Introduction
The Meuse river enters the Netherlands at Eijsden just south of Maastricht and follows a northerly course through the Limburg province and a small, adjacent part of eastern Belgium and the Dutch province of Brabant, towards the Cuijk and Mook area, 165 km downstream (Fig. 1). This part of the river is called the Meuse valley due to its position in a terraced landscape and is the study area of this paper. The Meuse valley landscape developed under the influence of Pleistocene climate changes, tectonics and human activities. Downstream of it, the river bends westward, and runs through a Holocene delta plain landscape to reach the North Sea in its estuary near Rotterdam.
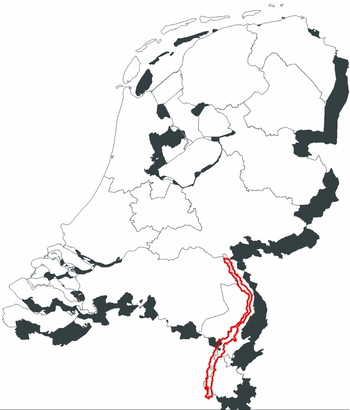
Fig. 1. Research area.
In the study area, the Late Weichselian and Holocene, lowest terrace and floodplain levels are on average 2–5 km wide. This youngest part of the valley geomorphology comprises the Weichselian Lateglacial Interstadial and Younger Dryas river terraces, dissected by Holocene channel belts, and reflects c. 15,000 years of fluvial and aeolian landscape formation, human habitation and land use. In the course of time, humans started to influence and shape the landscape. Regional geomorphological–geological studies of the Meuse and larger tributaries include Van den Broek & Maarleveld (Reference Van den Broek and Maarleveld1963), Kasse et al. (Reference Kasse, Vandenberghe and Bohncke1995, Reference Kasse, Hoek, Bohncke, Konert, Weijers, Cassee and Van der Zee2005), Huisink (Reference Huisink1997) and Tebbens et al. (Reference Tebbens, Veldkamp, Westerhoff and Kroonenberg1999). These studies focused on the Weichselian Pleniglacial to Younger Dryas landscape development, and mainly covered the northern half of the study area (north of the town of Roermond). Comparable studies for the southern part of the Dutch Meuse valley, such as Paulissen (Reference Paulissen1973), are relatively rare, and pay more attention to the Holocene geomorphological elements. In part, the difference in research attention is due to the difference in geomorphology of the two sections: a narrow Holocene floodplain in the north and a broader one in the south where the Meuse valley crosses the Roer Valley Graben.
With the start of the ‘Maaswerken’ project in the mid-1990s and due to the 1992 Valletta (Malta) Convention legislation, large-scale archaeological research along the Meuse increased significantly. At that time, little was known about human habitation, especially in the Holocene bottom of the Meuse valley (see e.g. Verhart, Reference Verhart2000; Rensink, Reference Rensink2017). Since then an increasing amount of data from excavations and other field studies has revealed a wealth of Meuse valley archaeological sites, ranging in time from Early Mesolithic settlements at Well–Aijen to an 18th-century mass horse grave at Borgharen. Large-scale (>100 ha) water-management, sand- and gravel-extraction and nature-development projects and associated (geo)archaeological research have taken place in, for example, Well–Aijen (Tichelman, Reference Tichelmann2005; Ellenkamp & Ruijters, Reference Ellenkamp and Ruijters2012; Ter Wal & Tebbens, Reference Ter Wal and Tebbens2012; Kimenai & Mooren, Reference Kimenai and Mooren2014; Ellenkamp & Ruijters, Reference Ellenkamp and Ruijters2015), Lomm (Gerrets & De Leeuwe, Reference Gerrets and De Leeuwe2011) and Ooijen–Wanssum (Van Kappel et al., Reference Van Kappel, Hanemaaijer, Zuidhoff and Bos2009; Zuidhoff et al., Reference Zuidhoff, Huizer and De Jonge2012; Van Putten, Reference Van Putten2015; Ruijters et al., Reference Ruijters, Van Dijk, Ellenkamp and Tichelmann2016). Upstream of Roermond, key sites include Borgharen (Loonen & Van de Graaf, Reference Loonen and Van de Graaf2013) and Itteren (Meurkens & Tol, Reference Meurkens and Tol2011). Rensink (Reference Rensink2017) provides a detailed description of Meuse valley archaeology.
Unfortunately, the evident richness of archaeological heritage conflicts with the necessity to provide more space and storage capacity for the Meuse river during excessive discharges. To safeguard the downstream regions of the Netherlands from floods, new river channels are dug and levees are lowered. The looming high costs of archaeological research in large plan areas, totalling several thousands of hectares, until the year 2050 required policy and policy-making tools.
