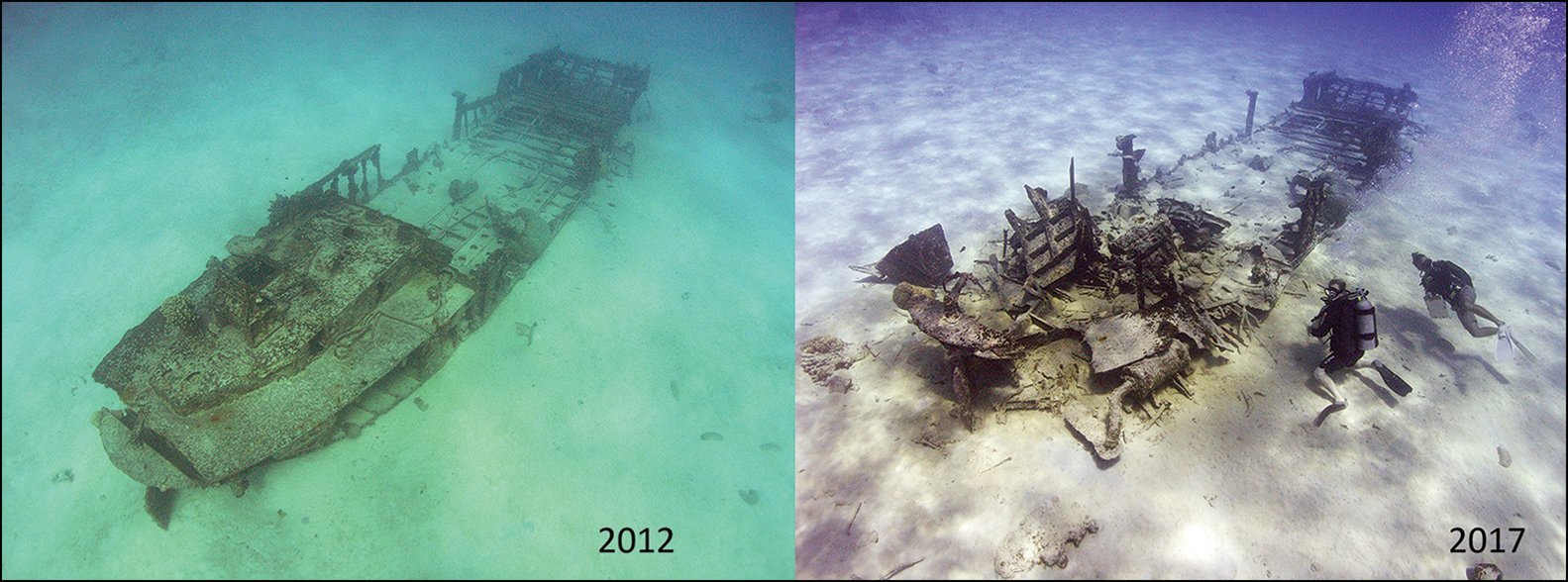
Figure 5. Daihatsu landing craft in Saipan in 2012 vs 2017 (after super typhoon Soudelor hit the Philippines and Saipan in 2015) (photographs by J. Carpenter, Western Australian Museum).
Managing coastal and underwater cultural heritage at risk of destruction
Strategies for the mitigation of the destructive impacts outlined above, and for the wider management of coastal and underwater cultural heritage, constitute a major area of research, and only a summary can be provided here. The concept of Integrated Coastal Zone Management (ICZM) was developed during the 1990s (Clark Reference Clark2018). Building on the ICZM concept, there has been extensive research into the management of coastal heritage. The global distribution of research into this concept includes studies on Australia, the Caribbean, Canada, France, India, Iran, Mediterranean countries, the UK and the USA, with a bias towards North America and Europe (e.g. Murphy et al. Reference Murphy, Thackray and Wilson2009; Daire et al. Reference Daire2012; Rowland & Ulm Reference Rowland and Ulm2012; see references 34–43 in the OSM). Coastal heritage has been integrated into some national shoreline management plans, applying the concept of ‘risk management’. These plans, intended to advance sustainable approaches to managing flood and coastal erosion risks, often propose one of four options for managing any particular stretch of coast (Murphy et al. Reference Murphy, Thackray and Wilson2009): 1) maintaining the existing coastline; 2) advancing the line; 3) managed realignment; and 4) no active intervention. The problem for heritage sites specifically is that, once lost to the sea, they cannot be reinstated, and so several of these options are problematic and alternative approaches will be needed.
In order to develop effective management strategies, some national heritage agencies have sought to document the impacts of coastal processes by undertaking rapid coastal surveys in vulnerable areas. Such baseline surveys increasingly use remote-sensing techniques, including satellite imagery, aerial photography, multispectral and drone imagery, together with photogrammetry, to document and monitor sites, and GIS to model processes and threats (Daire et al. Reference Daire2012; see references 44–47 in the OSM). There is growing recognition, however, of the need for prioritisation to deal with the large numbers of threatened sites, especially given the limited resources available (Berenfield Reference Berenfield2015; Rockman et al. Reference Rockman2016). There is also increasing acknowledgment that involving the public in heritage recording, including the assessment of condition, vulnerability and even the significance of sites, is of enormous benefit (Smith Reference Smith2014; Hambly Reference Hambly2017; Wragg et al. Reference Wragg and Dawson2017).
