Introduction
Rice is one of the most important crops grown in Arkansas, with over 639,000 ha planted in 2016. The state of Arkansas produces most of the rice grown in the United States, with more than twice the acreage of California, the second-place state (NASS 2016). Weed control is a major obstacle to rice production; the major weeds of Arkansas rice are barnyardgrass, sprangletops (Leptochloa spp.), red rice (Oryza sativa L.), northern jointvetch [Aeschynomene virginica (L.) Britton & al.], Palmer amaranth (Amaranthus palmeri S. Wats.), and broadleaf signalgrass (Norsworthy et al. Reference Norsworthy, Bond and Scott2013). Herbicide resistance adds to the difficulty of achieving adequate control of barnyardgrass and red rice. In Arkansas, barnyardgrass has evolved resistance to propanil, quinclorac, clomazone, and acetoacetate synthase (ALS)–inhibiting herbicides (Talbert and Burgos Reference Talbert and Burgos2007; Norsworthy et al. Reference Norsworthy, Bond and Scott2013).
To combat the pressure placed by herbicide-resistant weeds on current production systems, growers need new technologies. Rice with resistance to quizalofop, an acetyl-CoA carboxylase (ACCase)–inhibiting herbicide, is expected to be commercialized in the United States in 2018. Provisia™, the name of this technology, will be associated with both the herbicide-resistant rice trait and the commercial quizalofop product labeled for use. The use rate for quizalofop in quizalofop-resistant rice will range from 100 to 138 g ai ha–1 for single applications and 240 g ai ha–1 for maximum yearly application (Anonymous 2017). Quizalofop, a systemic herbicide, is most notably used in soybean [Glycine max (L.) Merr.] for POST control of annual and perennial grasses; however, it can provide moderate residual grass control (Shaner Reference Shaner2014). It is anticipated that quizalofop will be restricted solely to POST applications in quizalofop-resistant rice (Youman et al. Reference Youman, Guice, Rhodes, Schultz and Harden2016).
ACCase-inhibiting herbicides are commonly used in multiple crops to selectively control annual and perennial grass species. These graminicides inhibit the enzyme acetyl-CoA carboxylase, an integral step in fatty acid synthesis. Eventually, this inhibition blocks the production of phospholipids needed for cell growth (Shaner Reference Shaner2014). Sethoxydim, clethodim, fluazifop, and quizalofop are commonly used in broadleaf crops (Anonymous 2003a,b, 2009, 2015a,b), mainly because broadleaf plants are naturally tolerant to ACCase-inhibiting herbicides (Konishi and Sasaki Reference Konishi and Sasaki1994). Broadleaf species owe this tolerance to their possession of the herbicide-tolerant prokaryote form of ACCase from the accD gene; lacking this gene, grass species are sensitive to ACCase-inhibiting herbicides (Konishi and Sasaki Reference Konishi and Sasaki1994). Although ACCase-inhibiting herbicides have shown high efficacy against grasses, differing levels of tolerance across grass species have been observed. These differences have led to the labeling of cyhalofop and fenoxaprop for POST use in rice (Anonymous 2003b, 2016). The tolerance in rice to cyhalofop and fenoxaprop is due to reduced absorption through the cuticle and enhanced metabolism of the herbicide compared to other susceptible grass species (Ruiz-Santaella et al. Reference Ruiz-Santaella, Heredia and Prado2006).
