Introduction
It is well known that fatty acids are highly diverse in terms of their metabolic and functional properties. Oleic acid (OlAc) is chemically classified as a long-chain unsaturated omega-9 fatty acid (Fig. 1). Over the last decade large numbers of studies have suggested that the physiological role of OlAc extends beyond that of an energy source and includes the regulation of cell metabolism, inflammation, tissue development and longevity (Lee & Park, Reference Lee and Park2014; Han et al., Reference Han, Schroeder, Silva-García, Hebestreit, Mair and Brunet2017). A single cis double bond at the position Δ9 gives a high capability to OlAc to solubilize lipids (Robinson & Cistola, Reference Robinson and Cistola2014). Indeed, OlAc is a major determinant of plasma membrane fluidity (Funari et al., Reference Funari, Barceló and Escribá2003), which is obviously associated with important functions including cell–cell interaction, membrane transport and signalling events. This fatty acid is capable of donating a hydrogen cation to an acceptor, and opposes oxidation or inhibits reactions brought about by dioxygen or peroxides (Choe & Min, Reference Choe and Min2009). Moreover, OlAc can directly be attached to proteins, which is known as an important step in growth and differentiation of many cell types (Rios-Esteves & Resh, Reference Rios-Esteves and Resh2013).

Figure 1 Oleic acid molecule. The organic structure of oleic acid has 18 carbons and a single cis configuration double bond on the ninth carbon (n-9 or ω-9) from the carboxyl (acid) end. This double bond is located at the centre of the molecule and causes a bend in the carbon chain which alters the physical properties and promotes fluidity. Derived from the Protein Data Bank ID: OLA. C, carbon; H, hydrogen; O, oxygen.
The main lipid classes of bovine oocytes are triglycerides, phospholipids, cholesteryl esters and free fatty acids (FFAs) which accounted, respectively, for 57.3, 16.2, 15.5 and 10.9% of the total lipid extract (Kim et al., Reference Kim, Kinoshita, Ohnishi and Fukui2001). Free and esterified OlAc are metabolically regulated and compartmentalized into discrete extracellular fluids, cell organelles and within the cytosol. Oleic acid is a major monounsaturated fatty acid in the lipid extracts of bovine, sheep, porcine (Homa et al., Reference Homa, Racowsky and McGaughey1986; Prates et al., Reference Prates, Alves, Marques, Baptista, Horta, Bessa and Pereira2013; Dunning et al., Reference Dunning, Russell and Robker2014) and human oocytes (Matorras et al., Reference Matorras, Ruiz, Mendoza, Ruiz, Sanjurjo and Rodriguez-Escudero1998), with relative proportions between 10 and 25% of total fatty acids. Moreover, the phospholipid fractions from oocytes of cattle and other ruminants contained even more OlAc ranging from 20 to 26% (Fig. 2).

Figure 2 Fatty acid composition in phospholipids from bovine, sheep and porcine oocytes. Data of bovine are the average from Zeron et al. (Reference Zeron, Ocheretny, Kedar, Borochov, Sklan and Arav2001) and McEvoy et al. (Reference McEvoy, Coull, Broadbent, Hutchinson and Speake2000) studies. Other parts are based on data of Homa et al. (Reference Homa, Racowsky and McGaughey1986). 16:0, palmitic acid; 18:0, stearic acid; 18:1, oleic acid; 18:2, linoleic acid.
Potential reproductive effects are considered as the major aspect of biomolecules functionality in an organism (Ströhle & Döring, Reference Ströhle and Döring2010). The recent identification of OlAc as a blastomeric and post-cryopreservation survival biomarker in bovine may indicate the positive effects of this fatty acid on embryo development (González-Serrano et al., Reference González-Serrano, Pirro, Ferreira, Oliveri, Eberlin, Heinzmann, Lucas-Hahn, Niemann and Cooks2013; Ferreira et al., Reference Ferreira, de Oliveira, Gonçalves and Catharino2014). Other recent studies have shown the critical role of OlAc in paracrine supporting of oocyte development and in counteracting the detrimental effect of saturated fatty acids on oocyte development. The association between fertilization and fatty acids in general has been reviewed previously (McKeegan & Sturmey, Reference McKeegan and Sturmey2011; Dunning et al., Reference Dunning, Russell and Robker2014). However, to date, there has been no dedicated review of the literature dealing specifically with potential role of OlAc in reproduction.
This review will summarize current knowledge on the role of OlAc in the modulation of oocyte and preimplantation embryo development. We will focus primarily on changes in female sex hormones production, follicular development and early embryo development that are relevant to future clinical studies.
Oleic acid in endocrine-related reproduction function
Fatty acids may act more generally and indirectly via actions in the central nervous system to alter peripheral organ function. For instance, long-term control of food intake is affected by circulating OlAc in ruminants (Ingvartsen & Andersen, Reference Ingvartsen and Andersen2000).
Pituitary control
While dietary OlAc and linoleic acid (LnAc) caused no change in basal luteinizing hormone (LH), these long-chain fatty acids reduced gonadotropin-releasing hormone-induced LH release in dairy cows (Salehi et al., Reference Salehi, Colazo, Oba and Ambrose2015). Despite the fact that the physiological significance of these effects on cattle ovulation remains to be investigated, these results suggest that concentration of circulating OlAc is important in the neuroendocrine control of reproduction, which appears to be independent of hypothalamic function and directly modulates gonadotropin release from the pituitary.
