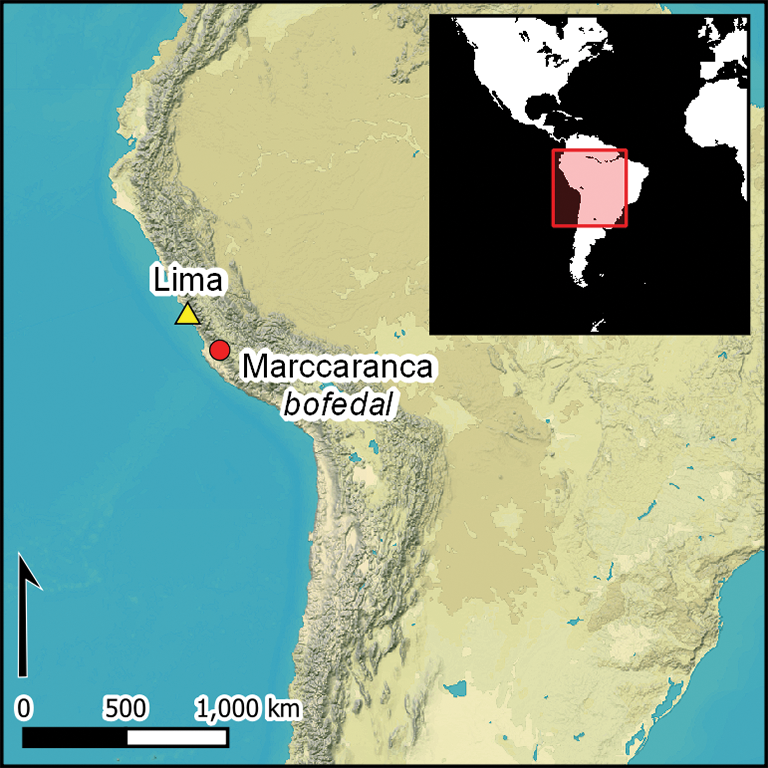
Introduction
Humans have been altering the physical environment for over 12 000 years and the Andes are no exception (Denevan Reference Denevan1992; Ellis et al. Reference Ellis2021). In the seemingly bleak, suni-puna alpine-tundra of the high Andes, pre-Hispanic populations used and developed existing natural moorlands and wetlands—the latter known as bofedales in the Central Andes or vegas in the Southern Andes (Lane Reference Lane2006). Here, we examine how these pre-Hispanic human populations created or augmented bofedales. This was usually achieved through the construction of canals or walled silt-traps in order to improve water and soil retention, thus providing more ample and better-quality pasturage. Dating such structures, especially silt-traps, however, is fraught with difficulty, “given [the] disturbance by erosion and deposition, by the process of agriculture, and by frequent rebuilding” (Denevan Reference Denevan2001: 172) that characterises their construction and use.
The results from an ongoing research project in the upper Ica drainage in southern Peru have revealed a series of walled silt traps or check dam-type structures, known as silt reservoirs. These were built to withhold silt and water, leading to peat and wetland growth at the summit of the Quebrada Marccaranca, where it opens into a natural basin. At some point in the past, the construction of these silt reservoirs led to the anthropogenic alteration of this natural basin, increasing the size of the wetlands. A series of three borehole samples and six radiocarbon dates from the basin's peat levels reveal that this anthropogenic alteration probably occurred during the Late Horizon or Inca period (AD 1400–1532), before the area's abandonment by the end of the eighteenth century.
In this article, we describe the use of silt reservoirs in the Andes, focusing particularly on the highest reaches of the upper Ica drainage, before charting the probable history of construction and use of these structures in the Quebrada Marccaranca. Finally, we consider the reasons for investment in these hydrological structures during the Inca period, analysing potential issues of imperial management of resources, land and surplus.