The Cultural Heritage Agency of the Netherlands (RCE), Rijkswaterstaat and the Province of Limburg all reacted to the noted conflict of interest and need for policy. As a consequence of the 1992 Valletta Convention, the process of Archaeological Heritage Management was introduced in the Netherlands. Legislation required archaeology to be firmly incorporated in spatial planning. In areas with a high probability of archaeological sites, initiators of ground-disturbing projects need to start the stepwise research process of desktop, auguring and trenching. Ultimately, this inventory process may reveal sites that need protection, ex situ by excavation or, preferably, in situ. In the process, local, provincial and state authorities act as inferential government.
The RCE is committed to optimally ensuring cultural heritage (in a wider sense than archaeology) in spatial planning. The national programme of ‘Identity and Safety’ (Dutch: ‘Eigenheid en Veiligheid’) focuses on cultural heritage in water-related spatial projects. In 2011 the Province of Limburg started to develop archaeological policy for the Meuse valley. The primary policy-making aim was to develop proportional research in which the costs of research were proportional to the yield in scientific knowledge. In 2013, RCE, Rijkswaterstaat and the Province of Limburg decided to combine their efforts and collectively pursue a new predictive map for the Meuse valley in Dutch Limburg.
This article describes the conception and main features of two of these policy-making tools: a geomorphogenetic map and an associated archaeological predictive map series of the Meuse valley. Furthermore, a short introduction to landscape evolution of the Meuse valley and human presence during the last 15,000 years is provided. Our contribution does not, however, aim at a comprehensive detailed reconstruction of the Meuse valley landscape genesis with its fluvial terraces and its (pre- and proto-)historic and modern-day inhabitants.
Landscape evolution and habitation
During the Lateglacial Interstadial, the Meuse river lowered its valley floor and formed a meandering channel belt, narrowing relative to the braid plain of the previous stadial stages (Huisink, Reference Huisink1997). During the Younger Dryas, when winter temperatures in northwest Europe dropped to near-glacial values, and vegetation cover and discharge regimes changed accordingly (e.g. Isarin & Renssen, Reference Isarin and Renssen1999), the Meuse channel belt widened to accommodate increased spring meltwater discharges. Although a (fully) braided style is often assumed to have characterised the river in Younger Dryas times, the morphology of the main channel of that time is not preserved and thus remains unclear. The Meuse main channel may have been a sinuous one, despite the braided style of secondary channels observed in the abandoned river-plain-edge terrace morphology. In that case, a better characterisation of the Younger Dryas situation than ‘braided’ may be a ‘wandering’ system, with channels regularly shifting over the valley floor (Cohen, Reference Cohen2003; Cohen et al., Reference Cohen, Stouthamer, Pierik and Geurts2012). The key to understanding the behaviour is the response of the river to climate and environmental changes, being rapid or more gradual. Under westerly winds extensive dunes were formed on the terraces east of the active river plain (e.g. Isarin et al., Reference Isarin, Renssen and Koster1997).
Traces of human activities during the Younger Dryas stadial may be found on the Interstadial and older terraces close to the active river plain. It may safely be assumed that Late Palaeo-lithic hunters and gatherers will have exploited food and raw material resources in the plain. However, the dynamic character of the shifting Meuse channels will have erased much, though not all, of the in situ evidence during early-spring peak discharges.
The winter and summer temperatures rapidly rose at c. 11.7 ka BP, signifying the Pleistocene–Holocene transition and leading to a change from a multichannel wandering to a single-channel meandering river. The Meuse river incised, this lowering of the bed elevation being due to sediment reworking. Overbank flood deposition of fine-grained, silty sediments took place outside the incising channel belt. The meandering Meuse formed an approximately 1 km wide flood plain, the formation of which gradually slowed down during the relatively stable Middle Holocene. This Mid-Holocene period was preceded by a phase of distinct Early Holocene climate and environmental changes around 6200 BC (8.2 ka event; e.g. Wiersma et al., Reference Wiersma, Roche and Renssen2011; Walker et al., Reference Walker, Berkelhammer, Bjorck, Cwynar, Fisher, Long, Lowe, Newnham, Rasmussen and Weiss2012). The noted deceleration was caused by the increased forest development of the warm and humid Atlantic period, leading to storage of water in the extensive ‘climatic optimum’ deciduous forest.
During the Preboreal, Boreal and Atlantic, the pointbars, levees and residual channels must have provided a relatively risk-free, open and naturally rich and varied environment, as testified by Mesolithic to Middle Bronze Age finds in Well–Aijen (e.g. Bouma & Müller, Reference Bouma and Müller2014; Kimenai & Mooren, Reference Kimenai and Mooren2014). The relatively stable landscape, with abundant forest cover along and at distance from the river (over time increasingly cleared by humans), led to intensive oxidation and leaching of high-lying sandy soils and to consolidation of the low-lying clayey soils. Most probably, the relatively stable situation changed at the end of the Bronze Age and start of the Iron Age, with an increase of river activity. Although archaeological data are limited (the absence of evidence is not evidence of absence), it is assumed that subsequently habitation moved from the pointbars and levees to the higher and safer Younger Dryas terrace. Clearly, more archaeological data are needed to verify the timing of this shift in habitation. The moment of the shift may very well be asynchronous, depending on local geomorphology.