Steroidogenesis
The follicle cells and the corpus luteum in the ovary secrete the female sex hormones estrogen and progesterone, respectively, which regulate ovulation and control the reproductive cycle. These functions appear to be related to cellular fatty acid status. Notably, the amounts of unsaturated fatty acids including OlAc were significantly greater in minor plasma membrane phospholipids of the corpus luteum in pregnant ewes than in regressing corpus luteum of non-pregnant ewes at day 13 of the estrous cycle (Zelinski et al., Reference Zelinski, Selivonchick and Stormshak1988). This selective increase in the OlAc content of membrane phospholipids reflects dynamic changes in OlAc partitioning during the reproductive cycle. In addition to dietary intake, OlAc is supplied by a fine regulated de novo synthesis mechanism (Fig. 3). Endogenous synthesis of OlAc is primarily regulated by stearoyl-coenzyme A desaturase (SCD) in multiple cell types, including follicular cells (Feuerstein et al., Reference Feuerstein, Cadoret, Dalbies-Tran, Guerif, Bidault and Royere2007; Aardema et al., Reference Aardema, van Tol, Wubbolts, Brouwers, Gadella and Roelen2017; Fayezi et al., Reference Fayezi, Ghaffari Novin, Darabi, Norouzian, Nouri, Farzadi and Darabi2017). A recent study showed that prepubertal heifers versus cows had higher levels of SCD gene expression in granulosa cells and OlAc in follicular fluid (Warzych et al., Reference Warzych, Pawlak, Pszczola, Cieslak and Lechniak2017). Moreover, SCD activity was profoundly increased by follicle-stimulating hormone (FSH), which initiates and supports follicular development and regulates steroidogenesis in females (Moreau et al., Reference Moreau, Froment, Tosca, Moreau and Dupont2006).

Figure 3 Diagram illustrating the metabolic pathways for oleic acid. Free oleic acid is derived from dietary lipid intake or endogenous de novo production. The latter is dependent to desaturation reaction, which is a rate-limiting step in the oleic acid synthesis. Oleic acid can be incorporated into cellular lipids, metabolized into bioactive derivatives or catabolized to release energy.
Free OlAc supplementation at an amount of 200 µM to the maturation medium of bovine cumulus–oocyte complexes (COC) significantly increased estradiol production, although no effect was found on progesterone (Maya-Soriano et al., Reference Maya-Soriano, Taberner and López-Béjar2013). Vanholder et al. (Reference Vanholder, Leroy, Van Soom, Opsomer, Maes, Coryn and de Kruif2005) also reported that a 2.5-fold higher free OlAc (500 μM), such as stearic acid and palmitic acid, stimulated estradiol secretion of bovine granulosa cells, while simultaneously inhibiting cell proliferation. A relatively lower concentration of OlAc (300 μM) also inhibited human granulosa cell proliferation (Mu et al., Reference Mu, Yanase, Nishi, Tanaka, Saito, Jin, Mukasa, Okabe, Nomura, Goto and Nawata2001). It is of note that all of these OlAc concentrations are 3-fold to 7.8-fold higher than the follicular fluid value of ~64 μM (Jungheim et al., Reference Jungheim, MacOnes, Odem, Patterson, Lanzendorf, Ratts and Moley2011) and are also in 1.5-fold to 2.3-fold excess of the free OlAc in plasma. In a later study, these fatty acids individually did not reproduce such effects on bovine theca cells (Vanholder et al., Reference Vanholder, Lmr Leroy, Van Soom, Maes, Coryn, Fiers, de Kruif and Opsomer2006). Such cell type-specific differences may be attributed to an in vivo adaptation process that modulates cellular sensitivity to FFAs. This hypothesis was confirmed by a more recent study, which reported decreases in estradiol and progesterone along with the gonadotropin hormone receptors following long-term (8 day) OlAc incubation in a serum-free, immature bovine granulosa cell culture model (Yenuganti et al., Reference Yenuganti, Viergutz and Vanselow2016). It has been suggested that OlAc-induced changes are due to a reduced response to gonadotropic hormones (Yenuganti et al., Reference Yenuganti, Viergutz and Vanselow2016). However, because OlAc increased estradiol secretion in short-term cultures of mature bovine granulosa cells (Vanholder et al., Reference Vanholder, Lmr Leroy, Van Soom, Maes, Coryn, Fiers, de Kruif and Opsomer2006), it rather appears to be due to cell-specific adaptive responses. Consistently, theca cells are more directly supplied with blood, which is rich in FFAs (Vanholder et al., Reference Vanholder, Lmr Leroy, Van Soom, Maes, Coryn, Fiers, de Kruif and Opsomer2006).
Altogether, these data underscore the dynamic changes occurring in the OlAc content of follicles during the reproductive cycle, which can modulate steroidogenesis.
Oleic acid in formation of competent oocytes
A unique diversity of lipid molecular compounds has been documented for oocytes. For instance, a more complex lipid composition was recorded during the in vitro maturation process of porcine oocytes (Pirro et al., Reference Pirro, Oliveri, Ferreira, González-Serrano, Machaty and Cooks2014). The knowledge accumulated regarding the effect of OlAc on follicular development and measures of oocyte developmental competence, including maturation and fertilization rate, is discussed further below. Table 1 summarizes data related to cattle, as the most studied species.