Anthropogenic wetlands in the Andes
Bofedales are poorly drained, high-altitude peatlands replete with cushion-like plants, such as Distichia muscoides, which, in turn, permit the growth of high-quality, softer grasses, including Calamagrostis crysanthan and Calamagrostis rigescens (Squeo et al. Reference Squeo, Warner, Aravena and Espinosa2006; Maldonado Fonkén Reference Maldonado Fonkén2014). These wetlands and their rich plant communities are found almost exclusively within the puna ecozone—an alpine-tundra grassland located above 4000m asl in the Central Andes. Bofedales provide an excellent herding habitat that is highly coveted by modern-day pastoralist communities (Browman Reference Browman, Galaty and Johnson1990); this was likely the case in the past, as well.
The puna is considered a critical resource for Andean highland communities, constituting 30 per cent of the Central Andean landscape; within this, bofedales constitute 2.5 per cent of the puna (Ravines Reference Ravines1978; Kuznar Reference Kuznar1995: 36; Young et al. Reference Young, León, Herrera-MacBryde and Davis1997). Even though bofedales provide optimum pasture for grazing, their use for herding is seasonal and generally restricted to the dry months just before the rains—usually between June and September. At other times of the year, the natural suni-puna pasture is normally sufficient for the needs of domesticated animals.
Given that the importance of bofedales is compounded by their relative scarcity, there has been a long tradition among Andean communities of enhancing these natural wetlands in order to increase their size and to improve the quality of the pasturage (e.g. Kuznar Reference Kuznar1995; Palacios Rios Reference Palacios Rios and Morlon1996; Carhuallanqui Reference Carhuallanqui1998; Quesada & Lema Reference Quesada and Lema2011; Verzijl & Guerrero Quispe Reference Verzijl and Guerrero Quispe2013). As the sixteenth-century Indigenous chronicler Felipe Guaman Poma de Ayala (Reference Poma de Ayala and Pease1993: 780) notes in his admonition to King Philip III of Spain, indigenous water management systems needed to be maintained—systems that canalised “water for irrigating said fields, even the pasture of the herds they irrigated in the highlands and in the valleys” (authors’ translation).
Archaeologically, these systems have been difficult to detect, mainly due to the often-ephemeral nature of the technologies used to irrigate these pastures artificially, such as the use of unlined canals cut into the fresh sod, and also as a result of continued siltation. Nonetheless, the use of such systems to enhance Andean wetlands has been suggested to have commenced from at least the Middle Horizon (AD 600–1000) onwards and has been linked to the rise of the Tiwanaku state in that period (Vining & Williams Reference Vining and Williams2020: 13). Domic and colleagues (Reference Domic2018: 13) have sought to push back the start of anthropogenic wetland intervention in northern Chile even further, to 2000 years ago.
Among the varied types of infrastructure, Lane (Reference Lane2009: 177–78) identifies silt reservoirs—principally composed of low walls and sluices—as a particular pre-Hispanic form of terrace analogous to check dams or cross-channel terraces documented in the general literature (Denevan Reference Denevan2001: 75) but with one key difference. Check dams and cross-channel terraces are usually found in the middle or lower reaches of side valleys and ravines to counteract the effects of erosion, as well as regulating the flow of water and trapping silt. Check dams of this type were first documented by Regal Matienzo (Reference Regal Matienzo2005: 72) in the Río Chira Basin in 1970, while other instances were noted by Donkin (Reference Donkin1979) in Ecuador, Chile and north-west Argentina. In the latter region, for example, a recent study by Zuccarelli Freire and colleagues (Reference Zuccarelli Freire, Meléndez, Oviedo and Quesada2021) highlights the use of these features across narrow gullies to counteract the effects of land erosion.
Although the silt reservoirs we have previously identified in the Ancash highlands are similar types of structure, they are located at a much higher altitude—generally at the upmost level of a drainage, or along its upper reaches (Lane Reference Lane2009). The altitude at which these features are found underlines their basic function; aside from controlling water and sediment, they provide the technical means through which to enlarge or create artificial wetlands with enriched pasturage for domesticated animals—in this case, llamas and alpacas.