Although graminicides are generally not applied PRE or for residual weed control, it is known that they do have limited residual activity (Barber et al. Reference Barber, Norsworthy and Scott2015). Persistence and efficacy of an herbicide in soil largely dictate the length of a plant-back interval following application. Herbicide persistence in soil can have an effect on prolonged weed suppression or can cause carryover effects in a subsequent crop (Ogle and Warren Reference Ogle and Warren1954). The length and extent of activity of residual herbicides may depend on both soil moisture and soil texture, among other soil chemical properties. Generally, soil-applied herbicides need 1.3 to 1.9 cm of precipitation for optimum activation (Riar et al. Reference Riar, Norsworthy, Bararpour, Bell and Schrage2012). Activation is the movement of an herbicide into the soil profile, where it can come into contact with the germinating seed (Knake et al. Reference Knake, Appleby and Furtick1967). Smith et al. (Reference Smith, Ferrel, Webster, Fernandez, Dittmar, Munoz and MacDonald2016) determined that efficacy of S-metolachlor on Palmer amaranth was greatest when 0.6 and 1.3 cm of irrigation were applied compared to a nonirrigated check. Specific herbicides with high water solubility can move with water through the soil in the presence of rainfall or irrigation. Hence, it is possible to lose an herbicide via runoff or leaching if too much water is present (Friesen Reference Friesen1965). However, this movement is also influenced by an herbicide’s Kd (soil sorption) and Koc (soil organic carbon sorption), which can bind an herbicide to soil particles and organic matter (Wauchope et al. Reference Wauchope, Yeh, Linders, Kloskowski, Tanaka, Rubin, Katayama, Kordel, Gerstl, Lane and Unsworth2002).
Generally, plant-back intervals to monocot (grass) crops range from 30 to 120 d following most ACCase-inhibiting herbicide applications (Barber et al. Reference Barber, Norsworthy and Scott2015; Anonymous 2003a,b, 2009, 2015a,b, 2016). However, previous research on ACCase-inhibiting herbicides has shown no significant residual herbicidal activity onto subsequent grass crop plantings (Mahoney et al. Reference Mahoney, Shropshire and Sikkema2016; Spader et al. Reference Spader, Lopes, dos Santos, Mendonca and Pelissari2012). Planting within graminicide plant-back intervals would be unlikely in the Mid-South; however, crop failure after a graminicide application could limit subsequent planting options. Likewise, the occurrence of glyphosate-resistant grass weeds in the Mid-South could also cause a decreased time between ACCase-inhibiting herbicide application and the planting of a sensitive crop. Glyphosate-resistant ryegrass [Lolium perenne ssp. multiflorum (Lam.) Husn.] was confirmed in Arkansas in 1995 (Heap Reference Heap2017), glyphosate-resistant goosegrass [Eleusine indica (L.) Gaertn.] (Mueller et al. Reference Mueller, Barnett, Brosnan and Steckel2011) in Tennessee in 2011; glyphosate resistance in barnyardgrass was recently documented in Tennessee and Mississippi (Steckel et al. Reference Steckel, Bond, Montgomery, Phillips and Nandula2017). As a result of glyphosate resistance and the consequent reduced efficacy, many producers have begun to add graminicides to glyphosate applied prior to planting, causing reduced time between application and grass crop planting (Steckel et al. Reference Steckel, Bond, Montgomery, Phillips and Nandula2017). Furthermore, there has been little research to document how precipitation or irrigation could influence the residual activity of ACCase-inhibiting herbicides. Thus, we report on research conducted to determine the residual activity of ACCase-inhibiting herbicides on grass weeds and crops, with and without use of irrigation for activation. We hypothesized that graminicides evaluated would have some residual activity and thus may cause injury to corn, grain sorghum, and rice not resistant to quizalofop planted soon after application.
Materials and Methods
Experiments were conducted in 2014 and 2015 to determine the length of residual activity that could be expected on grass crops and grass weeds following ACCase-inhibiting herbicide application. The field experiment was conducted at the Agricultural Research and Extension Center in Fayetteville, Arkansas (36.4° N 94.9° W) on a Leaf silt-loam soil (fine, mixed, active, thermic Typic Albaquults) with a pH of 5.2 and organic matter content of 1.8%. Experiments were initiated June 13, 2014 and June 18, 2015. The experiment was set up as a split-split plot design, with the whole-plot factor being means of activation (irrigation immediately after application vs rainfall), the split-plot factor being plant-back interval (0, 7, and 14 d after application), and the split-split plot factor being herbicide treatment (six graminicides evaluated at multiple rates). Whole plots (refers to the factor of activation assigned to these plots) had either a 1.3-cm overhead irrigation applied with a traveling gun sprinkler system (Water Reel™, Smith Irrigation Equipment, Kensington, KS) or no irrigation (herbicide activation due to rainfall occurring just after spraying). Irrigation equipment was pre-calibrated with multiple rainfall gauges to ensure that accurate irrigation amounts were achieved.