Project approach
The mapping project consisted of two phases. The first phase focused on mapping the geomorphology of the Meuse valley in Dutch Limburg (Isarin et al., Reference Isarin, Ellenkamp, Heunks, De Kramer, Paulussen, Tebbens and Zuidhoff2014). The second phase was the translation of this geomorphogenetic map (Dutch: Geomorfogenetische Kaart van het Maasdal; GKM) to a series of archaeological predictive maps (Dutch: Archeologische Verwachtingskaart van het Maasdal; AVM). To create a new landscape map in the short time available, the mapping started from expert-judgement and lidar imagery interpretation. No formal written source material synthesis was executed at that stage. During workshops and brainstorm sessions the project team, consisting of earth scientists and geoarchaeologists experienced in Meuse valley landscape development, literally plotted their expertise and field knowledge on the highly detailed height maps of the lidar Digital Elevation Model (DEM) (Dutch: Actueel Hoogtebestand Nederland, AHN; Fig. 3). As a starting point for the GKM, the local-scale geomorphological maps and legend of Zuidhoff & Huizer (Reference Zuidhoff and Huizer2015) and Zuidhoff et al. (Reference Zuidhoff, Huizer and De Jonge2012) were used. These studies produced geomorphological maps for 18 ‘plan areas’ in the northern half of the Meuse valley (north of Roermond), supported by 200 lithogenetically interpreted cross-sections. Subsequently, the surrounding areas were mapped by extrapolation from the ‘plan areas’, primarily using the lidar DEM. The legend was optimised by iteration. Then source material comprising reports, articles, theses and (historical) map series was used as verification for the concept landscape model and concept maps. Finally, the maps were digitised for subsequent GIS-based translation to the archaeological predictive map series.

Fig. 2. Classical terrace map by Van den Broek & Maarleveld (Reference Van den Broek and Maarleveld1963).

Fig. 3. Digital Elevation Model of the Ooijen–Wanssum area, northern Limburg.
We conceived the predictive map series of the AVM using a similar strategy to that of its geomorphogenetic predecessor, i.e. by collecting, integrating and synthesising expert judgement gathered from extensive field experience. In practice, the GKM earth-scientific team was expanded with equally experienced archaeologists to form the AVM team. The first challenge was to realise an archaeological predictive model valid for the entire Meuse valley in Dutch Limburg. Predictive models may differ between (geo)archaeologists or research firms. Similar datasets may and do lead to different predictive maps, which in turn may lead to differences in the scope and intensity of research in the case of spatial projects (e.g. Verhagen et al. Reference Verhagen, Deeben, Hallewas, Zoetbrood, Kamermans, Van Leusen, Berger, Bertoncello, Braemer, Davtian and Gazenbeek2005; Cohen et al. Reference Cohen, Arnoldussen, Erkens, Van Popta and Taal2014). The GKM provided a solid and uniform basis for this predictive model, whereas the large and representative AVM team provided a wide basis and collective acceptance.
The expert knowledge involved in constructing the predictive map series is primarily based on site-oriented research. At the same time, these sites were used for testing, simply because they constitute the only available archaeological dataset. We realise that, from the perspective of statistical testing of archaeological concepts, both the landscape model and the archaeological predictive model should be separated from the data necessary to test these models. On the other hand, this separation may not be ideal for creating a map that maximally honours all existing earth-scientific landscape data. Logically, data and model are closely related and therefore interacting and interdependent. In other words, the models may not be truly conceptual, based on data that are also present in the personal knowledge and experience of the GKM and AVM teams. Thus, specific data, both raw and interpreted, transformed into information and knowledge, were used for the models and for testing.