That these features are also recorded at much lower elevations, within the upper limits of modern-day agriculture, demonstrates that the range of high-altitude Andean pastoralism was far wider in the past than today (Lane & Grant Reference Lane, Grant, Capriles and Tripcevich2016). Documented in the north-central Andean highlands, as well as in the Socaire region of the Chilean Altiplano (Donkin Reference Donkin1979: 126; Lane Reference Lane2009: 177–78), these features are located in the suni (transitional farming-herding zone: 3500–4100m asl) and puna (alpine-tundra herding grassland: 4100–5000m asl) ecozones of the Andes. Here, we analyse analogous silt reservoirs discovered in the Marccaranca bofedal in the upper Ica drainage of the south-central Andes. As with the north-central Andean examples, the defining feature of these structures is their capacity to store water, manage sediments and generate a micro-environment in which improved pasturage provided the means for successful camelid herding.
SAN 3: the Marccaranca bofedal
The Marccaranca bofedal (13°46´12.9″S, 75°12´36.2″W) is located within the puna ecozone, at approximately 4050m asl. At the top of the eponymous side valley and stream—a headwater tributary of the Ica River—the bofedal is found within prime herding territory, as attested by the presence of numerous abandoned corrals (most likely of pre-Hispanic and early modern date) (Figures 1 & 2). In its present state, the bofedal covers an area of 8ha. Today there are still two sizable alpaca herds (>250 head) based in the vicinity.

Figure 1. Map of Peru, showing the location of the study area (figure by L. Coll).

Figure 2. Map of the study area, showing the Chocorvos-Inca-Spanish Colonial settlement site of Viejo Sangayaico (SAN 1a-e), bofedal of Marccaranca (SAN 3) and pre-Hispanic ritual platform (ushnu), and the present-day calvary of Huinchocruz (SAN 4) (figure by L. Coll).
The natural Marccaranca bofedal has been augmented by six silt reservoirs (A–F), covering two spring gullies (A–B & C), a central area (D–E), and, finally, the bofedal stream outlet (F), downslope through the Marccaranca Valley (see Figure 3). The springs feeding into silt reservoirs A–B and C emerge from the Incahuasi granodiorite bedrock uplands that form the prevailing geology at this location. The underlying geology of the bofedal and the area of Incahuasi granodiorite is a phaneritic-textured, intrusive, igneous rock that is similar to granite but containing more plagioclase than orthoclase feldspar (Palacios Moncayo Reference Palacios Moncayo1994).