Conventional rice, quizalofop-resistant rice, grain sorghum, and corn were planted in single rows perpendicular to the treated plots across each of the four replications of the experiment at the aforementioned three intervals. The conventional rice cultivar ‘Roy J’ and an experimental quizalofop-resistant variety (Provisia™ rice, BASF Corp., Research Triangle Park, NC) were planted at a seeding rate of 68 seeds m–1 of row. For grain sorghum, DeKalb™ hybrid DKS53-67 was planted at a seeding rate of 20 seeds m–1 of row, and a Smartstax™ (glyphosate/glufosinate-resistant) corn hybrid ‘Croplan 6274SS’ was planted at a seeding rate of 13 seeds m–1 of row. Herbicides were applied to a tilled, bare soil prior to planting crops using a CO2-pressurized backpack sprayer calibrated to deliver 143 L ha–1 at 276 kPa. Herbicide treatments evaluated are listed in Table 1, with some herbicides applied at two rates. The split-split plot to which herbicides were applied was 1.8 by 7.6 m. The plots were over-sprayed with 2,4-D at 533 g ae ha–1 (Weedar™ herbicide, Nufarm Americas Inc., Alsip, IL) at 2 and 4 wks after initiating the experiment to control broadleaf weeds.
Table 1 Herbicide treatments applied before first planting at Fayetteville, Arkansas.

Stand counts from 1 m of row for each crop were recorded 14 d after planting (DAP). Visual observations were collected for crop injury and weed control on a scale of 0 to 100, with 0 being no injury or weed control and 100 being complete crop death or weed control. Biomass from 1 m of row for all crops and a random 1 m2 for a natural population of broadleaf signalgrass and barnyardgrass were collected at 35 d after each separate planting. Biomass samples were oven-dried at 65 C for 14 d.
All data were analyzed with JMP Pro 12.1 (SAS Institute Inc., Cary, NC) using the Fit Model procedure. For data that met the assumptions for ANOVA, means were separated using Fisher’s protected LSD (α=0.05). Because of differing environmental conditions, years were analyzed independently. Unlike crop response, barnyardgrass and broadleaf signalgrass measurements were analyzed as a split-plot design, because the weed species evaluated were a natural population; thus, there were no multiple plant-back intervals.
Results and Discussion
Overall, significant interactions and main factor effects (i.e., activation, herbicide treatment, and plant-back interval) occurred with year; thus, 2014 and 2015 data were analyzed and are presented independently. Probably, this fact can be attributed to the differing rainfall patterns between years. For 2014, ideal conditions for this experiment occurred, as there was minimal rainfall after initiation of the experiment (Figure 1), with the first appreciable rainfall of 1.2 cm 10 d after treatment (DAT). This rain-free period allowed differentiation between activation treatments through use of irrigation. Thus, the main effect and interactions with activation were generally significant for the parameters evaluated. However, in 2015 a rainfall event began within 24 h of herbicide application, with 10.4 cm of rainfall occurring within 72 h of initiating the experiment (Figure 1), resulting in no difference between activation treatments.

Figure 1 Precipitation 21 d after herbicide treatment for Fayetteville, Arkansas in 2014 and 2015. Experiments were initiated on June 13, 2014 and June 18, 2015.
Crop densities at 14 DAP resulted in no significant herbicide interactions or main effects for either year (data not shown). Although a significant main effect was observed for plant-back interval for multiple crop stand counts both years, within a plant-back interval no differences between treated and nontreated plots were observed either year; thus, differences may be due to conditions that affected germination at planting. Graminicides did not appear to have an effect on stand establishment of any crop evaluated.
All crops exhibited a negative response from residual activity of the evaluated herbicides, except for quizalofop-resistant rice. Quizalofop-resistant rice showed no significant effect from any of the applied herbicides, with no more than 4% injury observed in 2014 and 3% injury in 2015 (data not shown).
