Introduction
The U.S. Department of Agriculture–Agricultural Research Service (USDA-ARS) has played a fundamental and prominent role in weed science research in the United States and internationally. This review is a synopsis of Weed Science Society of America (WSSA)-sponsored presentations on ARS research. The webinar presentations were recorded and are available at: https://wssa.net/meeting/weed-science-webinar-series. The first section briefly covers the history of ARS weed science research, which is followed by descriptions of recent research in a broad range of weed science categories that include management tactics (integrated weed management [IWM], biological control, and automation), basic mechanisms (competition, invasive plant genetics, and herbicide resistance), and ecosystem impacts (invasive plant spread, climate change, conservation, and restoration). The final section of the review concludes with a discussion of future directions.
Past—ARS Weed Science Research
Beginning in the mid-1970s, ARS weed science research grew rapidly, concomitant with academic and industry weed science research. With the increasing use of herbicides in the 1950s through the 1970s, much of this research was related to herbicides, including a considerable number of studies on the environmental fate of herbicides. Weed science did not become an organized discipline until the age of chemical management of weeds. The early “weed scientists” were trained in botany, plant physiology, and agronomy, but not in weed science. From 1975 until the present, a National Program Leader (NPL) for ARS weed science research projects was part of the program management team in Beltsville, MD (ARS Office of National Programs). Since the 1970s, there have been several NPLs whose primary responsibility has been weed science (Table 1). Additionally, two weed science NPLs have been entomologists, partly due to the overlapping program responsibilities of ARS NPLs.
Table 1. Agricultural Research Service Weed Science National Program Leaders

a Lawrence Christy and Joe Antognini shifted jobs several times between National Program Leader and Research Leader of an ARS unit conducting research on destroying illicit drug crops.
By the mid-1970s, there were ARS weed science projects at about 35 locations across the United States and a few overseas laboratories. The largest group of scientists engaged in weed science research was at the Southern Weed Science Laboratory (SWSL) located in Stoneville, MS. Circa 1980, there were about 20 scientists at SWSL, including visiting scientists, engaged in research on herbicide efficacy; aquatic weed management; biological control of weeds using insects and plant pathogens; IWM; application technology for chemical and bioherbicides; modes of action of herbicides and mechanisms of resistance to herbicides; environmental fate of herbicides; and weed taxonomy, biology, and ecology. This diverse research was conducted by agronomists, plant biologists, entomologists, plant pathologists, soil scientists, chemists, ecologists, taxonomists, and agricultural engineers.
The SWSL was the largest and most diverse weed science laboratory in the world at that time, with only the Weed Research Organization in the United Kingdom being similar in size. Neither exists today, and no laboratory with a similar scope in weed science has taken their place. Other strong ARS weed science groups in 1980 were those in Beltsville, MD (herbicide mode of action and environmental fate, and organic weed management), Fargo, ND (herbicide metabolism), and Davis, CA (aquatic weed management). Many outstanding ARS weed scientists were found at more isolated locations and in smaller programs, which is still true today.
In 2003, a special issue of Pest Management Science highlighting USDA-ARS pest management summarized weed science research on herbicide resistance (Vaughn Reference Vaughn2003), aquatic weed management (Anderson Reference Anderson2003), weed biology (Forcella Reference Forcella2003), allelopathy and natural products for weed management (Duke et al. Reference Duke, Baerson, Dayan, Rimando, Scheffler, Tellez, Wedge, Schrader, Akey, Arthur, De Lucca, Gibson, Harrison, Peterson and Gealy2003), biological control of weeds (Quimby et al. Reference Quimby, DeLoach, Wineriter, Goolsby, Sobhian, Boyette and Abbas2003), and an area-wide pest management project on the invasive species leafy spurge (Euphorbia esula L.) (Anderson et al. Reference Anderson, Prosser, Wendel, Delfosse and Faust2003). Forcella (Reference Forcella2003) summarized the state of ARS weed science research in 2001 and found that 130 scientists in 28 states were identified as working on weed science–related projects. In 2023, there were 35 ARS scientists conducting weed science research in 13 states.
The attrition in weed science research since those days is mirrored at many universities and in industry. Still, ARS has more scientists working on weed management and related science areas than any other U.S. organization (and perhaps the world, based on geographic coverage). ARS weed scientists have helped to shape WSSA, with eight presidents since its inception. Seventeen ARS weed scientists have won the WSSA Outstanding Research Award since 1973, and many are WSSA Fellows. The contribution of ARS weed scientists to our understanding of weed biology and management has been exemplary. For example, an expert in herbicide mode of action was the ARS Deputy Administrator of Crop Production and Protection in the Office of National Programs from the mid-1990s to 2010 and a top plant physiologist in weed science organized and launched the Office of Scientific Quality Review (OSQR) and became the office’s first Scientific Quality Review Officer in 2000. The OSQR evaluates and improves all ARS projects with review panels composed of peer scientists from outside ARS. Two weed scientists have also been elected to the ARS Science Hall of Fame, the highest honor of ARS, which is an agency with almost 2,000 scientists.
Additional ARS accomplishments in weed science include: discovery of several herbicide modes of action (e.g., endothall); development of safer methods for illicit drug plant management in South America; improved weed management recommendations; inoculative biological control insects for numerous invasive plants (e.g., alligator weed [Alternanthera philoxeroides (Mart.) Griseb]); development of improved aquatic weed management methods; discovery of weed biological control microbes; a rapid diagnostic method for glyphosate-resistant weeds; advances in understanding of regulation of dormancy in weedy species and their seeds; understanding of herbicide metabolism and environmental fate of herbicides; the rope wick herbicide applicator; other novel weed management tools; and advances in sustainable weed management. These and many more historical accomplishments of ARS weed scientists have laid the groundwork for present ARS weed science research and propelled the field into the future.
Present—ARS Weed Science Research
Tactics
IWM in Cropping Systems
IWM provides the best chance to control troublesome weeds by utilizing two or more of the following strategies: biological, chemical, cultural, mechanical, and prevention (Harker and O’Donovan Reference Harker and O’Donovan2013). The main goals of IWM in cropping systems are to reduce reliance on a single tactic, incorporate complexity that leads to greater diversity, and provide an advantage to the crop over the weed. Successful IWM is not measured by the efficacy of an individual tactic, but rather the long-term ability to reduce and prevent an increase in weed populations.
Crop production involves biological and social systems that are both complex and dynamic. Developing agronomically relevant, cost-effective, and environmentally sound IWM is a process that requires patience and persistence, as results are often more long term than other approaches.
Two major reasons for utilizing and improving IWM are: (1) weeds have been, and continue to be, a major threat to the crops that feed and support humanity; and (2) chemical control is the primary defense against weeds in U.S. crops. Throughout the latter half of the 20th century, herbicides were developed and widely adopted for controlling weeds in crops (Westwood et al. Reference Westwood, Charudattan, Duke, Fennimore, Marrone, Slaughter, Swanton and Zollinger2018). In the 1970s, herbicide resistance emerged, and today the United States leads the world in the number of documented cases (Heap Reference Heap2023). While multiple types of herbicide resistance continue to develop in weeds, the discovery and development pipeline has stagnated with the corresponding adoption of herbicide-resistant crop technology (Duke and Dayan Reference Duke and Dayan2022). Limited new herbicide commercialization is expected in the foreseeable future for various reasons, including the high cost of bringing new herbicide products to market (Shaw et al. Reference Shaw, Barrett, Schroeder, Asmus, Ervin, Jussaume and Coble2018). Nonetheless, overreliance on limited herbicide chemistries and continued evolution of herbicide resistance are expected unless significant action is taken to avert or mitigate the problem (Haywood et al. Reference Haywood, Vadlamani, Stubbs and Mylne2021; Westwood et al. Reference Westwood, Charudattan, Duke, Fennimore, Marrone, Slaughter, Swanton and Zollinger2018).