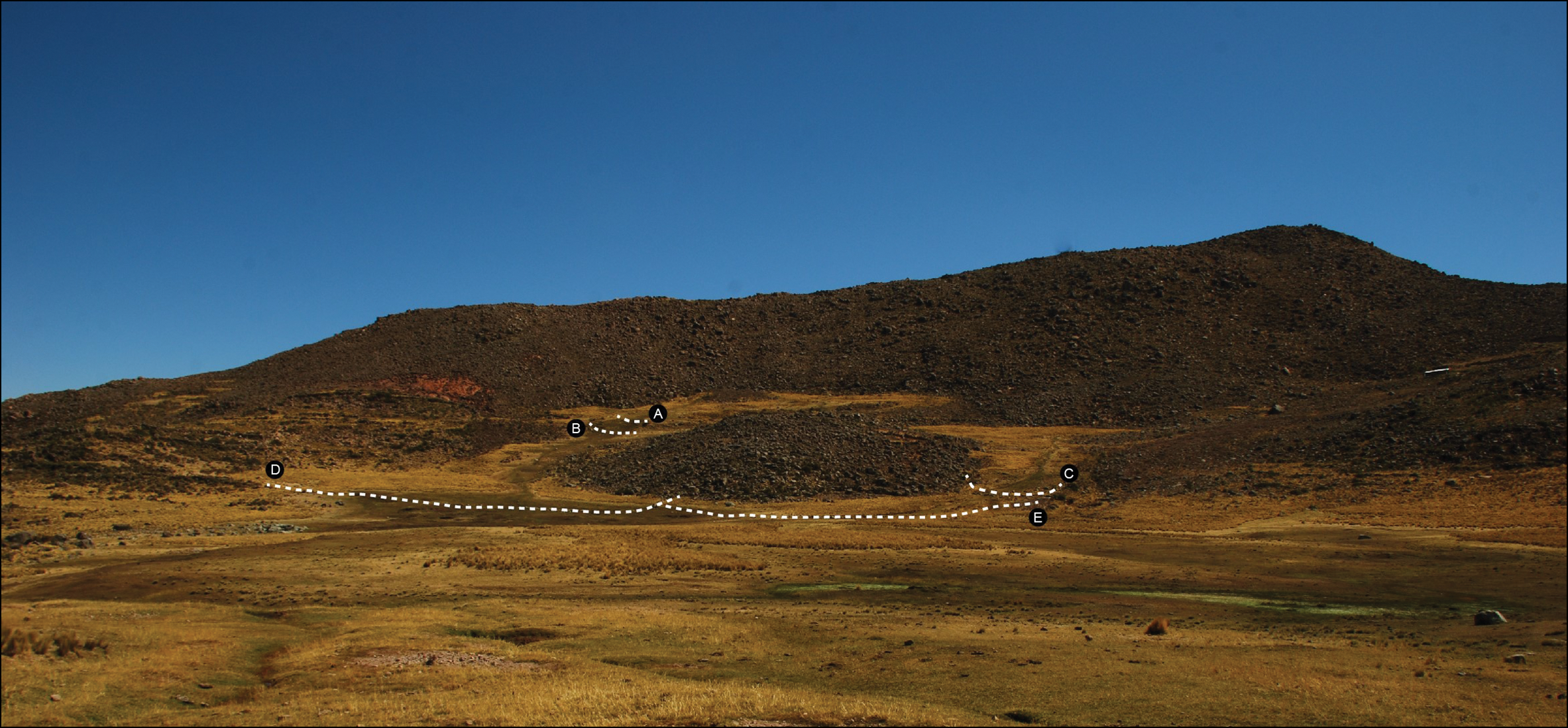
Figure 3. The Marccaranca bofedal (SAN 3), showing silt reservoirs A–E (photograph by K. Lane).
Incahuasi granodiorite has a low relative porosity of 0.55–0.59 per cent, with a crack porosity similar to that of Andesite (10–20 per cent) (Schild et al. Reference Schild, Siegesmund, Vollbrecht and Mazurek2001: 118). Thus, in any bofedal basin it underlies, water tends to pool while still seeping through natural cracks, replenishing underground aquifers and springs located downslope. It is therefore likely that this bofedal was altered for the dual purposes of expanding wetland pastures and providing an increase in water for geological storage and subsequent use in terrace agriculture downslope.
In general, while accumulating peat has buried large parts of the silt reservoir structures, in some areas, it is possible to see that the original walls would have been double-faced, infilled with clay and smaller stones, with a maximum thickness of between 1.2 and 1.4m. The reservoir walls, built on the then-existing ground surface, are composed of irregular stone coursing, with larger foundational stones at the base and middling to smaller stones towards the top. The coursing was held together by a compact, silty-clay mortar. In many places, the upper sections of the reservoir have collapsed, leaving only the large base stones visible above ground. The maximum height above the ground surface of these surviving structures is 1.4m, although in most cases, only approximately 0.5–0.7m is currently preserved (Figure 4). A test-pit excavation near to profile 2 (see Figure 5) revealed that silt reservoir E's wall reached a depth of 0.7m, providing an indication of the depth of siltation since construction—at least potentially for those located in the bofedal basin (D–F). The wetness of the reservoirs’ exterior walls demonstrates that, while maintaining wet conditions behind them, excess water would have seeped through.

Figure 4. Detail of silt reservoir E, with Sturt Fraser, showing maximum preserved wall height (photograph by K. Lane).

Figure 5. Drone flight panoramic and plan of SAN 3, with profile sediment descriptions (figure by L. Coll).