Constructing the GKM
The northern section of the GKM is to a large extent based on a relatively large dataset consisting of c. 4500 hand-augered borehole descriptions and 200 lithogenetical cross-sections, oriented to the Meuse valley axis (‘VerkenningPLUS’: in English, inventory). In 2012, ADC ArcheoProjecten, a Dutch research firm, started a geoarchaeological coring campaign in 18 research areas located between Mook and Roermond, covering a total of c. 2750 ha (Zuidhoff et al., Reference Zuidhoff, Huizer and De Jonge2012; Zuidhoff & Huizer, Reference Zuidhoff and Huizer2015). The focus was on lithogenesis instead of the traditional lithology. In addition, 2 × 2 m test pits were used for detailed profile analysis. The primary aim was to map and reconstruct the litho- and morphogenesis of the main potential water-management project areas (see Isarin et al., Reference Isarin, Ellenkamp, Heunks, De Kramer, Paulussen, Tebbens and Zuidhoff2014). In the course of the process, the landscape proved more varied and complex than assumed. Consequently, other litho- and geomorphogenetic units and profile types were incorporated in the landscape model and its legend. In the drawing room, we mapped the lithogenetic units on detailed lidar DEM (Fig. 3) plots using criteria such as channel pattern, relative and absolute heights of the surface and channel deposits (gradient lines), texture and the ‘freshness’ of the landscape, i.e. the intactness and visibility of the morphology at the present-day surface. Furthermore, we used reference images from comparable present-day rivers, such as the Allier (France) and Wolga (Russian Federation), as well as from recent fluvial sedimentology process research (e.g. Lageweg et al., Reference Lageweg, Van Dijk and Kleinhans2013). Subsequently, hand-drawn boundaries were digitised. The team collectively inspected the digital maps and corrected errors. The conception of the subsequent AVM offered a second round of verification of the GKM, by confrontation with archaeological data, knowledge and expertise.
The GKM shows four dimensions, with x, y (map) as well as z and time (legend). Incorporation of the factor time was essential, in anticipation of the second phase of the project, the translation of the GKM into archaeological predictive maps for four designated archaeological periods. We distinguished five map levels: (1) terraces, (2) fluvial units, (3) age, (4) relatively high (and old) levee and floodplain deposits and (5) relatively low (and young) levee and floodplain deposits (Fig. 6, further below). Added to these five units are specific easily visible elements in the landscape, such as embankments, extraction pits and canals. However, no detailed analysis of surface disturbances was executed.
In general, three Meuse terraces may be distinguished (within the set study area): the Holocene floodplain (H), the Younger Dryas Terrace (D) and the Lateglacial Interstadial Terrace (I). It must be noted that the Lateglacial Interstadial Terrace may include at least two phases of formation (classically placed in the two relatively warm periods of the Bølling and Allerød) and that equally within the Younger Dryas and Holocene floodplain one can see subdivisions, at least locally. Outside the study area, the terrace staircase continues to higher, older levels such as from the Last Glacial (Late Pleniglacial) and older. Small patches of Late Pleniglacial terrace age cannot be fully excluded to have locally preserved within the study area as well (within the Interstadial terrace complex, below Younger Dryas aeolian cover).
In terms of fluvial units, we distinguished floodplains (K after the term ‘Kom’ in Dutch), levees (O after the Dutch ‘Oeverwal’), residual channel (G, referring to ‘restGeul’), pointbar (W, ‘kronkelWaard’), brook valley (B, ‘Beek’), tributary (R, ‘zijRivier’) and alluvial fan (U, after the Dutch term ‘dalUitspoelingswaaier’). Furthermore, a subdivision was made within the terraces based on elevation: a relatively low (L) or relatively high topographical position (H), and unknown (T). At several locations Zuidhoff & Huizer (Reference Zuidhoff and Huizer2015) observed that the Younger Dryas Terrace has at least two development phases, represented by a difference in height (approximately 0.5–1.5 m). The exact genesis and placement in time of this phenomenon are not yet clear. It may be envisaged that the centre of river activity shifted during the course of the Younger Dryas (possibly because of extensive dune formation or choking by coversands, or tectonics) and down-cutting took place, followed by renewed sedimentation by the (semi-)braiding Meuse river (see also Kasse, Reference Kasse1995; Cohen et al., Reference Cohen, Stouthamer, Pierik and Geurts2012).
Although the existence of an Early Holocene phase of pointbar formation, associated with significant incision during the Pleistocene–Holocene transition, had been recognised before, the true significance of these ‘old’ pointbars – also in archaeological terms – became clear only during the Well–Aijen project (Kimenai & Mooren, Reference Kimenai and Mooren2014). It turned out that on these pointbars intensive and continuous human activities took place from the Early Mesolithic until the Middle Bronze Age. These data suggest relatively limited river activity during the Early and Middle Holocene. Recent studies at Wanssum suggest that, at least locally, point-bar formation restarted in the course of the Subboreal (Ruijters et al., Reference Ruijters, Van Dijk, Ellenkamp and Tichelmann2016). A third phase of intensified (most probably partly man-induced) river activity and pointbar formation is attributed to the Roman period (Subatlantic). The recognition of phases of point bar formation (either generic or local) required a chronological subdivision of pointbars (W) and related channels (G) on the GKM:
-
1. Early Holocene and Middle Holocene (Preboreal–Atlantic, GKM code 1);
-
2. Mid-Holocene (Subboreal, GKM code 2);
-
3. Late Holocene (Subatlantic, GKM code 3) not specified;
-
4. Late Holocene Roman Times–Early Middle Ages (GKM code 4);
-
5. Late Holocene Late Middle Ages–Modern Times (GKM code 5);
-
6. Late Holocene nineteenth century (GKM code 6).
It may be clear that due to a lack of areal coverage (and national scale overview; see Hoek et al., Reference Hoek, Lammertsma, Bohncke, Bunnik, Kasse, Schokker and Westerhoff2017) of absolute (14C, OSL) and relative (pollen, archaeology) dates, and the sheer size of the area under study, it is impossible to attribute accurate absolute ages to the units and features of the GKM. The geomorphogenetic units were mapped, after which an approximate age was attached. Actual direct dates of the units are available from a few geoarcheologically investigated sites and sites of previous research only. As a result, slight diachronicity may be present, for example between geomorphological units on the one hand and periods 1, 2 and 3 on the other. In addition, the phases in the landscape development, being generic or local, synchronous or not, have only recently been recognised. The effects on the behaviour of the Meuse of tributaries and streams, such as the Geleenbeek in Sittard (Ruijters et al., Reference Ruijters, Ellenkamp and Tichelman2015), the Geul (De Moor et al., Reference De Moor, Kasse, Van Balen, Vandenberghe and Wallinga2008) and Roer rivers (Van Balen et al., Reference Van Balen, Kasse and Bohncke2017), tectonics (Houtgast & Van Balen, Reference Houtgast and Van Balen2000) and the erosion of older fluvial formations, are only partly understood. Therefore, we attempted to provide a relative chronological framework at best, by using available sources, such as historical maps. It must be noted that periods 1–6 become shorter in duration towards the present and thus do not represent equally long periods. Cohen et al. (Reference Cohen, Arnoldussen, Erkens, Van Popta and Taal2014) applied a similar series of decreasing time steps (nine instead of six) in their riverside archaeological predictive mapping (UIKAV). The longer the time period, the more landscape changes may have taken place, making it difficult to capture these in one time slice.
Units four and five in the GKM legend are the relatively old and young levee and floodplain deposits, and aeolian sediments respectively. Sand, silt and clay were deposited and conserved primarily, though not solely, on top of the (pre-)Younger Dryas terrace either as fluvial deposits or as dunes. The relatively old fluvial sediments include the flood deposits of the so-called Wijchen member, dated to the Preboreal and Boreal, as well as the presumed Subboreal levee and floodplain sediments. Dunes formed during the Younger Dryas are present on the eastern bank of the Younger Dryas floodplains (e.g. Kasse, Reference Kasse1995; Isarin et al., Reference Isarin, Renssen and Koster1997). These dunes primarily cover Lateglacial Interstadial or Late Pleniglacial terraces. Their parabolic shape may easily be recognised in the brown colours in the northeast from the present-day Meuse channel (Fig. 3). However, locally, for example in Lomm and Well, the dunes were observed within the Younger Dryas floodplain, albeit restricted in size (Ellenkamp & Ruijters, Reference Ellenkamp and Ruijters2015). The relatively young deposits comprise dunes reworked by wind in the Middle Ages (Dutch: ‘Stuifzanden’) and late medieval to present-day fluvial flood sediments.
As an example, we include two figures showing a cut-out from the GKM for the Ooijen–Wanssum area (northern Limburg) (Fig. 4) and for the area northwest of Sittard (southern Limburg) (Fig. 5). Figure 4 may be compared with the lidar DEM image (Fig. 3). Note, in red, the Interstadial (or older) terrace between the Younger Dryas terrace remnants and the Holocene channel belt with the present-day Meuse channel to the northeast and a Younger Dryas terrace on the southwest side. Figure 5 shows a relatively wide Holocene channel belt west of the present-day Meuse and a wide Younger Dryas terrace east of the Meuse. See Figure 6 for the legend of the GKM, showing the distinguished geomorphogenetic units.
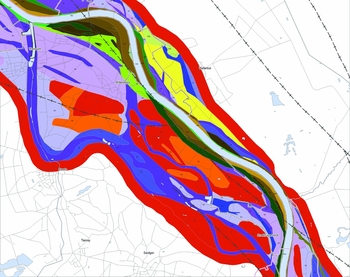
Fig. 4. Cut-out from the GKM: the Ooijen–Wanssum area, northern Limburg.

Fig. 5. Cut-out from the GKM: northwest of Sittard, southern Limburg.