With increasing climate variability, weeds are not only getting harder to control with conventional tactics, but also may have greater impact on crop yields. Current crop projections in various greenhouse gas emissions scenarios often do not factor in the future effects of weeds. Recent work by the ARS Global Change and Photosynthesis Research Unit (GCPRU) in Urbana, IL, explored relationships among weather variability, herbicide performance, and crop losses using historic data sets from herbicide evaluations conducted by the University of Illinois over the last three decades. Landau et al. (Reference Landau, Hager, Tranel, Davis, Martin and Williams2021a) found the efficacies of important preemergence herbicides in corn (Zea mays L.) are threatened by more variable weather. Additional research found crop yield losses due to weeds are exacerbated by high temperatures or low rainfall during corn silking (Landau et al. Reference Landau, Hager and Williams2021b) or soybean [Glycine max (L.) Merr.] seed fill (Landau et al. Reference Landau, Hager and Williams2022). Unfortunately, higher temperatures and greater rainfall variability during crop flowering are expected in the future for much of the U.S. Corn Belt (Hayhoe et al. Reference Hayhoe, Wuebbles, Easterling, Fahey, Doherty, Kossin, Sweet, Vose and Wehner2018; Romero-Lankao et al. Reference Romero-Lankao, Smith, Davidson, Diffenbaugh, Kinney, Kirshen, Kovacs and Ruiz2014). Herbicide resistance and deteriorating weed control threaten crop adaptation to climate change, thus underscoring the critical need for innovation in and diversification of weed management.
The GCPRU and researchers in academia have explored several aspects of IWM with a particular interest in making crops more competitive with weeds. For example, robust relationships have been found for sweet corn hybrid competitiveness with weeds in vastly different production regions (Williams et al. Reference Williams, Boydston and Davis2008b). In addition, sweet corn competitive ability has been shown to directly influence herbicide performance, whereby poorly competitive hybrids are at greater risk of weed control failure (Williams et al. Reference Williams, Boydston and Davis2008a). Underlying principal canopy factors describing crop development were found to account for most of the variation in competitive ability among commercial hybrids (So et al. Reference So, Williams, Pataky and Davis2009). Corn morphology and phenology undergo distinct changes across the wide planting window of sweet corn (Williams Reference Williams2008) that influence competitive ability with weeds (Williams Reference Williams2006). Furthermore, multiple biological factors have been elucidated that contribute to weed escapes in sweet corn, including maize dwarf mosaic virus, the most prevalent viral disease of sweet corn (Williams and Pataky Reference Williams and Pataky2012). These facets of crop competitive ability have been exploited by researchers and practitioners, including plant breeders, to improve IWM in not only sweet corn, but other row crops as well.
More recent research on crop competitive ability has explored the role of sweet corn plant density tolerance (PDT). Greater PDT in field corn accounts for much of the yield gain due to genetic improvement over the last half-century (Duvick Reference Duvick2001). The relatively slow yield gains over time and poor competitive ability of sweet corn with weeds, as compared with field corn, were believed to be driven in part by poor PDT. Indeed, researchers at GCPRU found historical changes in PDT since the first introduction of hybrid sweet corn in the 1930s (Dhaliwal et al. Reference Dhaliwal, Ainsworth and Williams2021). While variation in PDT exists among modern processing sweet corn hybrids (Williams Reference Williams2012), most commercial hybrids have average to poor PDT (Williams Reference Williams2015a). Recent on-farm research throughout the Midwest shows hybrids with superior PDT are being underplanted (Dhaliwal and Williams Reference Dhaliwal and Williams2019, Reference Dhaliwal and Williams2020b). Moreover, transcriptional and functional analyses have identified candidate physiological processes involved in sweet corn PDT (Choe et al. Reference Choe, Drnevich and Williams2016, Reference Choe, Ko and Williams2021). While PDT is intraspecific competition (crop vs. crop), not interspecific competition (crop vs. weed), previous research has shown the two are linked (Williams and Boydston Reference Williams and Boydston2013). Tolerance to density and general stress is advantageous when the crop is competing with weed escapes.
IWM in vegetable legumes is also being improved by ARS scientists at the GCPRU. Edamame is a special type of vegetable soybean developed and cultivated for consumption at the immature seed stage. Previously, nearly all edamame available to U.S. consumers was imported from Asian countries, despite the United States being the leading producer of grain-type soybean. Food processors wanted to grow the crop domestically; however, only a single herbicide was registered, and weeds were a major problem. In partnership with the USDA IR-4 Project, universities, herbicide companies, and GCPRU scientists conducted research that facilitated the registration of 12 herbicides from eight sites of action (Williams et al. Reference Williams, Hausman and Moody2017, Reference Williams, Moody and Hausman2019; Williams and Nelson Reference Williams and Nelson2014). Nonchemical tactics also were developed, including the use of competitive cultivars (Williams Reference Williams2015b), exploiting crop seed size for competitiveness (Crawford and Williams Reference Crawford and Williams2018), and using an early-terminated rye (Secale cereale L.) cover crop system (Crawford et al. Reference Crawford, Williams and Wortman2018; Korres et al. Reference Korres, Kitis, Hausman, Moody and Williams2020). Additional agronomic research aimed at improving crop performance included determining appropriate seeding depths (Crawford and Williams Reference Crawford and Williams2019) and identifying economically optimal plant densities for machine-harvested edamame (Dhaliwal and Williams Reference Dhaliwal and Williams2020a). Collectively, certain obstacles to the development of a competitive, sustainable U.S. edamame industry have been removed, including IWM components that have been adopted by the vegetable industry.
The use of IWM in organic systems is challenging, because organic farmers do not have access to cost-effective, systemic herbicides used by conventional farmers, and thus are generally limited to mechanical and cultural methods of weed control. Weed management costs in high-value, high-input organic specialty crops such as vegetables and strawberries in regions such as California can be extremely expensive (i.e., $12,350 to $24,700 or more per hectare per crop). ARS researchers at the Crop Improvement and Protection Research Unit in the Salinas Valley of California developed a simple, highly effective novel hoe with a flexible blade for controlling weeds adjacent to plastic mulch. This hoe has been an important tool to help organic farmers control weeds in cover-cropped strawberry [Fragaria × ananassa (Weston) Duchesne ex Rozier (pro nm.) ssp. cuneifolia (Nutt. ex Howell) Staudt (pro nm.)] furrows without damaging the plastic mulch (Brennan and Smith Reference Brennan and Smith2018). Cover cropping in these strawberry systems is important for reducing soil erosion and runoff, but needs to be done with weed-suppressive cover crops and tools that minimize weed growth and seed production in the furrows (Brennan and Smith Reference Brennan and Smith2018).
Advancements in Weed Biological Control in Non-Crop Systems
Classical biological control has been practiced for more than a century with high safety, but varied success. The Invasive Plant Research Laboratory (IPRL) in Fort Lauderdale, FL, is quantitatively evaluating the benefits of biological control agent impacts on weed populations, such as water hyacinth [Eichhornia crassipes (Mart.) Solms], A. philoxeroides, and Brazilian peppertree (Schinus terebinthifolius Raddi), for invaded plant communities in riparian, wetland, and terrestrial ecosystems (McEvoy et al. Reference McEvoy, Higgs, Coombs, Karaçetin and Starcevich2012), and the occurrence of “indirect effects” of released agents on trophic food webs (Tipping et al. Reference Tipping, Smith, Lake, Minteer, Goode, Foley and Gettys2020). Much work is now underway by the IPRL, along with the Invasive Species and Pollinator Health Research Unit (ISPHRU) in Albany, CA, and the Pest Management Research Unit (PMRU) in Sidney, MT, in several areas, including advances in molecular tools to identify agents and pinpoint host provenance and for other applications; broad ecological examinations, including response to changes in climate; and utilization of novel tools to ask wide-ranging questions.