Silt reservoirs A, B and C are located across the two small spring ravines that feed into the main bofedal basin (Figure 6). Silt reservoirs D and E are found below A, B and C to capture water and sediments from the western side of the bofedal. Silt reservoir E abuts D, suggesting that it was built later, although the time elapsed between their construction is unknown. Finally, silt reservoir F is positioned at the lower southern edge of the bofedal, prior to it entering the upper section of the Marccaranca Valley. Silt reservoir F is, therefore, foundational in shoring up the bofedal and facilitating water and soil storage in the larger bofedal basin. All the silt reservoirs utilise existing freestanding Incahuasi granodiorite blocks where present, with the northern end of D, E and F anchored directly onto outcrops of the same rock, lending these structures additional support. The end sections of silt reservoirs A, B and C are freestanding.

Figure 6. Detail of silt reservoir C, showing the extent of the bofedal area behind the reservoir wall (photograph by K. Lane).
Discussion: peat, pollen and dates
During fieldwork conducted in 2013, three borehole profiles were taken from the Marccaranca bofedal (Figure 5). Profiles 2 and 3 were located behind silt reservoir E, with profile 2 flush against its inner wall and profile 3 located 5m to the north-west of profile 2. Profile 6 was sited approximately 50m from profile 2, in the middle of the bofedal, and some 75m north of silt reservoir F. Two radiocarbon dates were obtained for each borehole profile (Table 1). In 2018, a small test-pit was dug alongside the profile 2 borehole to ascertain the depth of the wall at this point. A radiocarbon date from the base of this wall was then used to estimate the probable construction date of silt reservoir E.
Table 1. Radiocarbon dates from borehole samples from the Marccaranca bofedal. All samples are peat. δ13C estimated by IRMS. Dates calibrated with OxCal v4.4.4 (Bronk Ramsey Reference Bronk Ramsey2021) using a mixed calibration curve (Marsh et al. Reference Marsh2018) of SHCal20 and IntCal20 (Reimer et al. Reference Reimer2020). Medians and ranges rounded by 10 years.

The data from all three boreholes confirm the anthropogenic manipulation of the Marccaranca bofedal starting in the pre-Hispanic period. Profile 6, moreover, is particularly significant, as it records a sequence of five distinct stages of change in the composition of the Marccaranca bofedal basin over an even longer period of time (Figure 6): 1) an initial humic and peat deposition episode; 2) humic lenses and gritty silting; 3) a black peat episode; 4) a grey silt of a slowly in-filling lake; 5) a final episode of peat formation and gritty lens, creating the bofedal we see today.
The two radiocarbon dates from profile 6, obtained at depths of between 1.57–1.60m and 2.16–2.20m, date the lower section of this profile to 5240 cal BC (5320–5060 BC, 95% probability; OxA-32393) and 5680 cal BC (5740–5620 BC, 95% probability; OxA-32394), respectively (Table 1). These dates record, respectively, the episode of black peat (Stage 3) and the basin's initial peat level (Stage 1), demonstrating that bofedal-type conditions have persisted here for the last seven millennia. Later episodes of silting evident in the profile demonstrate the region's dynamic environment. It is possible that the slow infilling lacustrine environment represented by Stage 4 was caused by construction of the silt reservoirs, thus leading to the retention of greater volumes of water in this part of the basin. Subsequent peat formation reveals the stabilisation of the basin's ecological system, leading to its present-day state.
Profiles 2 and 3 (Figure 6), located on the edge of the bofedal, are much more homogeneous than profile 6, showing base levels of fine gravel (like profile 6), followed by long sequences of brown or black peat up to the present day. Figure 7 shows that the deepest parts of profiles 2 and 3 have median radiocarbon dates of 1420 cal BC (1500–1300 BC, 95% probability; OxA-32498) and cal AD 740 (AD 670–880, 95% probability; OxA-32392), respectively (Table 1). These pre-date the creation of silt reservoir E and demonstrate that wetland conditions here persisted through the Late Holocene up to the end of the first millennium AD.