Table 1 Effect of oleic acid supplementation on cumulus-granulosa cell function, oocyte characteristics and embryo development in cattle
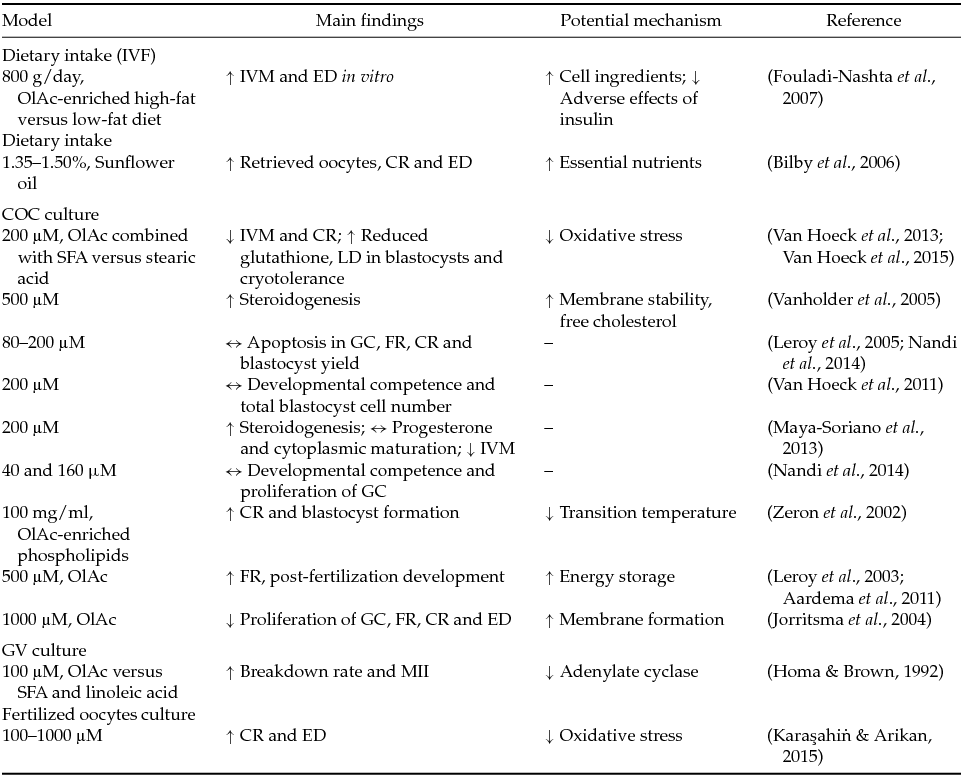
*COC, cumulus–oocyte complex; CR, cleavage rate; d, days; ED, embryo development; FR, fertilization rate; GC, cumulus–granulosa cells; GV, germinal vesicles; IVM, in vitro maturation rate; LD, lipid droplets; MII, metaphase II oocytes; OlAc, oleic acid; SFA, saturated fatty acids. ↓, significant decrease; ↑, significant increase; ↔ no significant change.
Follicular fluid
The free fatty acid fraction in bovine (Leroy et al., Reference Leroy, Vanholder, Mateusen, Christophe, Opsomer, de Kruif, Genicot and Van Soom2005) and human (Valckx et al., Reference Valckx, Arias-Alvarez, De Pauw, Fievez, Vlaeminck, Fransen, Bols and Leroy2014a) sera and ovarian follicular fluid is rich in palmitic acid, stearic acid and OlAc. The concentration of individual FFA in human serum was reflected in follicular fluid, but overall the relationship was weak (Jungheim et al., Reference Jungheim, MacOnes, Odem, Patterson, Lanzendorf, Ratts and Moley2011). Notably, human follicular fluid showed an increase of 3.3% in stearic acid and a reduction of −7.0% in OlAc relative to serum (Jungheim et al., Reference Jungheim, MacOnes, Odem, Patterson, Lanzendorf, Ratts and Moley2011). These data suggest that a selective transport mode determines OlAc concentration in follicular fluid. This selective transport may be accompanied by other metabolites, as evidenced in the placental transport of OlAc coupled with amino acids in primary human trophoblasts (Lager et al., Reference Lager, Gaccioli, Ramirez, Jones, Jansson and Powell2013). Hudson et al. (Reference Hudson, Berg, Green, Back, Thorstensen, Peterson, Pitman and McNatty2014) have shown that in a low amino acid environment the physiological concentration of OlAc, but not palmitic acid or stearic acid, caused a reduction in fluorescence dye transfer from bovine cumulus cells to the oocyte. The expression of connexin proteins, as the main structural components of gap junctions, has been considered to be modulated by nutrients (Hudson et al., Reference Hudson, Berg, Green, Back, Thorstensen, Peterson, Pitman and McNatty2014). These data support the hypothesis that OlAc in the follicle microenvironment can contribute to the regulation of metabolite transport through the control of gap junctional communication between cumulus cells and the developing oocyte.
Fatty acid composition and glucose content in follicular fluid appear to be generally involved in follicular development (Warzych et al., Reference Warzych, Cieslak, Madeja, Pawlak, Wolc and Lechniak2014). Notably, OlAc in follicular fluid was related to a higher bovine oocyte quality, in terms of COC morphology and follicular diameter (Warzych et al., Reference Warzych, Cieslak, Madeja, Pawlak, Wolc and Lechniak2014). However, the fatty acid composition of follicular fluid was not associated with bovine (Sinclair et al., Reference Sinclair, Lunn, Kwong, Wonnacott, Linforth and Craigon2008) or human (O'Gorman et al., Reference O'Gorman, Wallace, Cottell, Gibney, McAuliffe, Wingfield and Brennan2013) oocyte cleavage following in vitro fertilization (IVF). In another study, the concentration of palmitic acid and stearic acid in human follicular fluid was associated with morphologically poor and good COCs, respectively (Sinclair et al., Reference Sinclair, Lunn, Kwong, Wonnacott, Linforth and Craigon2008). In a clinical study, we have reported that the number of mature oocytes from women undergoing IVF cycle was inversely associated with total saturated fatty acid content of phospholipids in follicular fluid (Shaaker et al., Reference Shaaker, Rahimipour, Nouri, Khanaki, Darabi, Farzadi, Shahnazi and Mehdizadeh2012). Associations between phospholipid OlAc and the number of retrieved mature oocytes showed a positive trend (Shaaker et al., Reference Shaaker, Rahimipour, Nouri, Khanaki, Darabi, Farzadi, Shahnazi and Mehdizadeh2012). Moreover, a higher concentration of total saturated and a lower concentration of total unsaturated fatty acids were found in patients with cleavage failure (O'Gorman et al., Reference O'Gorman, Wallace, Cottell, Gibney, McAuliffe, Wingfield and Brennan2013). Intrafollicular OlAc concentration is significantly increased in phospholipid fraction while showing a reduction in the non-esterified fatty acids fraction as bovine follicles become dominant (Renaville et al., Reference Renaville, Bacciu, Comin, Motta, Poli, Vanini and Prandi2010). Follicular fluid of women with higher body mass index and lower IVF outcome showed increased phospholipid content (Fayezi et al., Reference Fayezi, Darabi, Nouri, Rahimipour and Mehdizadeh2014) but reduced concentration of OlAc in the phospholipid fraction (Valckx et al., Reference Valckx, Arias-Alvarez, De Pauw, Fievez, Vlaeminck, Fransen, Bols and Leroy2014a). These observations argued for a link between relative ratio of OlAc/other fatty acids in follicular fluid phospholipids and oocyte development.