Shifts in temperature, precipitation, stochastic weather events, and other factors associated with climate change are impacting invasive plants and their associated herbivores (Liu et al. Reference Liu, Yu, Sun and Ding2022; Ward and Masters Reference Ward and Masters2007). Much effort is being devoted by the IPRL, ISPHRU, and PMRU to ensure climatic compatibility between the source and recipient communities, and additional research in the proceeding years will be conducted to ensure that as climate and ranges of weeds shift, the agents will follow (Gurr et al. Reference Gurr, Wratten, Landis and You2017; McEvoy et al. Reference McEvoy, Higgs, Coombs, Karaçetin and Starcevich2012; Reddy et al. Reference Reddy, Pratt, Hopper, Cibils-Stewart, Walsh and Mc Kay2019). Increasingly, in addition to temperature-dependent biology studies, range modeling based on those responses and presence/absence data is also being used to predict how populations will not only expand once released, but might shift in response to changes in temperature (Sánchez-Guillén et al. Reference Sánchez-Guillén, Córdoba-Aguilar, Hansson, Ott and Wellenreuther2016). For example, IPRU scientists demonstrated that the presence of a shared parasitoid had no impact on a native congener, but this example of unrealized indirect effects may shift if plant chemistries change (Tipping et al. Reference Tipping, Smith, Lake, Minteer, Goode, Foley and Gettys2020). Many of these plant–insect–parasitoid interactions are indirect, interacting, and context dependent, emphasizing the need for larger-scale investigations for the impacts of global change drivers on biological control agents and their hosts.
The advent of advanced consumer and professional-level unmanned aerial systems (UAS) has widened the potential for detection of nascent populations of both invasive plants and their newly introduced biological control agents. The IPRL noted that several populations of Floracarus perrepae, a herbivorous mite and biological control agent for the invasive climbing fern [Lygodium microphyllum (Cav.) R. Br.], were discovered only after intensive in-person searching (Lake et al. Reference Lake, Smith, Pratt, Boughton and Pemberton2014). Before these searches, it was assumed that the agent had not dispersed from its initial introduction (AJ Boughton, personal communication). Like many invasive weeds, climbing fern grows in extremely remote and difficult to access areas, and remote detection would be far more efficient. Detecting insect herbivory from UAS equipped with high-resolution cameras is currently a major avenue of research within agroecosystems (Garcia Furuya et al. Reference Garcia Furuya, Ma, Faita Pinheiro, Georges Gomes, Gonçalvez, Junior, de Castro Rodrigues, Blassioli-Moraes, Furtado Michereff, Borges, Alaumann, Ferreira, Osco, Marques Ramos, Li and de Castro Jorge2021; Tetila et al. Reference Tetila, Machado, Astolfi, Belete, de, Amorim, Roel and Pistori2020). The IPRL, ISPHRU, and PMRU are continuing to develop university and industry partnerships to expand the detection capabilities with UAS and provide more information for training artificial intelligence (AI).
New Technology for Weed Identification and Control
Recent advances in computer vision systems that can detect and identify weeds at the species level enable monitoring and precision management applications that are increasing knowledge on weed management, reducing herbicide inputs, and providing more effective control of herbicide-resistant weeds (Beckie et al. Reference Beckie, Ashworth and Flower2019; Christensen et al. Reference Christensen, Dyrmann, Laursen, Jørgensen, Rasmussen, Kerry and Escolà2021). Training and validating the machine learning (ML) algorithms that enable species-level classification of weeds within crop fields requires a large number of annotated images of individual species at multiple growth stages; the lack of suitable data sets is a major barrier to further innovation in this area (Wu et al. Reference Wu, Chen, Zhao, Kang and Ding2021). To overcome this barrier, ARS researchers in the Sustainable Agricultural Systems Laboratory (SASL) in Beltsville, MD, and university collaborators are generating what will eventually be an open-access image repository of all North American weeds. High-resolution imagery has been collected for the most problematic weed species in corn, soybean, and cotton (Gossypium hirsutum L.) crops using both a high-throughput robotic phenotyping platform (a.k.a., Benchbot; Figure 1A) and isolation of target weeds growing naturally in crop fields. The SASL and collaborators have developed both approaches that will allow for both automated segmentation and annotation of the weed imagery (Figure 1B), eliminating the most time-consuming and costly step in image data collection for computer vision applications.
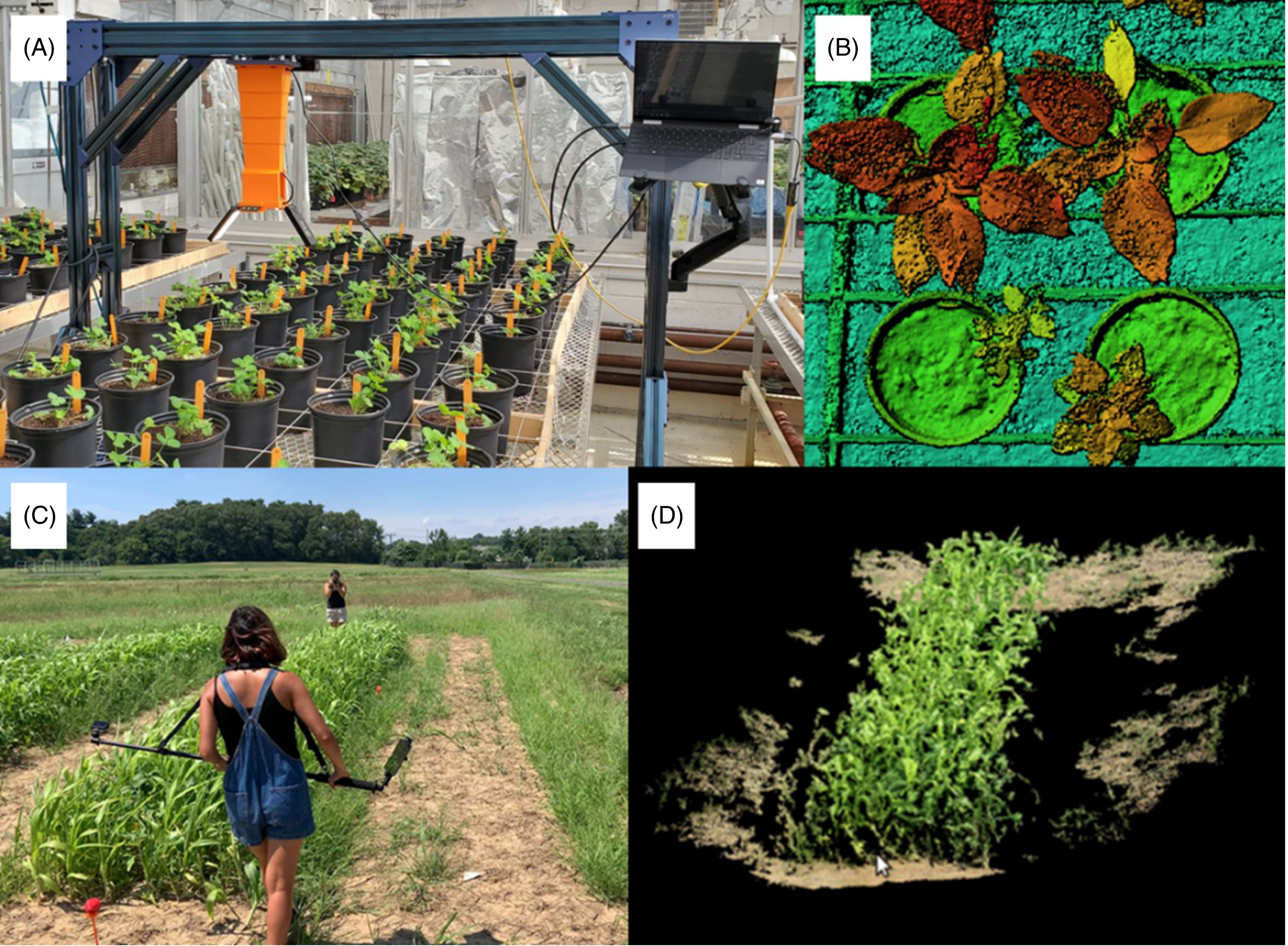
Figure 1. (A) BenchBot autonomous high-throughput imaging system, (B) example imagery from BenchBot after automated segmentation of weeds from background objects, (C) testing of the handheld version of the Weeds3D system at the Beltsville Agricultural Research Center, and (D) example 3D reconstruction of plant biomass from the Weeds3D systems.