Constructing the AVM
The AVM is in fact a series of five maps, i.e. a map for four archaeological periods and a cumulative map in which the four distinguished periodic maps are combined. The four archaeological periods distinguished by Groenewoudt & Smit (Reference Groenewoudt and Smit2014; see also Table 1) are:
-
• hunter-gatherers and early farmers: Late Paleolithic (12 000 BC) to Middle-Neolithic A (3400 BC). The AVM uses the abbreviation ‘JV’, after the Dutch ‘jagers – Verzamelaars’;
-
• early agricultural societies: Middle-Neolithic B (3400 BC) to Middle-Bronze Age (1500 BC). The AVM uses the abbreviation ‘LBV’, after the Dutch ‘LandBouwers Vroeg’;
-
• late agricultural societies: Middle-Bronze Age (1500 BC) to Early Middle Ages C (AD 900). The AVM uses the abbreviation ‘LBL’, after the Dutch ‘LandBouwers Laat’;
-
• state societies: Early Middle Ages C (AD 900) to present day (AD 1950). The AVM uses the abbreviation ‘SSL’, after the Dutch ‘StaatsSamenLevingen’.
Table 1. Geological and archaeological chronology of the Meuse valley geomorphogenetic (GKM) and archaeological (AVM) map series.

For each of these four periods, four main cultural themes were specified, according to Rensink & Van Doesburg (Reference Rensink and Van Doesburg2015):
-
• habitation, including defence, signified with the letter ‘W’ (Dutch: ‘Wonen’);
-
• burial signified with the letter ‘B’ (Dutch: ‘Begraving’);
-
• economy and infrastructure, signified with the letter ‘E’ (Dutch: ‘Economie’);
-
• rituals, signified with the letter ‘R’ (Dutch: ‘Rituelen’).
For both ‘economy and infrastructure’ and ‘rituals’ we distinguished between a wet (w) and dry (no code) setting variety.
It is beyond the scope of this article to describe in detail the conception of the AVM. The concepts are quite similar to those applied in the mapping project for the downstream part of the Meuse and the Dutch Rhine river branches: the ‘Archeologische Verwachtingskaart Uiterwaarden Rivierengebied’ (UIKAV; Cohen et al. Reference Cohen, Arnoldussen, Erkens, Van Popta and Taal2014). These authors applied a base prediction using landscape zonation polygon-by-polygon, and locally raised the scores on additional criteria (features noted on municipal maps, nationally protected areas). The actual implementation in our maps, however, differs: we also apply a base prediction based on landscape zonation, and for specific locations in the landscape (e.g. confluences of brooks with the Meuse terraces affecting distance to water source) further location-based scores are added (via buffer polygons calculated in GIS). We now stepwise focus on the main stages of the, strongly iterative, production process of the AVM.
The first step was identification of habitation and land-use models (Dutch: ‘Bewoningsbeelden’). The limited number of available excavated, well-documented and well-dated sites hampers an inductive way of constructing the AVM. Therefore, instead of focusing on the statistically established and quantitative habitation–landscape relation, we applied more qualitative habitation models, based on the generally accepted, generic relation between landscape (high, dry and safe versus wet and risky terrain) and human activities, such as habitation, hunting, agriculture, burial and rituals, in the past. The identification of the habitation and land-use models, based on and starting from the map units in the GKM (e.g. dune, pointbar from time period ‘1’ or relatively low part of the Younger Dryas terrace) and the four archaeological periods and cultural themes mentioned above, was one of the main tasks of the AVM team.
Secondly, to construct the habitation and land-use model and to calibrate the connection of the model to landscape units, we identified key sites and reference sites. Archaeological key sites are the best-documented and most informative archaeological sites within the research area. Reference sites are comparable sites, located outside the Meuse valley, but relevant for understanding the landscape–human-activity relation. At the same time, we assigned an archaeological base prediction to the GKM's geomorphogenetical units by applying generic and specific knowledge of the Meuse valley landscape and the expert-judgemental habitation models (see step 1). In practice, this step meant a simple ‘yes’ or ‘no’ to the question whether specific geomorphogenetic units were available to and used by humans during a specific period. Not surprisingly, GKM units such as ‘dunes located on relatively high parts of the Younger Dryas terrace’ turned out to be preferred locations for all of the four distinguished cultural themes or activities in all four periods.
The base prediction (step 3) is the first of two building blocks of the AVM. The second block is the systematic refinement of the basic prediction by specification (step 4) and operationalisation (step 5) of so-called supplementary location criteria. Evidently, the basic prediction is rather general, hampering statements about chances to find specific sites. Some of the GKM's units are in fact significantly large areas that require a more refined prediction model. We define supplementary criteria as the generic site-location factors related to the natural environment, such as the distance to open water sources or to a specific gradient zone. These landscape-related factors identify differences in human activities between and within geomorphogenetic units. For the AVM, supplementary criteria were defined for the habitation (W) and burial (B) themes only. Obviously, and in contrast to the two other cultural themes – economy and infra-structure, and rituals – activities related to habitation and burial require the best landscape positions. The supplementary criteria are:
-
1. distance to the (during that specific period) active Meuse;
-
2. distance to the mouth (confluence) of the Meuse tributaries;
-
3. distance to the next terrace level, often marked by a distinct and steep rise in height and vegetation change;
-
4. ‘dry and fertile’. This criterion describes the way specific units are drained, suited for agriculture and located high enough for safe habitation. The GKM legend implicitly includes sand sorting and grain size;
-
5. distance to the water source, other than 1 and 2.