Follicular growth
A recent study using high-resolution mass spectrometry technique demonstrated that the fatty acid patterns of porcine follicles were largely different from non-follicular ovarian tissue (Uzbekova et al., Reference Uzbekova, Elis, Teixeira-Gomes, Desmarchais, Maillard and Labas2015). Moreover, porcine follicles were heterogeneous in their lipid composition, possibly reflecting changes in fatty acids over the natural course of ovarian folliculogenesis (Uzbekova et al., Reference Uzbekova, Elis, Teixeira-Gomes, Desmarchais, Maillard and Labas2015). More recently, Warzych et al. (Reference Warzych, Pawlak, Pszczola, Cieslak and Lechniak2017) have shown that sexual maturity in bovine alters the follicular environment with regard to the lipid droplet content within the oocyte and follicular fluid fatty acid composition. Overall, these data further support the idea that dynamic change in fatty acid content of follicular compartments contributes to regulating female gametogenesis. Several studies, which are discussed below, point to the specific effect of OlAc on the process of follicular growth.
Diet supplementation with long-chain fatty acids has been shown to increase the ovulation rate, the number of follicles and serum progesterone in ewes (El-Shahat & Abo-El Maaty, Reference El-Shahat and Abo-El Maaty2010). However, depending on the type of fatty acid used in the diet, the effect on oocyte development can be very different (Leroy et al., Reference Leroy, Sturmey, Van Hoeck, De Bie, McKeegan and Bols2014). Consistent with this hypothesis, more oocytes were collected from dairy cows fed OlAc as compared with cows fed trans OlAc, LnAc or linolenic acid (Bilby et al., Reference Bilby, Block, do Amaral, Sa Filho, Silvestre, Hansen, Staples and Thatcher2006).
Follicular growth and antrum formation in bovine are affected by elevated FFAs concentrations in vitro (Van Hoeck et al., Reference Van Hoeck, Bols, Binelli and Leroy2014; Valckx et al., Reference Valckx, De Bie, Michiels, Goovaerts, Punjabi, Ramos-Ibeas, Gutierrez-Adan, Bols and Leroy2015). Moreover, experimental data support the idea that FFAs not only modulate final oocyte maturation, but also potentially interfere with follicular growth at the early stages of development (Leroy et al., Reference Leroy, Valckx, Jordaens, De Bie, Desmet, Van Hoeck, Britt, Marei and Bols2015). The same manner as in vivo, however, depending on the type and ratio of fatty acids the final effect may be different (Leroy et al., Reference Leroy, Valckx, Jordaens, De Bie, Desmet, Van Hoeck, Britt, Marei and Bols2015). The diameter of in vitro-cultured murine follicles was higher and apoptosis markers in luteinized granulosa cells were reduced with OlAc treatment (210 μM), compared with high stearic acid (Valckx et al., Reference Valckx, Van Hoeck, Arias-Alvarez, Maillo, Lopez-Cardona, Gutierrez-Adan, Berth, Cortvrindt, Bols and Leroy2014b). In addition, when OlAc was used in combination with stearic acid and palmitic acid these differences were eliminated (Valckx et al., Reference Valckx, Van Hoeck, Arias-Alvarez, Maillo, Lopez-Cardona, Gutierrez-Adan, Berth, Cortvrindt, Bols and Leroy2014b). Furthermore, in a rat model, the FSH/FSH receptor pathway in granulosa cells that triggers ovarian follicular growth from primordial stage involves the activation of de novo OlAc synthesis as a downstream consequence (Moreau et al., Reference Moreau, Froment, Tosca, Moreau and Dupont2006). Finally, a more complete understanding of the role of OlAc in follicular growth will require developing new strategies that focus on earlier stages of gametogenesis, including primordial germ cell specification and proliferation.
Oocyte quality and fertilization
Post-fertilization events such as the formation of zygote and embryogenesis are considered as markers for developmental capacity of oocytes. It has long been suggested that the polyunsaturated fatty acid (PUFA) supplements can beneficially affect fertility (Wathes et al., Reference Wathes, Abayasekara and Aitken2007). Although PUFA-enriched diet increased dominant follicle size in dairy cows and OlAc-enriched diet increased recovery rate of oocytes, no significant additional beneficial effects were seen between OlAc- and PUFA-enriched diets with respect to oocyte quality as characterized by subsequent embryo development (Bilby et al., Reference Bilby, Block, do Amaral, Sa Filho, Silvestre, Hansen, Staples and Thatcher2006). Compared with a low-fat diet, a high-fat diet containing mainly palmitic acid and OlAc resulted in significantly improved developmental potential of oocytes in lactating dairy cows, as evidenced by increased rate of blastocyst production and quality during IVF, possibly through enhanced biosynthesis of cell ingredients and neutralizing the adverse effects of insulin (Fouladi-Nashta et al., Reference Fouladi-Nashta, Gutierrez, Gong, Garnsworthy and Webb2007).