The SASL is also leading the development of Weeds3D, a low-cost computer vision platform for weed detection, species identification, and biomass estimation (Figure 1C). Using the structure-from-motion approach (Ramos et al. Reference Ramos, Avandaño and Prieto2018), Weeds3D uses GoPro camera technology, cloud data processing, and a custom Android application to generate 3D point cloud reconstructions of weed and crop biomass (Figure 1D), allowing for high-resolution mapping of weed populations while also keeping the technology affordable and scalable for farmers. More recently, the SASL led in adapting the Weeds3D system to include a low-cost OAK-D (OpenCV AI Kit 3D) camera system that integrates stereo cameras with a single RGB (red, green, blue) camera. This approach is less computationally intensive and enables real-time species identification and depth mapping. Weeds3D will eventually be integrated with smart sprayers, camera-guided cultivation, and other precision weed management technologies and will be used by researchers to monitor the long-term impacts of precision IWM programs.
In addition to ground-based research efforts, the ARS Crop Production Systems Research Unit (CPSRU) in Stoneville, MS, and the ARS Aerial Application Technology Research Unit (AATRU) in College Station, TX, have utilized UAS to capture and assess target weeds with optional application features. The scientists at CPSRU have developed a rapid and nondestructive method combining hyperspectral plant sensing and ML algorithms to differentiate glyphosate-resistant (GR) and glyphosate-sensitive (GS) weeds. Three weed species, Palmer amaranth (Amaranthus palmeri S. Watson), Italian ryegrass [Lolium perenne L. ssp. multiflorum (Lam.) Husnot], and johnsongrass [Sorghum halepense (L.) Pers.] were the focus of studies showing that the technique could differentiate GR from GS plants with a classification accuracy of between 75% and 94% (Huang et al. Reference Huang, Lee, Nandula and Reddy2018, Reference Huang, Zhao, Pan, Reddy and Zhang2022; Reddy et al. Reference Reddy, Huang, Lee, Nandula, Fletcher, Thomson and Zhao2014). The AATRU scientists have shown positional accuracy of current dual-channel real-time kinetics global positioning systems (GPS) for individual weeds to be less than 5 cm. These results are the basis of expanding research at CPSRU to distinguish other GR and GS weed species biotypes and at AATRU to develop a database to precisely guide UAS-based applications depending on meteorological conditions in the field.
Mechanisms
Role of Plant Physiology in Weed–Crop Competition
In weed science, a well-established phenomenon is the critical period for weed control (Nieto et al. Reference Nieto, Brando and Gonzalez1968), which posits that weeds only significantly impact yield when present during a narrow window early in the growing season. Weeds removed before or left after that window have minimal impact on yield. Importantly, the window occurs early in the growing season when weeds are generally too small to shade the crop and resources are rarely limited in well-managed agroecosystems. Yet more resources (e.g., water, nutrients) cannot compensate for weed presence and change the percentage of yield loss between weedy and weed-free conditions (Bandeen and Buchholtz Reference Bandeen and Buchholtz1967; Benaragama and Shirtliffe Reference Benaragama and Shirtliffe2020; Kropff and van Laar Reference Kropff, van Laar, Kropff and van Laar1993; Lindquist et al. Reference Lindquist, Evans, Shapiro and Knezevic2010; Norsworthy and Oliveira Reference Norsworthy and Oliveira2004; Price et al. Reference Price, Korres, Norsworthy and Li2018; Young et al. Reference Young, Wyse and Jones1984). In addition, when weed density is increased, a hyperbolic reduction in crop yield occurs instead of the linear response that might be expected if weeds were reducing yield due only to resource competition (Kropff and Spitters Reference Kropff and Spitters1991; Spitters et al. Reference Spitters, Kropff and De Groot1989).
An alternative hypothesis is that weeds are producing a signal(s) that induces changes in the biochemistry and physiology of the crop by altering growth and development. A team led by scientists at the ARS Sunflower and Plant Biology Research Unit (SPBRU) in Fargo, ND, is conducting research to better understand how blocking the production, transmission, perception, or transduction of weed-generated signals may allow crops to reach full production potentials in the presence of weeds through the early part of the growing season.
Since phytochrome was first discovered by USDA-ARS researchers (Poulos et al. Reference Poulos, Griesbach, Hapeman, Duke and Armbrust2016), many studies have demonstrated that crops can perceive differences in the ratio of red to far-red light (Huber et al. Reference Huber, Nieuwendijk, Pantazopoulou and Pierik2021). Perception of increased far-red light by (crop) plants causes changes in development that reduce yield. For example, plants exposed to high levels of far-red light grow taller with more fragile stems (increase lodging), flower earlier (less accumulation of resources for seed production), and produce fewer leaves (limited uptake of nutrients and water) (Huber et al. Reference Huber, Nieuwendijk, Pantazopoulou and Pierik2021). There have been numerous studies designed to identify gene expression differences in crops and model plants in response to weed pressure (Bowsher et al. Reference Bowsher, Shetty, Anacker, Siefert, Strauss and Friesen2017; Bruggeman et al. Reference Bruggeman, Horvath, Fennell, Gonzalez-Hernandez and Clay2020; Horvath et al. Reference Horvath, Hansen, Moriles-Miller, Pierik, Yan, Clay, Scheffler and Clay2015, Reference Horvath, Bruggeman, Moriles-Miller, Anderson, Dogramaci, Scheffler, Hernandez, Foley and Clay2018, Reference Horvath, Clay, Bruggeman, Anderson, Chao and Yeater2019).
At SPBRU, several genes have been identified that involve light signaling in soybean in response to weed presence (Horvath et al. Reference Horvath, Hansen, Moriles-Miller, Pierik, Yan, Clay, Scheffler and Clay2015). In corn, differences in response to weeds have been observed through the upregulation of a gene called FAR-RED IMPAIRED RESPONSE 1 (Horvath et al. Reference Horvath, Bruggeman, Moriles-Miller, Anderson, Dogramaci, Scheffler, Hernandez, Foley and Clay2018). However, the most interesting response was the induction of biotic defense responses associated with salicylic acid signaling, identified as a protein kinase complex known as TARGET OF RAPAMYCIN (TOR). TOR is responsible for altering the activity of many genes and proteins involved in cell division, nutrient use and transport, and hormonal signals that control plant developmental processes (Burkart and Brandizzi Reference Burkart and Brandizzi2021; Dobrenel et al. Reference Dobrenel, Caldana, Hanson, Robaglia, Vincentz, Veit and Meyer2016; Saxton and Sabatini Reference Saxton and Sabatini2017). The hypothesis is that weeds induce a general stress response that downregulates TOR, an effect that is the primary driver of reduced growth and yield (Horvath et al. Reference Horvath, Clay, Swanton, Anderson and Chao2023).