Figure 7. Top) drone flight panoramic and plan of SAN 3, indicating the position of silt reservoirs and profiles; bottom) section of profile locations and radiocarbon samples (figure by L. Coll).
The latter two samples from profiles 2 and 3 date the use of the bofedal after the building of silt reservoir E. The profile 3 date has a median of AD 1850 (AD 1690–1930, 95% probability; OxA-32352), showing continued peat formation from the eighteenth to the twentieth century (Figure 7). The median of the profile 2 date is cal AD 1440 (1410–1460, 95% probability; OxA-32351), which comes from the base of the silt reservoir E wall and provides a critical terminus ante quem date range for the building of this structure within the first half of the fifteenth century—sometime during the early Inca period.
Recent scholarship has endeavoured to show that the traditional document-based Inca chronology (Rowe Reference Rowe1945) does not reflect the actual time depth of imperial expansion (e.g. Ogburn Reference Ogburn2012; Marsh et al. Reference Marsh, Kidd, Ogburn and Durán2017). Indeed, recent dates from the nearby southern coast Acari Valley suggests an Inca presence as early as AD 1430–1460 (Valdez et al. Reference Valdez, Bettcher and Huamaní2020: 1569), significantly earlier than Rowe's ethnohistorically informed AD 1476 date (see also Bongers et al. Reference Bongers, O'Shea and Farahani2018: 227 for the mid-Chincha Valley immediately to the north). These studies suggest Inca expansion into the region by the early to mid fifteenth century, which agrees with our profile 2 date that marks the construction of at least some of the bofedal infrastructure at Marccaranca. This is key, given the wider evidence for Inca investment in hydraulic infrastructure across the Andes (e.g. Regal Matienzo Reference Regal Matienzo2005). The possibility of imperially driven construction at the Marccaranca bofedal is lent further support by the proximity of the nearby Inca Road and the important Inca occupation at Viejo Sangayaico (SAN 1), located just 3km immediately downslope in an area of extensive bench terrace construction (Lane et al. Reference Lane2016).
Pollen samples were also taken at 40mm intervals from all three profile cores. Selected sub-samples were processed using standard techniques for the extraction of sub-fossil pollen and spores (Moore et al. Reference Moore, Webb and Collinson1999). Samples of 1.5ml were analysed and a sum of 300–400 pollen grains plus spores was counted for each sample. Pollen flora were identified using published works on regional pollen and a comparative collection from the Palaeoecology Laboratory at the University of Southampton, UK. The pollen data are plotted using Tilia and Tilia View (TiliaIT 2021), with pollen calculated as a percentage of the total pollen and spores as a percentage of the sum + spores (Figures 8–10). The few tree/shrub taxa are plotted as a group (left of each diagram), while the dominant herb flora are plotted in alphabetic order of family.

Figure 8. Profile 2 pollen diagram, with calibrated radiocarbon medians. Dates calibrated with OxCal v4.4.4 (Bronk Ramsey 2021) using a mixed calibration curve (Marsh et al. 2018) of SHCal20 and IntCal20 (Reimer et al. 2020) (figure by R. Scaife).

Figure 9. Profile 3 pollen diagram, with calibrated radiocarbon medians. Dates calibrated with OxCal v4.4.4 (Bronk Ramsey 2021) using a mixed calibration curve (Marsh et al. 2018) of SHCal20 and IntCal20 (Reimer et al. 2020) (figure R. Scaife).

Figure 10. Profile 6 pollen diagram, with calibrated radiocarbon medians. Dates calibrated with OxCal v4.4.4 (Bronk Ramsey 2021) using a mixed calibration curve (Marsh et al. 2018) of SHCal20 and IntCal20 (Reimer et al. 2020) (figure by R. Scaife).