Although a 100 μM dose of albumin-bound OlAc did not show a statistically significant difference compared with the control albumin alone, the breakdown rate of bovine germinal vesicles and oocyte progression to MII were higher after incubation with OlAc as compared with palmitoleic acid, stearic acid and LnAc, possibly due to a specific activation of adenylate cyclase (Homa & Brown, Reference Homa and Brown1992). Moreover, LnAc significantly inhibited in vitro bovine cumulus cell expansion and decreased the percentage of oocytes at MII stage in a dose-dependent manner (Marei et al., Reference Marei, Wathes and Fouladi-Nashta2010). Although maturation rate was not affected, incubation with 500 µM albumin-bound OlAc during in vitro maturation significantly increased the percentage of bovine oocytes that were fertilized (Aardema et al., Reference Aardema, Vos, Lolicato, Roelen, Knijn, Vaandrager, Helms and Gadella2011). However, adding both saturated fatty acids palmitic acid and stearic acid reversed these effects. Furthermore, OlAc reversed the detrimental effects of palmitic acid and stearic acid (Aardema et al., Reference Aardema, Vos, Lolicato, Roelen, Knijn, Vaandrager, Helms and Gadella2011). Free stearic acid was much more potent than the combined OlAc, palmitic acid and stearic acid to increase the expression of genes related to apoptosis and oxidative stress, including Bcl-2-associated X protein (BAX), glutathione peroxidase 1 (GPX1) and superoxide dismutase 1 (SOD1), in bovine oocytes and luteinized granulosa cell cultures (Van Hoeck et al., Reference Van Hoeck, Leroy, Alvarez, Rizos, Gutierrez-Adan, Schnorbusch, Bols, Leese and Sturmey2013; Valckx et al., Reference Valckx, Van Hoeck, Arias-Alvarez, Maillo, Lopez-Cardona, Gutierrez-Adan, Berth, Cortvrindt, Bols and Leroy2014b). However, the expression level of the proliferation-related gene growth arrest and DNA-damage-inducible (GADD45B) was increased after treatment with 210 μM OlAc (Van Hoeck et al., Reference Van Hoeck, Rizos, Gutierrez-Adan, Pintelon, Jorssen, Dufort, Sirard, Verlaet, Hermans, Bols and Leroy2015). In addition, the glutathione content of bovine oocytes and the number of lipid droplets in the resulting morulae were lower following high stearic acid treatment compared with that of combined saturated fatty acids and OlAc (Van Hoeck et al., Reference Van Hoeck, Rizos, Gutierrez-Adan, Pintelon, Jorssen, Dufort, Sirard, Verlaet, Hermans, Bols and Leroy2015). These observations were attributed to the compensating action of OlAc.
A few studies have shown no significant effect at lower doses of free OlAc alone compared with vehicle control. OlAc at concentrations equivalent to that of FFAs in postpartum cows follicular fluid (200 μM) showed no effect (Leroy et al., Reference Leroy, Vanholder, Mateusen, Christophe, Opsomer, de Kruif, Genicot and Van Soom2005) on in vitro maturation, fertilization and cleavage rate and blastocyst yield. Despite significant increasing effect on cumulus cell steroidogenesis, exposure to a 200 µM concentration of OlAc resulted in no beneficial effects on cytoplasmic maturation, and nuclear maturation of heifer oocytes (Maya-Soriano et al., Reference Maya-Soriano, Taberner and López-Béjar2013). In contrast to palmitic and stearic acid, OlAc at concentrations between 40 and 160 μM showed no detrimental effect on bovine oocyte maturation rate and subsequent fertilization rate and embryo yield as well as on granulosa cell growth (Nandi et al., Reference Nandi, Tripathi, Farman, Gupta and Mondal2014). These data further highlight the importance of the ultimate OlAc ratio of the cellular lipids, over the entire quantity, which needs to be taken into account in future studies.
Concentration and form of OlAc supplementation in the growth medium may also determine the effect on the developmental capacity of oocytes. Albumin-bound OlAc at a high concentration equivalent to plasma FFAs in postpartum cows (1 mM) reduced the proliferation of granulosa cells, in vitro fertilization and subsequent cleavage and embryo development (Jorritsma et al., Reference Jorritsma, César, Hermans, Kruitwagen, Vos and Kruip2004). However, the study did not distinguish between supplementation alone with albumin and the control condition. Thus, besides the high concentration, these adverse effects can be caused by addition of albumin alone (Leroy et al., Reference Leroy, Vanholder, Mateusen, Christophe, Opsomer, de Kruif, Genicot and Van Soom2005, Reference Leroy, Van Soom, Opsomer and Bols2008). Notably, incomplete extraction of fatty acids from albumin has been postulated as a possible explanation for variations in embryo formation rate with different concentrations of albumin (Cagnone & Sirard, Reference Cagnone and Sirard2014). In contrast, a previous study showed that a high dose of albumin-bound OlAc (1.25 mM) was the most effective treatment among other unsaturated fatty acids for promoting rat embryos development to blastocyst stage (Khandoker & Tsujii, Reference Khandoker and Tsujii1999). Other studies have shown that a lower amount of OlAc (500 µM) exerts a promoting effect on oocyte developmental competence post-fertilization (Leroy et al., Reference Leroy, Vanholder, Van Soom, Opsomer, Bols and de Kruif2003; Aardema et al., Reference Aardema, Vos, Lolicato, Roelen, Knijn, Vaandrager, Helms and Gadella2011), possibly by increasing lipid storage in bovine oocyte (Aardema et al., Reference Aardema, Vos, Lolicato, Roelen, Knijn, Vaandrager, Helms and Gadella2011). In addition, OlAc prevents suppression of post-fertilization development induced by saturated fatty acids in bovine (Aardema et al., Reference Aardema, Vos, Lolicato, Roelen, Knijn, Vaandrager, Helms and Gadella2011; Van Hoeck et al., Reference Van Hoeck, Sturmey, Bermejo-Alvarez, Rizos, Gutierrez-Adan, Leese, Bols and Leroy2011). It has been hypothesized that OlAc may mitigate the negative effects induced by saturated fatty acids. As evidenced in bovine oocytes (Aardema et al., Reference Aardema, Vos, Lolicato, Roelen, Knijn, Vaandrager, Helms and Gadella2011) and in hamster ovary cells (Listenberger et al., Reference Listenberger, Ory and Schaffer2001), co-exposure to OlAc resulted in the metabolic channelling of palmitic and stearic acid into the non-toxic lipid droplets.
Altogether, these results suggest that the ultimate effect of free OlAc on oocyte in vitro maturation (IVM) is determined by the relative ratio of cellular OlAc to other fatty acids. While no consensus has been reached regarding the effect of OlAc alone on IVM, it is evident that OlAc counteracts the detrimental consequences of exposure to saturated fatty acids during IVM.