The SPBRU scientists have identified a small number of genes differentially expressed in corn growing with weeds, regardless of the species present (Horvath et al. Reference Horvath, Clay, Bruggeman, Anderson, Chao and Yeater2019). They isolated the promoter from one of these genes and identified several regions of that promoter that were conserved between corn, sorghum [Sorghum bicolor (L.) Moench], sugarcane (Saccharum officinarum L.), and rice (Oryza sativa L.). These conserved sequences are most likely to be involved in the regulation of this weed-inducible gene. When the promoter of the weed-inducible gene was connected to two different genes (a reporter gene known as RED3, and a bacterial gene called NahG that can degrade salicylic acid), increased expression of both genes occurred in the presence of weeds. Unfortunately, at least in preliminary experiments, increasing transcription of the NahG gene did not significantly improve the growth of corn in the presence of weeds. More work is needed to confirm these observations. Regardless, the identification of a weed-inducible promoter will allow future work on induction of other genes that might give corn and related crops increased ability to tolerate or repress nearby weeds. A weed-inducible promoter could also be used to drive expression of genes that produce phenotypes such as bioluminescence that are easily identified by aerial imagery from UAS, which could alert growers to weed infestations so they could be controlled before becoming problematic.
Molecular Basis for Controlling Invasive Plants
Innovative molecular-based solutions are being developed to advance invasive plant science and improve control. Combining molecular advancements with classical biological control can result in genetically tailored invasive plant management strategies that exploit plant defense systems with simultaneous exposure to microbial-based biological control agents. The Foreign Disease-Weed Science Research Unit (FDWSRU) in Frederick, MD, along with university and federal partners, is investigating diverse molecular approaches to improve and augment microbial-based biological control agents, including encapsulation technologies and complementary genetic biological control tools.
The FDWSRU is leading collaborative microbial encapsulation research that uses cutting-edge material science technology and value-added encapsulation chemistries (i.e., inorganic, organic, and hybrid materials). The goal is to assist in improving biopesticide efficacy by enhancing pathogen survival, infectivity, and slow-release mechanisms across harsh, unpredictable climatic conditions. Novel microbial encapsulation strategies can 1) produce prolonged release profiles responsive to environmental stimuli, 2) protect against environmental stress degradation, and 3) have improved foliar uptake and persistence, thereby increasing rates of weed suppression and pathogen establishment. New technological advances and lower manufacturing costs are allowing encapsulation platforms to be developed for and tailored to a wide variety of microorganisms and applications to targeted invasive plants, thus improving the efficacy and reliability of microbial biopesticides. The FDWSRU is currently investigating encapsulation delivery platforms that support the survival of and infection with a pathogenic bacterium recently characterized on the invasive plant garlic mustard [Alliaria petiolata (M. Bieb.) Cavara & Grande] as a bacterial model system (Tancos et al. Reference Tancos, Dubrow, Carpenter and Bogdanove2022).
Genetic biological control tools, such as ribonucleic acid interference (RNAi)-based applications or gene drives, have the potential to revolutionize invasive plant management strategies (Figure 2). RNAi is an evolutionarily conserved biological defense system that provides protection against endogenous and exogenous pathogenic and parasitic double-stranded RNA (dsRNA), including viral intermediates, transgenes, and transposons (Vaucheret et al. Reference Vaucheret, Beclin, Elmayan, Feuerbach, Godon, Morel, Mourrain, Palauqui and Vernhettes1998; Wilson and Doudna Reference Wilson and Doudna2013). Once dsRNA is detected, the cell’s biological defense system is activated, and dsRNA is cleaved into short 21- to 24-nucleotide RNA fragments (siRNA) by Dicer-like endonucleases (Wilson and Doudna Reference Wilson and Doudna2013). The siRNA fragments are subsequently used to target homologous messenger RNA (mRNA) for degradation. Degradation and suppression of targeted mRNA results in translational inhibition and transient gene silencing (Alder et al. Reference Alder, Dames, Gaudet and Mango2003; Wilson and Doudna Reference Wilson and Doudna2013). Foliar applications of exogenous dsRNA have been demonstrated to effectively inhibit viral infections and protect against fungal pathogens (Faustinelli et al. Reference Faustinelli, Power and Arias2018; McLoughlin et al. Reference McLoughlin, Wytinck, Walker, Girard, Rashid, De Kievit, Fernando, Whyard and Belmonte2018; Mitter et al. Reference Mitter, Worrall, Robinson, Li, Jain, Taochy, Fletcher, Carroll, Lu and Xu2017; Worrall et al. Reference Worrall, Bravo-Cazar, Nilon, Fletcher, Robinson, Carr and Mitter2019). The FDWSRU is investigating the efficacy, specificity, and deliverability of topically applied dsRNA in model plant systems such as A. petiolata. Exogenous applications of both dsRNA and endoribonuclease-prepared small interfering RNA (esiRNA) targeting phytoene desaturase of Nicotiana benthamiana Domin., a widely used experimental host in plant virology, resulted in photobleached phenotypes compared with negative controls (MA Tancos, unpublished data).

Figure 2. (A) Depiction of a bipartite synthetic Clustered Regularly Interspaced Short Palindromic Repeats (CRISPR) cassette (red) consisting of a Cas9 endonuclease and a guide ribonucleic acid RNA (gRNA) that is flanked by homology arms (HA) (black). (B) Following expression of the CRISPR cassette, gRNA binds to Cas9 and directs the complex to a unique sequence-specific site for DNA cleavage and homology-directed repair (HDR). Following HDR, the CRISPR cassette is copied into both genomic regions. (C) Standard Mendelian inheritance results in 50% of progeny inheriting a modified gene. In contrast, a gene drive would bias inheritance, theoretically resulting in all progenies (∼99%) inheriting the modified gene, thereby “driving” the modified gene into an invasive weed population.
Addressing Herbicide Resistance with Alternative Chemistries
Certain weed taxa are significantly more prone to herbicide resistance than others, although relatively few studies to date have quantitatively assessed the relationship between particular weed characteristics and the propensity for evolved resistance. Amaranthus palmeri has emerged as one of the most noxious and economically significant weed species, and U.S. biotypes have been identified with confirmed resistance to acetolactate synthase inhibitors, dinitroanilines, glyphosate, hydroxyphenylpyruvate dioxygenase inhibitors, and triazine herbicides (e.g., Nandula et al. Reference Nandula, Reddy, Koger, Rimando, Duke, Bond and Ribeiro2017). Scientists in the CPSRU in Stoneville, MS, along with university colleagues, discovered a novel genetic mechanism involving extra-chromosomal circular DNAs (eccDNAs) in A. palmeri, which causes a dramatic increase in the copy number of the gene encoding 5-enolpyruvylshikimate-3-phosphate synthase (EPSPS)—the target of the herbicide glyphosate. In highly resistant A. palmeri populations, EPSPS genes are amplified 40- to 100-fold and become distributed throughout the genome (Molin et al. Reference Molin, Yaguchi, Blenner and Saski2020). The increased number of functional EPSPS genes enables resistant individuals to maintain sufficient enzyme activity following glyphosate exposure, thus circumventing the inhibitory effects of the herbicide. eccDNAs are not unique to plants and have also been discovered in normal and cancerous human cells and several other eukaryotic organisms, although their precise biological role is not well understood at present (Molin et al. Reference Molin, Yaguchi, Blenner and Saski2020).