For example, a value of 190 m for the distance to the Meuse, confluence and water sources other than the former two was calculated for the period of hunter-gatherers and early farmers, by statistically analysing all the predictive maps of Meuse valley municipalities.
The criteria were subsequently awarded weight, depending on their importance for and contribution to the choice of location in the past. For instance, we considered the distance to the active river in the periods of the hunter-gatherers and early farmers (‘JV’) and early agricultural societies (‘LBV’) of greater importance than in later times.
We ultimately generated the AVM maps by simply adding the scores of the basic and supplementary prediction. The higher the predictive scores, the higher the potential of finding sites from that specific period during field research. The maps were then validated in two stages using the national archaeological database, ARCHIS2. In the first stage, all ARCHIS records (i.e. 17,463 records, corresponding to a single find) within the study area were used to calculate the correlation between the appearance of period-specific sites and their corresponding predictive areas. Overall this showed a relatively good correlation, varying from 58% to 100%. In the second stage an edited version of the ARCHIS2 database was used. The raw dataset contains single finds, whereas the dataset of the edited version is narrowed to correctly dated sites gathered by archaeological excavations. This resulted in a dataset of 684 records, each representing a ‘pure’ archaeological site. In general, the correlation between an archaeological site and its AVM prediction is high for the base prediction (>72%). For the supplementary prediction, however, a clear distinction is observed for the older periods (JV and LBV) where the correlation is relatively low (35–64%) and the younger periods (LBL and SSL) where the correlation is relatively high (93–100%). There are several explanations for this, but most likely the effect of increasing uncertainty with increasing age (for knowledge about geomorphogenesis as well as archaeology) is the main factor. See Ellenkamp (Reference Ellenkamp2015) and Isarin et al. (Reference Isarin, Rensink, Ellenkamp and Heunks2015) for a detailed account of the validation process.
As an example, Figure 7 shows a cut-out from the AVM periodic map of hunter-gatherers and early farmers for the Ooijen–Wanssum area. The orange and reddish colours signify the zones with relatively high predictive values, i.e. near the active channel belt (white) and residual channels (light blue). The associated legend is presented in Figure 8.
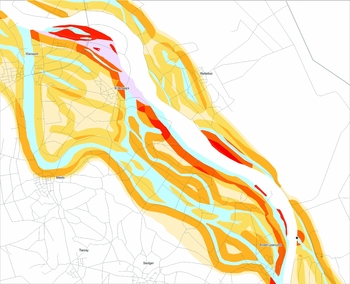
Fig. 7. Periodic map of hunter-gatherers (‘JV’). Cut-out from the AVM for the Ooijen–Wanssum area, northern Limburg.

Fig. 8. Legend of the AVM (see Fig. 7).
Discussion
The add-on value of the GKM and AVM map series, in comparison to traditional earth-scientific and predictive maps, results from the combined use of (1) information on landscape evolution (morphology, genesis, lithology, soil) and (2) habitation and land-use models specified for four different archaeological time periods. This integration enables the coupling of landscape use and human behaviour in the past with specific parts of the fluvial landscape of the Meuse valley through time.
It should be noted that for the AVM series, the scale of the predictive maps is an issue. The reliability of large-scale maps such as the GKM and AVM strongly depends on the availability of field data. Fieldwork intensity varies between areas, and even within one and the same research area. Consequently, the representativity of the collected data should be taken into account. The augering campaign in a plan area in Ooijen–Wanssum using a 17 × 20 m grid (Van Putten, Reference Van Putten2015) provided highly detailed lithogenetic information. In comparison, the number of landscape-reconstruction oriented cores in the preceding inventory phase was only 429 (Zuidhoff et al., Reference Zuidhoff, Huizer and De Jonge2012), as opposed to Van Putten's 3520 cores used to map both landscape details and archaeological site loci. Overall, it should be stressed that information from the northern section of the GKM (c. 83 Meuse kilometres, downstream of Roermond) is more accurate than the southern section. This is primarily due to the approximately 200 lithogenetic profiles (on average, every 500 m) referred to above. Extrapolations from these areas and interpretations are to a significant extent based on lidar DEM data. The more pronounced the height differences are, the more reliable the GKM map and therefore also the AVM are. On the other hand, a large flat area has fewer polygon boundaries than a highly accentuated terrain. As a consequence, this part of the map is more accurate and more reliable. The more categories of relative elevation there are, the more map reliability is hampered. In general, the differences in surface height decrease going downstream. In spite of these difficulties related to extrapolation and interpretation, we consider the landscape to be the principal basis for understanding and predicting human habitation and activities. Therefore, we believe that the underlying concepts of our landscape and predictive model are strong. Since the archaeological periods considered largely pre-date organised states, the high degree of ‘landscape determinism’ of the approach is defensible.