Grain Sorghum Response
In 2014, a significant herbicide treatment-by-activation interaction occurred for visible injury and biomass production of grain sorghum. The greatest injury resulted from the higher rate of sethoxydim with irrigation activation (20% injury), which was significantly greater than all other herbicide treatments except the higher rate of fluazifop with irrigation (15%) (Table 2). Greater injury from sethoxydim can most likely be attributed to having lower Kd and Koc compared to other herbicides evaluated (Table 3), which led to greater availability of the herbicide in the soil. Likewise, although fluazifop is tightly bound to the soil, it rapidly degrades to fluazifop-p-acid, which is highly mobile in the soil and probably led to greater injury to grain sorghum (Martens Reference Martens2014). Quizalofop (low and high), clethodim (low), fenoxaprop, fluazifop (high), and sethoxydim (high) resulted in greater injury when activated by sprinkler irrigation compared to the same herbicide without irrigation activation, averaged across plant-back intervals. Without irrigation for activation, injury was much lower; the highest injury was only 7% from multiple treatments, with few differences between treatments. Likewise, biomass for grain sorghum followed a trend similar to that of injury, with the lowest biomass resulting when sethoxydim was applied at a high rate with irrigation activation (85%) (Table 2); however, the sethoxydim (high) treatment with activation was only different from sethoxydim (low) with activation for relative biomass. Relative biomass was significantly reduced for quizalofop (low and high), fluazifop (high), and sethoxydim (high) with herbicide activation compared to nonactivated treatments (Table 2). Plant-back timing did not have a significant effect on either injury or relative biomass.
Table 2 Injury (14 DAP) and biomass (35 DAP) of grain sorghum, corn, and conventional rice as influenced by the residual activity of ACCase-inhibiting herbicides with and without irrigation activation in 2014 at Fayetteville, AR.Footnote a Footnote b
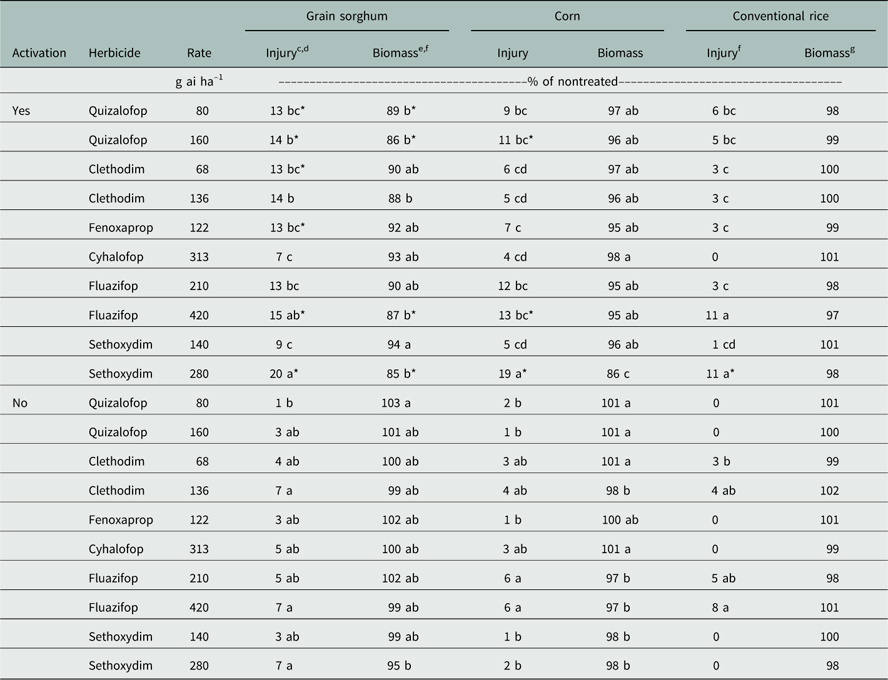
a Abbreviations: ACCase, acetyl-CoA carboxylase; DAP, d after planting.
b Means within a column and activation level followed by the same lowercase letter are not different.
c Injury data expressed as percent relative to the nontreated control.
d Asterisk denotes increased injury with activation compared to no activation within an herbicide treatment.
e Biomass data expressed as percent relative to a nontreated control. Nontreated control resulted in 285, 296, and 38 g m–1 of row oven-dried biomass for grain sorghum, corn, and conventional rice, respectively.
f Treatments averaging 0 were removed from analysis for conventional rice injury due to violating the assumptions of ANOVA (homogeneity of variance).
g Conventional rice biomass resulted in no significant difference between treatments (P>0.05).