All three profiles show clear evidence of bofedal-type plant communities—dominated by plants from the Poaceae, Plantago, Cyperaceae and Asteraceae families—persisting from the Middle Holocene (profile 6) through to the present day (profiles 2 and 3). Because of their relative proximity, profiles 2 and 3 show clear correlations; for instance, in their high counts of Poaceae and Asteraceae (the latter is documented as Tubuliflorae indet. in the profile pollen diagrams), between their stages 2 and 3, respectively. The upper levels of profile 2, stage 3, however, contain high counts of Cyperaceae and Plantago, which are apparently not reflected in the uppermost levels of profile 3, stage 4, probably due to local variations in the bofedal's vegetation.
Strikingly, all the profiles show evidence for maize (Zea sp.) pollen in pre-Hispanic contexts. Self-pollinating maize produces a very low pollen rain of heavy grains, which is never dispersed far from the plant. Its presence here therefore suggests its import from lower altitudes for consumption by herders, for foddering animals (as is the case today; McCorkle Reference McCorkle and Browman1987), or perhaps even high-altitude cultivation. The cultivation of maize pollen could be possible in unused corrals using guano-enriched soils, with the corral walls and the proximity of the bofedal providing a buffer against the wind and cold. Similar shared use of space for herding and farming is recorded throughout the Andes, including cultivation of maize and potato in the seasonal qochas or ponds of the circum-Titicaca Basin at nearly 4000m asl (Flores Ochoa et al. Reference Flores Ochoa, Paz Flores, Rozas and Morlon1996; Craig et al. Reference Craig2011).
While bofedal-type grasses, sedges and herbs, such as Plantago and Asteraceae, dominate these pollen sequences, in profile 2 there is a marked decline in Plantago and wetland sedges (Cyperaceae) that is accompanied by a corresponding peak in grasses (Poaceae). This change occurred after the silt reservoir's construction and is dated to cal AD 1440 (AD 1410–1460, 95% probability; OxA-32351; see Figure 8). This sequence is precisely what one would expect, given that increased grass production would have enhanced the herding potential of the bofedal. Such bofedal enhancement under Inca auspices has been documented for the Inca period (Chepstow-Lusty et al. Reference Chepstow-Lusty2009). Subsequently, grasses decline, and sedges increase (see Chepstow-Lusty et al. Reference Chepstow-Lusty2009: 779–80), presumably as a result of the system falling into disuse following a catastrophic collapse in camelid numbers triggered by the introduction of Old World animals and animal diseases during the early Spanish colony (Flores Ochoa Reference Flores Ochoa, Milliones and Tomeada1980). Profile 3, however, appears to be comparable with a lower-resolution chronology (see Figure 9).
Conclusions
The Marccaranca bofedal is one component of a landscape transformed through time, first by the native Chocorvos, a Late Intermediate Period (AD 1000–1400) ethnic group (Rowe Reference Rowe and Steward1946: 188), and then by the Inca (AD 1400–1532). Our data suggest that at least one, and therefore possibly some of the other silt reservoirs at Marccaranca, date to the early Inca period—tracking other imperial developments in the basin, including the occupation of Sector B at Viejo Sangayaico (SAN 1 in Figure 2; Lane et al. Reference Lane2016), which is located only 3km downslope and which has extensive associated agricultural terracing (Nanavati et al. Reference Nanavati2016).
At 3650m asl, the multi-period site of Viejo Sangayaico is located precisely at the transition between the agricultural suni and herding puna ecozones, thereby providing the setting for multiple social, economic and religious interactions between their respective herder and farmer communities (sensu Parsons et al. Reference Parsons, Hastings and Mendieta1997). Once incorporated into the empire, Viejo Sangayaico was the main Chocorvos and Inca settlement, controlling south–north access along the section of Inca Road that ran adjacent to the Marccaranca bofedal and linked the upper Ica drainage to the northern Pisco drainage. The latter is a particularly important transit route that connected the highlands and the coast with major road and imperial infrastructure, including the administrative centres at Tambo Colorado and Huaytará (Bueno Mendoza Reference Bueno Mendoza2003; Serrudo Torobeo Reference Serrudo Torobeo2010).