The efficient metabolic incorporation of OlAc by bovine oocyte has been demonstrated by altered lipid droplets (Aardema et al., Reference Aardema, Vos, Lolicato, Roelen, Knijn, Vaandrager, Helms and Gadella2011), energy metabolism-related gene expression, and mitochondrial function and ultrastructure (Van Hoeck et al., Reference Van Hoeck, Leroy, Alvarez, Rizos, Gutierrez-Adan, Schnorbusch, Bols, Leese and Sturmey2013) following exposure to OlAc during IVM. The physical properties and thermal responses of the plasma membrane remarkably changed during the process of oocyte maturation. A study performed using Fourier transform infrared analysis detected a large difference in membrane lipid phase transition temperature among human germinal vesicles, mature (MII) oocytes and zygotes (Ghetler et al., Reference Ghetler, Yavin, Shalgi and Arav2005). The higher phase transition temperature in zygotes may reflect differences in the fatty acids within the membrane phospholipids. Indeed, a high ratio of OlAc to saturated fatty acids reduced the transition temperatures of lipids and increased membrane fluidity in animal oocytes (Arav et al., Reference Arav, Pearl and Zeron2000; Zeron et al., Reference Zeron, Ocheretny, Kedar, Borochov, Sklan and Arav2001). Electrofusion of bovine COCs with liposomes prepared from egg phosphatidylcholine with a high content of OlAc decreased transition temperature and increased blastocyst formation in vitro (Zeron et al., Reference Zeron, Tomczak, Crowe and Arav2002). Bovine GV-stage oocytes were much more susceptible to cryoinjury than mature oocytes and embryos (Arav et al., Reference Arav, Zeron, Leslie, Behboodi, Anderson and Crowe1996). Interestingly, the fusion of large membrane fragments from mature oocytes with bovine GV oocytes membrane enhanced their chilling resistance (Arav et al., Reference Arav, Zeron, Leslie, Behboodi, Anderson and Crowe1996). Notably, in contrast with palmitic acid and stearic acid, no adverse effect on the embryo cryotolerance was observed with OlAc treatment during in vitro maturation of bovine oocytes (Shehab-El-Deen et al., Reference Shehab-El-Deen, Leroy, Maes and Van Soom2009). These observations were attributed to alteration in the membrane lipid composition. Because of the large capacity of OlAc to solubilize lipids (Robinson & Cistola, Reference Robinson and Cistola2014), such potential changes in OlAc will profoundly affect cell membrane function.
The lower bovine fertility during summer than winter is attributed to disrupted development of oocyte due to heat stress (Rivera & Hansen, Reference Rivera and Hansen2001). Notably, OlAc reaches 38% of total fatty acids and became the most abundant fatty acid in membrane phospholipids of bovine germinal vesicle oocytes during winter, an increase of 28% as compared with the summer season (Zeron et al., Reference Zeron, Ocheretny, Kedar, Borochov, Sklan and Arav2001). This compositional change generally modifies physical properties of membranes, such as melting temperature (Zeron et al., Reference Zeron, Ocheretny, Kedar, Borochov, Sklan and Arav2001). Such a process may be involved in increased oocyte quality in winter. Whether there is any change in the plasma membrane fatty acids during the oocyte maturation remains to be determined.
As noted earlier, the concentrations of fatty acids, including OlAc, in follicular fluid and oocyte quality potential were intimately correlated in bovine (Warzych et al., Reference Warzych, Pawlak, Pszczola, Cieslak and Lechniak2017). According to these results, during the prepubertal period de novo synthesis of fatty acids, as the main energy resources in ovarian follicles, is low and insufficient to fully support oocyte development (Warzych et al., Reference Warzych, Pawlak, Pszczola, Cieslak and Lechniak2017). A study on human cumulus–granulosa cells has demonstrated that SCD activity is required for cumulus cell lipid storage and steroidogenesis (Fayezi et al., Reference Fayezi, Ghaffari Novin, Darabi, Norouzian, Nouri, Farzadi and Darabi2017). In addition, oocyte maturation was negatively affected by SCD inhibition in cumulus–granulosa cells, possibly due to deficient lipid-mediated paracrine support. Subsequently, these effects were rescued by supplementation of the main product of SCD OlAc, confirming the idea that its de novo production in cumulus cells contributes to the acquisition of oocyte meiotic and developmental competence during folliculogenesis (Fayezi et al., Reference Fayezi, Ghaffari Novin, Darabi, Norouzian, Nouri, Farzadi and Darabi2017). It is interesting to note that de novo synthesis and paracrine release of OlAc by astrocytes induced neuronal differentiation (Tabernero et al., Reference Tabernero, Lavado, Granda, Velasco and Medina2001). This function was mediated by the activation of protein kinase C (Tabernero et al., Reference Tabernero, Lavado, Granda, Velasco and Medina2001), which is known to promote oocyte maturation and early embryo development (Mondadori et al., Reference Mondadori, Neves and Gonçalves2008; Tepekoy et al., Reference Tepekoy, Ustunel and Akkoyunlu2014). In addition, Aardema et al. (Reference Aardema, van Tol, Wubbolts, Brouwers, Gadella and Roelen2017) have shown that treatment of SCD inhibitor plus stearic acid during IVM led to a decrease in the SCD activity index in cumulus cells, as well as a reduction in the number of produced blastocysts. The authors attributed these findings to the protective effect of SCD against saturated fatty acids via conversion to unsaturated fatty acids. Taken together, a sufficient accessibility of metabolic processes to OlAc and its relative amount to the cell fatty acid content are particularly important in oocyte maturation.
Oleic acid in preimplantation embryo development
Embryo cleavage and blastocyst formation or hatching are the main stages of early embryogenesis. The ratio of various fatty acids is an important metabolic feature and may strongly influence reproductive outcomes. The relative percentage of OlAc markedly increased during the developmental stages of bovine (Menezo et al., Reference Menezo, Renard, Delobel and Pageaux1982) and rabbit (Yahia Khandoker et al., Reference Yahia Khandoker, Tsujii and Karasawa1998) embryos. Consistent with these data, OlAc and LnAc were more abundant in the late preimplantation stage human embryos (Haggarty et al., Reference Haggarty, Wood, Ferguson, Hoad, Srikantharajah, Milne, Hamilton and Bhattacharya2006). These observations can reflect a differential pattern of fatty acid uptake during embryo development which may be conserved among species. Indeed, at later developmental stages, a trend towards increasing uptake of OlAc (Wang & Tsujii, Reference Wang and Tsujii1999; Haggarty et al., Reference Haggarty, Wood, Ferguson, Hoad, Srikantharajah, Milne, Hamilton and Bhattacharya2006) and LnAc (Haggarty et al., Reference Haggarty, Wood, Ferguson, Hoad, Srikantharajah, Milne, Hamilton and Bhattacharya2006) over palmitic acid was seen in human and mouse embryos. In addition, during preimplantation embryo development the channelling of exogenous OlAc was preferentially directed towards phospholipids and away from intermediate energy substrates such as triglycerides (Wang & Tsujii, Reference Wang and Tsujii1999). According to these data, active uptake and metabolic channelling of OlAc towards phospholipids can contribute to early embryo development.