In addition to understanding the molecular basis for resistance mechanisms in weeds, like A. palmeri, researchers must identify new herbicide chemistries that feature novel modes of action that can be used in IWM approaches. Nature has produced a wealth of specialized phytotoxic metabolites that could potentially be developed or directly utilized for this purpose, and this vast resource is largely untapped. This potential is underscored by the fact that a significant percentage of the existing synthetic compounds used for crop protection were actually inspired by natural products, despite their fairly modest presence overall within the global pest control market (Sparks et al. Reference Sparks, Sparks and Duke2023). The Natural Product Utilization Research Unit (NPURU) in Oxford, MS, is focused on pursuing natural products as new herbicides that can target multiple cellular processes, are typically more environmentally benign, generally possess shorter environmental half-lives, pose lower risks to non-target organisms, and tend to be more favorably perceived by the public and are likely to face fewer regulatory hurdles for commercialization (Duke et al. Reference Duke, Rimando, Baerson, Scheffler, Ota and Belz2002; Marrone Reference Marrone2019).
The NPURU is actively investigating spliceostatin C, an analogue of spliceostatin A (SSA) which was previously identified as an anti-tumor compound and potent inhibitor of mammalian pre-mRNA splicing (Roybal and Jurica Reference Roybal and Jurica2010). In collaboration with a commercial company, NPURU scientists determined that spliceostatin C is a highly potent phytotoxin and exhibits activity comparable to commercially available herbicides (Marrone Reference Marrone2019), with a mode of action similar to that of SSA (Bajsa-Hirschel et al. Reference Bajsa-Hirschel, Pan, Padney, Asolkar, Chittiboyina, Boddy, Machingura and Duke2023). Importantly, spliceostatin C is also highly active against glyphosate-resistant Amaranthus weed species. Effective control of A. palmeri with spliceostatin C was achieved at approximately 500 mg ai ha−1.
A second natural product evaluated by NPURU scientists is the monoterpene aldehyde citral, a major component of swingle oil (Citrus aurantiifolia Christm.). Citral is phytotoxic to a number of plant species and is used commercially for weed management in organic farming. Citral is composed of a mixture of the geometric isomers Z-3,7-dimethyl-2,6-octadien-1-al (neral) and E-3,7-dimethyl-2,6-octadien-1-al (geranial), with commercial citral typically containing 60% geranial and 40% neral. While the phytotoxic effects of citral are well documented, no specific mode of action had previously been assigned to this herbicide. Recently, NPURU scientists determined that the mode of action of citral likely involves the inhibition of single-stranded DNA-binding proteins required for transcription.
Additional natural product–based herbicide leads were identified by NPURU scientists working with the medicinal plant Ammi visnaga (L.) Lam., known as toothpickweed or khella, a herb belonging to the Umbelliferae family. Prior work examining the allelopathic potential of A. visnaga reported phytotoxicity against legumes, maize, and several weeds commonly associated with wheat (Triticum aestivum L.) cultivation. Scientists from NPURU and a small company found two furanochromones, khellin and visnagin, to possess herbicidal activity, and mode of action studies are now being conducted. Both khellin and visnagin show promise as natural product herbicides and/or lead molecules for the design of novel synthetic herbicides, and a patent was also recently awarded for this use (Sosa et al. Reference Sosa, Travaini, Walter, Cantrell, Duke, Carrillo, Ceccarelli and Ortega2021).
Microorganisms also produce phytotoxic compounds that could potentially be isolated and developed into new herbicides, or alternatively, the living microbes could themselves be used directly as weed biological control agents. One promising ARS-led study at the Biocontrol of Pests Research Unit (BPRU) in Stoneville, MS, demonstrated that the bioherbicidal pathogenic fungus Myrothecium verrucaria (Alb. and Schwein.) Ditmar:Fr. (strain IMI368023) (MV)can control both glyphosate-resistant and glyphosate-susceptible A. palmeri seedlings (Hoagland and Boyette Reference Hoagland and Boyette2018). Myrothecium verrucaria has bioherbicidal activity on several weed species and genera, including kudzu [Pueraria lobata var. montana (Lour.) Merr.], purslanes (Portulaca spp.), spurges (Euphorbia spp.), morningglory (Ipomoea spp.), hemp sesbania [Sesbania herbacea (Mill.) McVaugh], and A. palmeri (Duke et al. Reference Duke, Pan, Bajsa-Hirschel and Boyette2022; Hoagland and Boyette Reference Hoagland and Boyette2016). Additionally, important synergistic interactions of some herbicides and MV for control of certain weeds were discovered at BPRU (Boyette et al. Reference Boyette, Hoagland, Weaver and Reddy2008, Reference Boyette, Hoagland and Stetina2014a, Reference Boyette, Hoagland, Weaver and Stetina2014b).
Many plant species naturally produce highly potent phytotoxins referred to as allelochemicals, which serve as agents in a type of chemical warfare occurring between plants competing for limited environmental resources (Inderjit and Duke Reference Inderjit2003; Weston and Duke Reference Weston and Duke2003). Allelopathic interactions have been proposed to have profound effects on the evolution of plant communities through the loss of susceptible species via chemical interference and by imposing selective pressure favoring individuals resistant to inhibition from a given allelochemical (e.g., Schulz and Wieland Reference Schulz and Wieland1999). The allelochemical sorgoleone, which is exclusively produced in root hairs of Sorghum spp., is currently being developed as a plant-incorporated protectant (transgenically imparted like Bt toxin) herbicide by ARS scientists at NPURU (Figure 3). The NPURU investigators have to date identified all of the genes encoding enzymes required for the biosynthesis of sorgoleone (Baerson et al. Reference Baerson, Dayan, Rimando, Nanayakkara, Liu, Schroder, Fishbein, Pan, Kagan, Pratt, Cordonnier-Pratt and Duke2008; Cook et al. Reference Cook, Rimando, Clemente, Schröder, Dayan, Nanayakkara, Pan, Noonan, Fishbein, Abe, Duke and Baerson2010; Pan et al. Reference Pan, Bajsa-Hirschel, Vaughn, Rimando, Baerson and Duke2021). These efforts represent an extremely important advancement for the plant-incorporated protectant field and could be the first example of a plant-incorporated protectant herbicide.