Since the 1990s, attention has also been directed towards the management of underwater cultural heritage. European and international laws advocate that, wherever possible, underwater archaeological sites should be preserved in situ (e.g. Council of Europe 1992; ICOMOS Charter on the Protection and Management of Underwater Cultural Heritage (ICOMOS 1996); UNESCO Convention on the Protection of Underwater Cultural Heritage (UNESCO 2001)). Consequently, there has been an increase in research on the threats and deterioration processes affecting underwater cultural heritage, including shipwreck sites and submerged landscapes, and general approaches to the management of this heritage (UNESCO 2015; ICOMOS 2020; see references 48–54 in the OSM). A distinct disparity compared with coastal heritage, however, is the lack of baseline data on underwater sites. Few have been surveyed at an appropriate level of detail, and even fewer monitored to provide repeat data that are suitable for change detection.
Thus, unlike coastal archaeology, where multiple studies have demonstrated climatic and anthropogenic impacts and attempted to assess vulnerability at multiple spatial scales, the full extent and consequences of climate change on underwater sites remain to be explored systematically, beyond a few isolated case studies. Yet, as with approaches to coastal heritage, this situation is improving with increasing implementation of citizen-science projects, as exemplified by the work of the Nautical Archaeology Society, the Florida Public Archaeology Network (FPAN) and the Australian GIRT Scientific Diver project (Viduka et al. Reference Viduka2020).
Discussion
As part of a wider ecosystem, coastal and underwater cultural archaeological heritage are at risk from the effects of climate change. A wide range of physical, chemical and biological deterioration processes, or threats, already acting on these sites are being exacerbated by anthropogenic climate change, as well as by various direct human activities. Since the first IPCC report in 1990, there has been an awareness of the impact of these changes on coastal archaeology and, in many respects, there has been some significant progress in integrating the management of this heritage into coastal planning policies at national and international levels. Intensifying debate about climate change and sustainable development during the 2000s has accelerated research on the threat of climate change to cultural heritage and the historic environment generally. Yet, despite three decades of work, we still lack a coherent agenda or approach that engages all the necessary scientific disciplines and stakeholders required to study and manage sustainably the world's coastal and underwater cultural heritage. This is by no means a criticism of any specific previous work, but rather a reflection on the context and nature of how archaeological and cultural resource management has been conducted over the past 30 years. Furthermore, when compared with natural resource conservation, coastal and underwater cultural heritage still lags in terms of its integration with coastal and marine sciences, as well as planning and environmental policy-making.
The increasing availability of remote-sensing data, including open-access satellite imagery and marine environment databases, combined with the use of drones, photogrammetry and marine geophysics, can provide resource managers and heritage agencies with quantitative data about the condition of sites and the long-term effects on them of environmental changes. These data can underpin science-based decision making in order to influence appropriate site management plans and inform the prioritisation of preservation strategies, many of which reflect current national and international plans to preserve archaeological sites in situ. As a result of this growing evidence base, many practitioners propose the evaluation and management of these sites via the concepts of threat, vulnerability and risk. Threat is an event or process, such as a storm, erosion or human activity, while vulnerability is the sensitivity of a site or artefacts to that threat; risk is the negative impact of the threat to a sensitive site. Another important concept is the idea of loss: the recognition that not all sites can be saved and that there is a need to prioritise what can be preserved, based, in part, on evaluation of the relative significance of individual sites (Cook et al. Reference Cook, Johnston and Selby2019).
Discussion of the effects of climate change and the need to protect underwater cultural heritage still lag behind the progress made by coastal heritage management. As with coastal archaeology, there is a general presumption in favour of the preservation of underwater cultural heritage in situ. Regardless of whether we consider coastal or underwater archaeology, in the face of the increasing threats posed by climate change, the reality is one of ever-decreasing funding available to resource managers and agencies for the monitoring and management of these sites.
On a positive note, there has been a fundamental change in the role of the public in heritage preservation, with a significant increase in the inclusion and participation of the public in safeguarding their heritage through citizen-science projects. Additionally, heritage practitioners may find either support or better public appreciation of the threats to coastal and underwater cultural heritage through the increasing awareness of the socio-cultural value of archaeology as part of the ocean or ‘blue’ economy. The latter is aligned with economic and trade activities and a need to integrate conservation and sustainability into the wider management of the maritime domain (Konar & Ding Reference Konar and Ding2020). The need to increase awareness of the value of such heritage emphasises the necessity for more and closer interactions and collaboration between all stakeholders. The United Nations Decade of Ocean Science for Sustainable Development (2021–2030) has just commenced, with the aim of delivering scientific knowledge for the sustainable management of oceans, including coastlines. Similarly, the Ocean Decade Heritage Network (https://www.oceandecadeheritage.org; Trakadas et al. Reference Trakadas2019) endeavours to integrate archaeology and cultural heritage management within the ambitions of marine sciences. The research discussed above highlights the immensely complex and intertwined factors associated with diverse strands of marine research and management. Whether the motivation is site preservation, engaging with citizen scientists, adhering to regulatory requirements or maximising ‘blue’ economy opportunities, the key is interdisciplinary engagement between cultural heritage practitioners, marine scientists, maritime specialists and the public. Together we can take the next steps towards climate change adaptation and resilience for coastal and underwater cultural heritage resources.
Acknowledgements
The authors would like to thank Saad Buyadem, Saleh Alaurfi, Jan Majcher, Michelle Taube and Jon Carpenter for providing and assisting in the preparation of the photographs in this article.
Funding statement
This research received no specific grant from any funding agency or from commercial and not-for-profit sectors.
Supplementary material
To view supplementary material for this article, please visit https://doi.org/10.15184/aqy.2022.115.