The data obtained from phosphosphingolipidome analysis showed increased concentration of phosphatidylcholine classes containing OlAc (3.8-fold), LnAc (4.5-fold) and linolenic acid (3-fold) for in vivo produced compared with in vitro produced bovine embryos (Sudano et al., Reference Sudano, Santos, Tata, Ferreira, Paschoal, Machado, Buratini, Eberlin and Landim-Alvarenga2012). Moreover, phosphatidylethanolamines, ceramides (Ferreira et al., Reference Ferreira, de Oliveira, Gonçalves and Catharino2014) and cholesteryl ester (González-Serrano et al., Reference González-Serrano, Pirro, Ferreira, Oliveri, Eberlin, Heinzmann, Lucas-Hahn, Niemann and Cooks2013) containing primarily OlAc have been identified as blastomeric biomarkers for bovine embryo quality using ionization mass spectrometry approach. Indeed, in vivo produced bovine blastocysts showed higher concentrations of OlAc in phospholipids and cholesteryl-ester fractions compared with their in vitro derived counterparts (González-Serrano et al., Reference González-Serrano, Pirro, Ferreira, Oliveri, Eberlin, Heinzmann, Lucas-Hahn, Niemann and Cooks2013). Accordingly, unsaturated fatty acid content may be an important factor in the post-cryopreservation survival of in vivo (Sudano et al., Reference Sudano, Santos, Tata, Ferreira, Paschoal, Machado, Buratini, Eberlin and Landim-Alvarenga2012) and in vitro (Pereira et al., Reference Pereira, Baptista, Vasques, Horta, Portugal, Bessa, Silva, Pereira and Marques2007) produced bovine embryos. Moreover, a diet fed to cows enriched in LnAc compromised the embryo cryotolerance and decreased the hatching rate of in vivo produced embryos (Guardieiro et al., Reference Guardieiro, Machado, Bastos, Mourão, Carrijo, Dode, Leroy and Sartori2014). Therefore, it appears that among unsaturated fatty acids, a beneficial effect is produced by OlAc on embryo cryotolerance.
Hatching rate of bovine blastocyst in a culture supplemented with 0.1% serum lipid fraction tended to be lower compared with total serum-containing medium. This lipid fraction was extracted using ether, a nonpolar solvent capable of extracting major lipid classes. Compositional analysis showed that this fraction had a fatty acid concentration comparable with total serum. However, it contained significantly reduced concentrations of OlAc and increased amounts of palmitic acid and stearic acid compared with total serum (Cagnone & Sirard, Reference Cagnone and Sirard2014). Nevertheless, a large-scale gene expression analysis of bovine blastocysts failed to detect differences in transcriptome following these in vitro culture conditions (Cagnone & Sirard, Reference Cagnone and Sirard2014). These results suggest that posttranscriptional modifications may be responsible for the changes in the embryo development upon serum lipid, particularly OlAc, supplementation.
Based on previous evidence for the association between unsaturated fatty acid content and cryopreservation success of embryos (Shehab-El-Deen et al., Reference Shehab-El-Deen, Leroy, Maes and Van Soom2009; Sudano et al., Reference Sudano, Santos, Tata, Ferreira, Paschoal, Machado, Buratini, Eberlin and Landim-Alvarenga2012), an in vitro study demonstrated that supplementation of bovine embryo culture medium with OlAc at a concentration of 1.0 mM increased the rates of embryonic cleavage, development and quality (Karaşahiṅ & Arikan, Reference Karaşahiṅ and Arikan2015).
Stinshoff et al. (Reference Stinshoff, Wilkening, Hanstedt, Bollwein and Wrenzycki2014) have observed that conjugated LnAc significantly suppressed the expression of SCD, a key enzyme for de novo OlAc synthesis, and resulted in reduced bovine embryo development in vitro, a phenotype very similar to when mouse embryos (Ben-David et al., Reference Ben-David, Gan, Golan-Lev, Arora, Yanuka, Oren, Leikin-Frenkel, Graf, Garippa, Boehringer, Gromo and Benvenisty2013) were exposed to selective inhibitors of SCD. The requirement for LnAc, as another abundant unsaturated fatty acid, in embryo development is controversial. Only limited positive effects on in vitro embryonic development and quality were observed in bovine by supplementation of LnAc (Karaşahiṅ & Arikan, Reference Karaşahiṅ and Arikan2015). Remarkably, treatment of mouse pronuclear and 2-cell stages with albumin-bound PUFAs including LnAc resulted in a significantly lower percentage of cleaved embryos and blastocysts (Nonogaki et al., Reference Nonogaki, Noda, Goto, Kishi and Mori1994). Consistent with these results, the treatment of bovine COCs with LnAc, even at a concentration not affecting oocyte maturation (50 mM), significantly decreased subsequent cleavage and blastocyst rates (Marei et al., Reference Marei, Wathes and Fouladi-Nashta2010). These adverse effects were accompanied by a sharp increase in the arachidonic acid-dependent pro-inflammatory pathway (Marei et al., Reference Marei, Wathes and Fouladi-Nashta2010). In agreement to these findings, a reduced arachidonic acid content in bovine oocytes was associated with the improved quality of derived embryo without an increase in oocyte maturation or embryo production rates (Lapa et al., Reference Lapa, Marques, Alves, Vasques, Baptista, Carvalhais, Silva Pereira, Horta, Bessa and Pereira2011).