The Marccaranca bofedal thus represents a key element within this wider region of rich ecological resource zones, which included agricultural terraces, along with natural bofedales and pastures—all providing surplus and supplies for the imperial requirements in the Pisco drainage and beyond. This interpretation is supported by ethnohistorical documentation of the Chocorvos shifting their powerbase from the upper Ica to the Pisco drainage, in accordance with the particularly strong Inca state presence there (Espinoza Soriano Reference Espinoza Soriano2019: 993–1000).
The Marccaranca bofedal's pollen record provides another line of evidence for how imperial investment in this landscape expanded pasture. The presence of maize pollen here at altitudes above 4000m asl hints at attempts to intensify production of this important pre-Hispanic staple crop by pushing its extreme ecological limits (Staller Reference Staller2016).
A neo-Wittfoglian position, while questioning the primacy of the state in initiating large hydraulic engineering projects, nevertheless advocates the subsequent intervention of the state in community-based systems geared towards increased production (cf. Wittfogel Reference Wittfogel1957; see Stanish Reference Stanish1994; Lane Reference Lane2009). Here, we propose just such a model, whereby Chocorvos productive resources, such as terraces, canals and bofedales, were systematised and enlarged via Inca imperial intervention across the entire continuum of hydrological manipulation. In effect, Inca hegemony in the upper Ica drainage seems to have been directed towards a politico-economic objective, attained through engineering and infrastructure investment in settlements, terraces, roads and pasture, and aimed towards the satisfaction of the imperial body-politic, which is especially evident throughout the all-important Pisco drainage that connected the Empire's highland heartland to its coastal hinterlands.
Elsewhere in the Americas, similar state or imperial intervention and enlargement of community-based systems have been suggested for the highland Middle Horizon (AD 600–1000) Tiwanaku polity and the coastal Late Intermediate Period (AD 1000–1400) Chimú state (Graffam Reference Graffam1992; Ortloff Reference Ortloff, Scarborough and Isaacs1993). Further afield in Mesoamerica, Mayan (400 BC–AD 1000) state action has also been posited as complementing local forms of water management (Wyatt Reference Wyatt2014). Indeed, the incidence of top-down intervention over local water-management systems seems to be a constant across the world, especially when the area in question was critical to a state or imperial polity. In this regard, the Roman Empire and its successor state in the east, Byzantium (27 BC–AD 1453), understood the importance of harnessing water as both a resource and a politico-ideological tool to further propagate the state (Wilson Reference Wilson2012).
Likewise, this has been observed in ancient India, China and elsewhere (e.g. Wu et al. Reference Wu2019; Kumar Singh et al. Reference Kumar Singh, Dey, Jaina and Mujumdar2020), suggesting that while Wittfogel (Reference Wittfogel1957) was incorrect in his contention that water infrastructure investment was the sole prerogative of ancient states and empires (see also Steward Reference Steward1955), he was correct in gauging the capacity for powerful polities to restructure and increase productivity through recourse to hydraulic engineering, leading to an expansion in state outreach and control. Similarly, in the present day, there seems to be a return to big-state investment in hydraulic engineering, this time more closely tied to local initiatives, sometimes as a means to counter the ever-increasing threat of climate change (Crow-Miller et al. Reference Crow-Miller, Webber and Molle2017). It would therefore seem useful for archaeologists to revisit the wider implications of Wittfoglian theory concerning the primacy of water management for ancient (and not so ancient) polities, endeavouring, in each case, to disentangle the political ecology underlying state intervention, regardless of whether such intervention was ultimately successful or not.
Acknowledgements
We thank all the members of the Proyecto de Investigación Arqueológica Cuenca de Ica (PIACI) and One River Project, as well as the local community of Sangayaico for all their help in making this project possible. Permission for the project was granted by the Ministerio de Cultura del Perú under RD nos 061-2013-DGPA-VMPCIC/MC and 430-2018-DGPA-VMPCIC/MC.
Funding statement
Funding for the project was obtained from the Leverhulme Foundation (UK [Project Code: RPG-17]: 2012–2016) and the Gerda Henkel Foundation (Germany [Project Code: AZ-30-F-17]: 2018–2021).