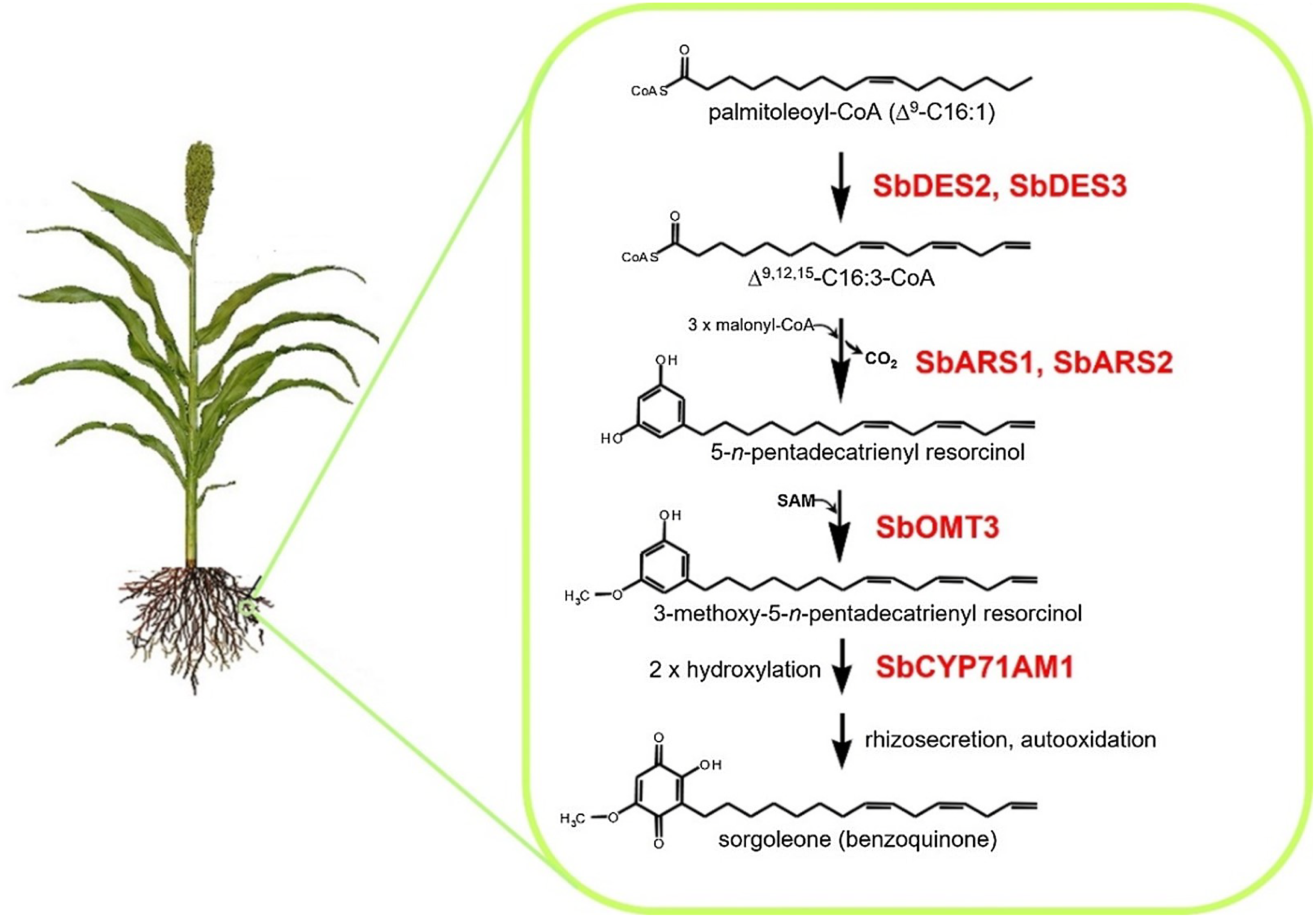
Figure 3. Sorgoleone has a potential as a plant-incorporated protectant herbicide. The highly bioactive benzoquinone allelochemical sorgoleone is produced exclusively within root hair cells of members of the genus Sorghum. Genes of the entire sorgoleone pathway are being used to transform crops to produce sorgoloeone (Pan et al. Reference Pan, Bajsa-Hirschel, Vaughn, Rimando, Baerson and Duke2021).
Impacts
Spread and Distribution of Invasive Plants
Invasive plant spatial spread and distribution are driven by the impacts of abiotic filters such as climate, soils, barriers, and disturbance on population dynamics, dispersal, and biotic interactions. In addition, genetic constitution and evolutionary changes following introduction contribute to spread and distribution in an invader’s novel range. Scientists at the ISPHRU in Albany, CA, and the PMRU in Sidney, MT, are conducting research to elucidate these drivers and modulators of invasion. Understanding the underlying factors driving spread and distribution can improve weed risk assessments and inform management strategies (Davies and Sheley Reference Davies and Sheley2007).
Ultimately, the spread and distribution of invasive plants are the result of plant demography and dispersal capabilities. Linking ecological drivers of propagule pressure to recruitment dynamics and biotic interactions can improve management efficacy and better predict costs. For instance, seed transport associated with flooding patterns might augment propagule pressure and shorten timelines for Russian olive (Elaeagnus angustifolia L.) stand increase (West et al. Reference West, Reinhold, Poole and Espeland2020). Rand et al. (Reference Rand, West, Russell and Louda2020) demonstrated seed predation by a biological control agent reduces already limited seed availability, further reducing populations of a non-target thistle (Cirsium canescens Nutt.), even when recruitment pressures differ across sites. These studies highlight the importance of considering how seed availability varies across time and space. Variation across sites influencing the importance of seeds versus clonal recruitment to an invasive plant’s persistence can also affect management decisions and biological control strategies. Long-term control requires targeting weeds at vulnerable life stages, and these targets often vary across space and time. Understanding how weed populations vary in the landscape and feed back to affect the efficacy of management tools is vital to sustainable, low-cost, low-input invasive plant control.
Abiotic environmental filters interact with the biotic tolerances of invasive plants to determine their spread and distribution. The ISPHRU conducted a biogeographic survey to assess the impact of yellow flag iris (Iris pseudacorus L.) on plant communities along estuarine gradients in its native (Andalusia, Spain) and invaded (California, USA) ranges (Gallego-Tévar et al. Reference Gallego-Tévar, Grewell, Whitcraft, Futrell, Bárcenas-Moreno and Castillo2022). Iris pseudacorus presence greatly reduced species richness and diversity in the naturalized range independent of variation in soil salinity, contrasting with its occurrence in highly diverse plant communities in the home range. Further, PMRU scientists discovered that I. pseudacorus disperses mostly by seed (Gaskin et al. Reference Gaskin, Pokorny and Mangold2016). Germination of seed-dispersing weeds is driven by their environmental tolerances or niche breadth. The ISPHRU scientists empirically assessed the germination ecology of I. pseudacorus seeds, documenting high germination and establishment capacity under increasing temperatures. Experimental results and a thermal time model revealed highest germination under diurnally fluctuating temperatures and high germination capacity under a wide range of environmental conditions (Gillard et al. Reference Gillard, Castillo, Futrell and Grewell2021, Reference Gillard, Castillo, Mesgaran, Futrell and Grewell2022). Results support weed risk assessment and suggest the need to prioritize management early in the season before seed production to prevent further spread of I. pseudacorus.
Disturbances such as fire and grazing influence invasive plant spread and distribution by differentially impacting natives and invasives, thereby impacting interspecific competition. The PMRU scientists and university collaborators found that the impact can be direct, such as differential fire-induced bud mortality among species, or indirect, such as altering microhabitat or trophic feedbacks (Hiers et al. Reference Hiers, Treadwell, Dickinson, Kavanagh, Lodge, Starns, Tolleson, Twidwell, Wonkka and Rogers2021). Disturbances such as fire and grazing alter soil chemistry and biology (McGranahan et al. Reference McGranahan, Wonkka, Dangi, Spiess and Geaumont2022), potentially either disrupting or enhancing plant soil feedbacks that drive plant invasions. The impacts of disturbance depend on the dominant ecosystem processes in the region and the responses of natives and invasives. The PMRU scientists and colleagues have shown that while wildfire can promote cheatgrass (Bromus tectorum L.) establishment in the Great Basin, historical wildfire is not associated with annual brome invasion in the Great Plains or shrubland-grassland ecotone between the two systems (Porensky and Blumenthal Reference Porensky and Blumenthal2016). Further, they assert that disturbance regime plays a role in determining invasive plant spread, as can be seen with fire severity and spatial pattern (Donovan et al. Reference Donovan, Roberts, Wonkka, Uden, Angeler, Allen, Wedin, Drijber and Twidwell2021).