Table 3 Adsorption to soil particles (Kd), adsorption to soil organic carbon (Koc), and solubility in water of ACCase-inhibiting herbicides.Footnote a

a Abbreviations: ACCase, acetyl-CoA carboxylase; N/A, not available.
In 2015, with increased rainfall soon after test initiation, grain sorghum injury did not respond to activation treatment. As in 2014, sethoxydim (high) showed the greatest injury of 16% (Table 4); similarly, sethoxydim (high) produced the lowest relative biomass of 92%. Unlike 2014, a significant main effect for plant-back timing occurred in 2015 for relative grain sorghum biomass. At the plant-back timings of 0 and 7 DAT, relative biomass was 96% of the nontreated control averaged across herbicides and activation. However, at 14 DAT, relative biomass increased to 98%, thus showing an overall decrease in residual activity of the herbicides due to plant-back interval (Table 4).
Table 4 Herbicide treatments and plant-back interval effects on injury (14 DAP) and biomass (35 DAP) of grain sorghum, corn, and conventional rice at Fayetteville, AR, in 2015.Footnote a Footnote b
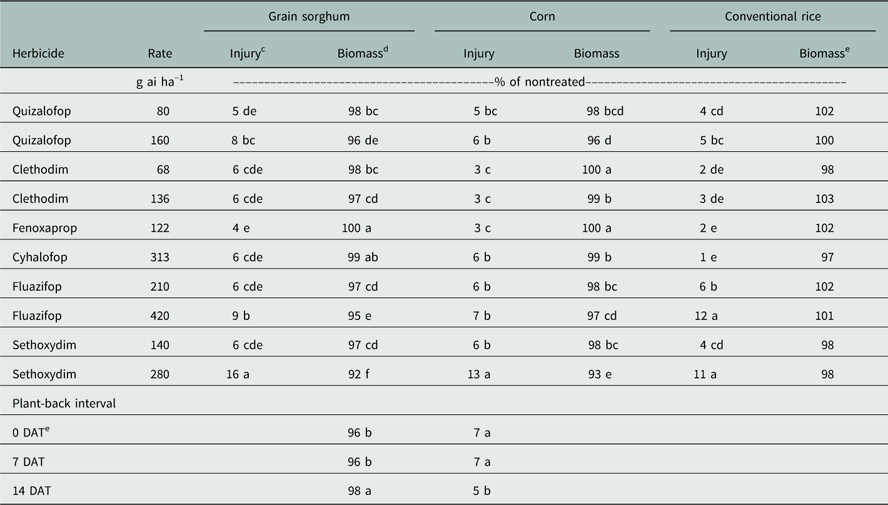
a Abbreviations: DAP, d after planting; DAT, d after treatment.
b Means within a column followed by the same lowercase letter are not different according to Fisher’s protected LSD (α=0.05).
c Injury data expressed as percent relative to the nontreated control.
d Biomass data expressed as percent relative to nontreated control. Nontreated control resulted in 276, 291, and 42 g m–1 of row oven-dried biomass for grain sorghum, corn, and conventional rice, respectively.
e Conventional rice biomass resulted in no interactions or main effects.
Research has shown that even though rainfall or irrigation is sometimes required to activate a herbicide in the soil, excessive rainfall can accelerate degradation of an herbicide, or cause a loss from runoff or leaching. This can reduce the length of residual activity of an herbicide (Heatherly and Hodges Reference Heatherly and Hodges1998; Splittsoesser and Derscheid Reference Splittsoesser and Derscheid1962).