Overall, the current data from in vitro models suggested that OlAc supplementation may beneficially effect embryo development that is obviously distinct from other bioactive fatty acids like LnAc and arachidonic acid.
Potential mechanism of reproductive function of oleic acid in female
A general weakness in the most studies reviewed lay in their observational nature, which did not allow mechanistic insights into the reproductive role of fatty acids, in particular OlAc. Nevertheless, current knowledge suggests that potential mechanisms underlying association of OlAc with female reproduction may include altered metabolic channelling, plasma membrane fluidity, oxidative stress and intracellular signalling events (Fig. 4).

Figure 4 Potential mechanisms underlying the effects of oleic acid on ovarian function and embryo development. The additive effects on embryo development are shown in dot-dash lines. Oleic acid profoundly increases partitioning of fatty acids towards lipid droplet which in turn serves as energy supply and protects oocytes and granulosa cells against lipotoxicity, and enhances lipid-mediated paracrine support of oocytes (I). Oocyte plasma membrane fluidity and cell divisions at the early stages of embryo development can be modified by oleic acid-promoted phospholipid synthesis (II). Oleic acid can influence follicular metabolic activities, steroidogenesis and oocyte development either directly by acting as a regulator of cellular signalling via the modulation of oxidative stress (III) and the activation of protein kinase C (PKC) (IV).
The division from one to four blastomeres requires a large increase in membrane surface area, as much as 1.74-fold (Pratt & George, Reference Pratt and George1989). Oleic acid is a major fatty acid in membrane phospholipids (Homa et al., Reference Homa, Racowsky and McGaughey1986; Kim et al., Reference Kim, Kinoshita, Ohnishi and Fukui2001; Zeron et al., Reference Zeron, Ocheretny, Kedar, Borochov, Sklan and Arav2001) and is essential for organization and normal function of membrane (for review see Lopez et al., Reference Lopez, Bermudez, Montserrat-De La Paz, Jaramillo, Varela, Ortega-Gomez, Abia and Muriana2014). The functional significance of OlAc in membrane formation at the earliest stages of zygote development remains to be investigated.
Oleic acid activates proliferative and maturational signalling pathways in which protein kinase C participates (Tabernero et al., Reference Tabernero, Lavado, Granda, Velasco and Medina2001). In light of the information available on the critical role of protein kinase C in bovine oocyte maturation and early embryo development (Mondadori et al., Reference Mondadori, Neves and Gonçalves2008), OlAc may be considered as an important regulator of reproduction acting at the level of a common signalling pathway. This aspect of reproduction control by OlAc will require further study, which will help to deal with conflicting viewpoints.
Advanced lipidomics analyses point to prostaglandins and cannabinoids as markers for female fertility (Vilella et al., Reference Vilella, Ramirez and Simón2013a; Agirregoitia et al., Reference Agirregoitia, Ibarra-Lecue, Totorikaguena, Mendoza, Expósito, Matorras, Urigüen and Agirregoitia2015). Metabolism of OlAc and omega-6 fatty acids – also known as n-6 PUFAs – shares common enzymatic pathway steps catalyzed by desaturases and prostaglandin-endoperoxide synthases (Cheng et al., Reference Cheng, Abayasekara, Elmes, Kirkup and Wathes2015). Therefore, OlAc can modify PUFA metabolism and, subsequently, prostaglandin and cannabinoids synthetic pathways. Indeed, OlAc significantly increased the ratio of prostaglandin E2 to prostaglandin F2α produced by endometrial cells isolated from the late pregnant ewes (Cheng et al., Reference Cheng, Abayasekara, Elmes, Kirkup and Wathes2015). Such change in prostaglandin ratio has been associated with embryo implantation in human (Vilella et al., Reference Vilella, Ramirez, Berlanga, Martínez, Alamá, Meseguer, Pellicer and Simón2013b) and fetal maturation in porcine (Cao et al., Reference Cao, Yosida, Kitazawa and Taneike2005; Ziecik et al., Reference Ziecik, Waclawik and Bogacki2008), which may similarly contribute to ovarian folliculogenesis or granulosa cell differentiation.
Conceiving and sustaining a pregnancy to term are the most reliable markers for oocyte quality and embryo competence. This aspect has been particularly examined in the study of dietary PUFAs. Indeed, feeding PUFAs dynamically during the postpartum period increased the occurrence of pregnancy in cattle (Silvestre et al., Reference Silvestre, Carvalho, Crawford, Santos, Staples, Jenkins and Thatcher2011; Dirandeh et al., Reference Dirandeh, Towhidi, Zeinoaldini, Ganjkhanlou, Pirsaraei and Fouladi-Nashta2013). Regarding dietary OlAc, this point has not been studied.
Conclusions
Although available data are not ideally detailed, most studies suggest that dietary or de novo synthesis of OlAc contributes to the acquisition of developmental competence by oocytes and to early stages of embryo development in mammals. The beneficial effects of OlAc may involve one or more of the following possible mechanisms. Firstly, OlAc profoundly increased the partitioning of fatty acids towards lipid droplet which in turn not only protects oocytes and granulosa cells against lipotoxicity but also may promote the lipid-mediated paracrine support of oocytes by surrounding cells. Secondly, OlAc acts as a major determinant of plasma membrane structural organization in follicular cells. Thirdly, OlAc acts directly as a metabolic component or regulator of oxidative stress and cellular signalling. Therefore, this monounsaturated fatty acid may play a significant role in oocyte and early embryo development, suggesting that future studies should explore in more detail its potential effects on physiopathology of female reproduction.
Acknowledgements
This work was supported by the Drug Applied Research Center at Tabriz University of Medical Sciences (grant number #93/710) and Shahid Beheshti University of Medical Sciences (grant number #1393-1-91-13033). Shabnam Fayezi is recipient of a grant from the interdisciplinary PhD programme in the National Elites Foundation (2015–2018).
Authors’ contributions
S.F. wrote the manuscript and made the figures. J.L., M.G.N. and M.D. contributed to the manuscript writing and editing. All authors read and approved the final manuscript.
Declaration of interest
The authors declare that they have no competing interests.