Ideally, the GKM and AVM are used by the Limburg and Noord-Brabant provinces and ‘Meuse municipalities’ and incorporated into their predictive maps (or at least used as a secondary reference) and archaeological policy. Initiators and spatial planners may also use the AVM, for it is relatively easy and straightforward to translate the periodic predictive maps into risk maps. The maps allow for avoiding the risk of delays and high budgets for investigations, and thus for a priori in situ conservation of archaeological sites. Although the maps may in the first place serve as a pragmatic tool for policy-making and optimising research strategy tools in heritage management, and as a basis for optimising spatial plans (water management, sand and gravel extraction and nature development), the scientific value of the GKM and AVM series is evident. The GKM makes a clearer discrimination within the Holocene floodplain, and between the Younger Dryas and Early Holocene geomorphological units, than hitherto available. Furthermore, during the conception of the maps the Early Holocene phase itself was better understood, whereas the Middle and Late Holocene were contrasted as being very different to the earlier phases.
Future research
The wide basis and collective acceptance for the predictive model underlying the AVM is certainly positive, but does not guarantee scientific quality in the long term. It is absolutely necessary that geological and archaeological data from future research be used as input for improvement of the models and ultimate maps. Available and future palaeoecological and chronological data should be incorporated to provide a better time control of both landscape changes and human activities (Hoek et al., Reference Hoek, Lammertsma, Bohncke, Bunnik, Kasse, Schokker and Westerhoff2017; Zuidhoff & Bos, Reference Zuidhoff and Bos2017). Moreover, the GKM and AVM map series cover the Holocene bottom of the Meuse valley and adjacent (sections of) Lateglacial terraces only, leaving the Interstadial and Pleniglacial landscape and its inhabitance out of consideration.
It may be noted that the ultimate archaeological predictive model was somewhat biased to the latest data (especially from Well–Aijen and Ooijen–Wanssum) and thus quite fresh at the time of adopting and applying it. Future archaeological research results must be used to test the validity of the models and underlying assumptions. New data may show differences in habitation patterns and site distribution between the various landscapes of the 165 km long Meuse valley.
Due to the increased effort to understand the landscape, the different phases of pointbar and levee formation have only recently been recognised. The lack of time control is reflected in the GKM and AVM by the modelled and fairly rigid distinction of six phases. Testing of both maps is a crucial part of Malta-based archaeology in the Limburg Province and its Meuse municipalities and, ideally, those of the province of Noord-Brabant and the neighbouring parts of Belgium. This testing requires adequate dating of archaeological sites and sediments, for example by ceramic or flint typology, accelerator mass spectrometry (AMS) and optically stimulated luminescence (OSL) dating. Moreover, it is required that lidar DEM data from the Belgian part of the Meuse valley are incorporated in a new version of the GKM.
The increased accuracy of geomorphology mapping, thanks to the lidar data, reveals a stronger sense of the lack of local dating. However, Malta-based archaeology research programmes may not provide all the chronological answers we require in the near future. We therefore still need upgraded geomorphogenetic understanding, for which the GKM is a starting point. At the same time, archaeological landscape occupancy models for different time periods need constant improvement, for which the AVM series and methodology provide a starting point.
Finally, it is crucial that researchers can notify their observations at a central point, to enable modifications and improvements to both maps and the underlying landscape and archaeological model. If not, the maps lose their dynamics and validity, turning the predictive archaeological map series into a more traditional static one.
Acknowledgements
We are grateful for the very useful comments of Dr K.M. Cohen (Utrecht University) and an anonymous referee. The authors would also like to thank the members of the GKM and AVM team for their expertise and their willingness to share their knowledge in this project. The GKM team members are: Drs Jurgen de Kramer (EARTH), Drs Rob Paulussen (ArcheoPro), Dr Ir. Leo Tebbens (BAAC) and Dr Frieda Zuidhoff (ADC ArcheoProjecten). We thank Dr Cees Kasse (VU) and Drs Marlies Janssens (VU) for their assistance with and contribution to the GKM project. The AVM team consisted of the GKM team, supplemented with Drs Fred Brounen (RCE), Drs Jos Deeben (RCE, ✝), Drs Tessa de Groot (RCE), Drs Jan Willem de Kort (RCE), Drs Fokko Kortlang (ArchAeO), Drs Jan Roymans (RAAP Zuid-Nederland), Dr Liesbeth Theunissen (RCE), Dr Leo Verhart (Stone Age) and Drs Ellen Vreenegoor (RCE).