Corn Response
Like grain sorghum, a significant herbicide treatment-by-activation interaction occurred for visible injury and reduced corn biomass in 2014. Greatest injury resulted from sethoxydim (high) with activation of 19% (Table 2), which was higher than any other treatment. Herbicide treatments without activation resulted in much lower injury; the highest injury of any treatment was only 6%. Injury from quizalofop (high), fluazifop (high), and sethoxydim (high) increased when irrigation was applied, over no activation treatments. Corn biomass showed similar results, with sethoxydim (high) with activation having the lowest relative biomass of 86%, which was lower than other treatments (Table 2). Similarly, relative biomass decreased with herbicide activation for quizalofop (high) and sethoxydim (high) compared to treatments without activation.
In 2015, only herbicide treatments and plant-back intervals were significant for corn injury or relative biomass. Similar to 2014, the herbicide sethoxydim (high) produced the greatest visual injury of 13% in 2015, which was greater than any other treatment (Table 4). Sethoxydim (high) also resulted in the lowest relative biomass (93%) of any herbicide. Plant-back timing had a significant effect on corn injury, with the 0 and 7-DAT timings resulting in 7% injury averaged across herbicides and activation, whereas the 14-DAT timing resulted in lower injury at 5%.
Conventional Rice Response
Conventional rice showed results similar to those of grain sorghum and corn, but with generally lower levels of injury. In 2014, conventional rice injury was 11% following fluazifop (high) and sethoxydim (high) with activation (Table 2). Activation treatment only increased the injury of sethoxydim (high) from 0 without to 11% with activation. Little difference was observed between activation treatments for fluazifop (high); visual injury was 8% even without activation. Biomass of conventional rice did not show any significant interactions or main effects. In 2015, herbicide treatment was the only factor that showed significant differences in crop injury for conventional rice. Overall, injury observed in 2015 was very similar to 2014 for those treatments with activation due to the rainfall events in 2015. Fluazifop (high) and sethoxydim (high) resulted in the greatest injury to conventional rice of 12% and 11%, respectively (Table 4).
Grass Weed Control
Control of grass weeds was evaluated both years, with broadleaf signalgrass (15 plants m–2) and barnyardgrass (3 plants m–2) being the predominant grasses in both years. Overall, little residual weed control was observed from any ACCase-inhibiting herbicide evaluated, with only the herbicide treatment being significant for the 14-DAT rating of broadleaf signalgrass (data not shown). Subsequent control rating and relative biomass at 35 DAT did not result in any significant interactions or mains effects for broadleaf signalgrass or barnyardgrass. Because of the low level of residual injury to grass crops evaluated, little residual control of grass weeds was expected from ACCase-inhibiting herbicides.
Practical Implications
The results from this research primarily help determine plant-back intervals for ACCase-inhibiting herbicides to grass crops. The experimental outcome demonstrates that quizalofop-resistant rice is tolerant to PRE applications of ACCase-inhibiting herbicides, the cyclohexanediones and aryloxyphenoxy propionic acids, with the greatest injury reaching only 4%. Thus, quizalofop-resistant rice can be planted immediately following a graminicide application without risk of injury. Injury to conventional rice can occur if planted in close proximity to an ACCase-inhibiting herbicide application but was generally less sensitive than grain sorghum or corn. Caution must be taken with subsequent planting of grain sorghum or corn after an ACCase-inhibiting herbicide application, especially with sethoxydim. No strong impact of plant-back interval (0 to 14 DAT) on grass crop response was apparent for either year, confirming that, although the residual activity was relatively low, many of the herbicides persisted in the soil past 14 d. Timing and amount of rainfall following application of an ACCase herbicide will affect the risk for injury to a subsequent crop or the length of time between application and planting of a grass crop. A rainfall event after herbicide application can increase the residual activity of ACCase herbicides; however, large rainfall events can decrease the persistence of the herbicide in the soil. Particle runoff is the likely cause (Wauchope 1978) because of the generally high adsorption to soil particles (Kd), high adsorption to soil organic carbon (Koc), and low water solubility of most ACCase-inhibiting herbicides (Table 3). In the same sense, increased microbial degradation from greater soil water availability (Parker and Doxtader Reference Parker and Doxtader1983) could reduce residual activity of ACCase-inhibiting herbicides, which are in large part degraded by soil microbes (Shaner et al. 2014). Overall, the ACCase-inhibiting herbicides studied produced little residual grass weed control and thus should only be relied on for POST control.