Plant invasions are rarely homogenous entities. The PMRU scientists have documented key processes such as selection, drift, gene flow, and founding events that can rapidly shape the genetic diversity and spatial population structure of an invasion (Gaskin et al. Reference Gaskin, Bon, Cock, Cristofaro, Biase, De Clerck-Floate, Ellison, Hinz, Hufbauer, Julien and Sforza2011). Also, mode of reproduction can vary widely within an invasion, and this can shape how invasions spread, especially when there is subspecific variation in tolerance and resistance to management practices. The PMRU scientists used molecular markers and flow cytometry to determine that the flowering rush (Butomus umbellatus L.) invasion in western North America is fundamentally different in genotypes, origins, and ploidy from an earlier invasion in eastern North America (Gaskin et al. Reference Gaskin, Andreas, Grewell, Haefliger and Harms2021a). The different genotypes show variation in susceptibility to foliar fungal pathogens (Harms et al. Reference Harms, Williams and Purcell2021). Further, the PMRU scientists used genetic tools to determine the distribution of common mullein (Verbascum thapsus L.) invasion in western North America (Gaskin et al. Reference Gaskin, Endriss, Fettig, Hufbauer, Norton and Sforza2021b). Despite this species facultatively outcrossing, the scientists found the invasion dominated by a single genotype, and 50% of the populations were monotypic. The identification of the most common and diverse invasive genotypes of V. thapsus allows their use in tests of management tools and further studies of mechanisms of this invasion. The genetic information also allowed the PMRU scientists to determine Eurasian origins, which can enable fine-tuned searches for natural enemies.
Climate Change Effects on Weeds and Invasive Plants
Given the array of climatic and atmospheric changes occurring, combined with the tremendous diversity of weeds and invasive plants, some species are likely to perform better under future climatic conditions, while others will perform less well (Liu et al. Reference Liu, Oduor, Zhang, Manea, Tooth, Leishman, Xu and Van Kleunen2017; Sorte et al. Reference Sorte, Ibanez, Blumenthal, Molinari, Miller, Grosholz, Diez, D’Antonio, Olden, Jones and Dukes2013; Vilà et al. Reference Vilà, Beaury, Blumenthal, Bradley, Early, Laginhas, Trillo, Dukes, Sorte and Ibáñez2021). Plant species will move as climates change and will challenge land managers with new weed and invasive plant problems (Bradley et al. Reference Bradley, Blumenthal, Wilcove and Ziska2010). Against this backdrop of variation, scientists at the Adaptive Cropping Systems Laboratory (ACSL) in Beltsville, MD, the ISPHRU in Albany, CA, and the Rangeland Resources and Systems Research Unit (RRSRU) in Fort Collins, CO, have worked to discern general patterns that may be of use to managers and policy makers, such as the vulnerability of different ecosystems to weeds and invasive plants, the types of weeds and invasive plants that are likely to be most problematic in the future, and the efficacy of chemical and biological control (Figure 4).

Figure 4. Agricultural Research Service researchers have focused on understanding how climate change influences weeds/invasive plants and their impacts and management. Image shows a study of how precipitation change influences cheatgrass (Bromus tectorum) invasion in rangelands of northeast Wyoming, USA. (Credit: Anna Kuhne)
The ongoing increase in atmospheric carbon directly stimulates plant growth, including the seed yield of a number of agronomic weeds (Patterson Reference Patterson1995; Ziska Reference Ziska2003; Ziska et al. Reference Ziska, Blumenthal, Runion, Hunt and Diaz-Soltero2011). To appreciate the implications of rising CO2 with respect to weed biology, it is necessary to understand the nature of individual versus group responses; as CO2 increases, both individual species’ responses and interspecific interactions change. Differential responses of weeds and crops, especially in early growth and seed development, can strongly influence weed impacts and crop production. These impacts have been the subject of numerous studies at the ACSL. For example, recent and projected changes in CO2 were found to increase the competitive ability of red (weedy) rice (Oryza punctata Kotzchy ex Steud.), thereby reducing the relative yield of cultivated rice (Ziska et al. Reference Ziska, Tomecek and Gealy2010). Rising CO2 may further contribute to the competitive advantage of O. punctata by increasing seed shattering and seedbank persistence (Balbinot et al. Reference Balbinot, da Rosa Feijó, Fipke, Gehrke, Agostinetto, Kruse, Ziska, Camargo and de Avila2022). In a recent meta-analysis of how climate change influences weed–crop interactions, the presence of weeds negated any benefits of elevated CO2 for crop production (Vilà et al. Reference Vilà, Beaury, Blumenthal, Bradley, Early, Laginhas, Trillo, Dukes, Sorte and Ibáñez2021). Across experimental studies, ACSL scientists found weeds to have similar effects on crops with and without warming or drought (Vilà et al. Reference Vilà, Beaury, Blumenthal, Bradley, Early, Laginhas, Trillo, Dukes, Sorte and Ibáñez2021). In contrast, analysis of decades of herbicide evaluation trials by scientists at the GCPRU in Urbana, IL, showed that very high temperatures or low rainfall can increase yield losses in both corn and soybean (Landau et al. Reference Landau, Hager and Williams2021b, Reference Landau, Hager and Williams2022).
Rangelands differ from cropping systems in that dominant native plants are often slow-growing, stress-tolerant species (Blumenthal et al. Reference Blumenthal, Mueller, Kray, Ocheltree, Augustine and Wilcox2020). Global changes that increase resource availability (elevated CO2, increased precipitation) can therefore put natives at a disadvantage relative to faster-growing invasive species, while changes that reduce resource availability (warming, decreased precipitation) can have the opposite effect (Bradley et al. Reference Bradley, Blumenthal, Wilcove and Ziska2010; Liu et al. Reference Liu, Oduor, Zhang, Manea, Tooth, Leishman, Xu and Van Kleunen2017). The RRSRU scientists have focused on climate change effects on invasion of mixed-grass prairie. Results have shown that several predicted changes favor invasive annual forbs and grasses relative to native plants (Blumenthal et al. Reference Blumenthal, Chimner, Welker and Morgan2008, Reference Blumenthal, Resco, Morgan, Williams, LeCain, Hardy, Pendall and Bladyka2013, Reference Blumenthal, Kray, Ortmans, Ziska and Pendall2016).
Climate change effects on invasive plants may also exacerbate their environmental impacts. The RRSRU scientists found that elevated CO2 greatly increased diffuse knapweed (Centaurea diffusa Lam.) biomass in disturbed mixed-grass prairie, leading to corresponding decreases in plant diversity (Blumenthal et al. Reference Blumenthal, Carrillo, Kray, Parsons, Morgan and Pendall2022). In western rangeland, B. tectorum can reduce fire return times from decades to less than 5 yr, with concomitant decreases in ecosystem diversity and the establishment of B. tectorum monocultures (Fusco et al. Reference Fusco, Finn, Balch, Nagy and Bradley2019). The RRSRU scientists have conducted research on B. tectorum demonstrating that warming can increase B. tectorum growth, while elevated CO2 can increase its flammability, suggesting the potential for intensified effects on rangeland fires (Blank et al. Reference Blank, White and Ziska2006; Blumenthal et al. Reference Blumenthal, Kray, Ortmans, Ziska and Pendall2016).
Climate change is also likely to interact with biological control (Reeves Reference Reeves2017), an interaction being studied by scientists at the ISPHRU. Gall-forming insects are often susceptible to plant water-deficit stress imposed by drought (Dhileepan Reference Dhileepan2004; Harris and Shorthouse Reference Harris and Shorthouse1996). A shoot tip-galling fly (Parafreutreta regalis Munro) was released for biological control of an invasive vine, Cape-ivy (Delairea odorata Lem.), in riparian, forest, and scrubland habitats in California. In greenhouse tests, P. regalis produced 52% fewer galls and 60% fewer adult progeny per gall on water deficit–stressed versus unstressed plants, and the life cycle required 10 additional d (Portman et al. Reference Portman, Santa Cruz and Moran2021). Development time of the wasp Tetramesa romana (another shoot tip-galler) was 5 to 7 d longer on water-stressed giant reed (Arundo donax L.) (Moran Reference Moran2015). This group has also observed reductions in Diorhabda spp. leaf-feeding beetle populations on Tamarisk spp. with spring freezes and flooding, suggesting that increases in extreme weather may also reduce the efficacy of biological control (Knutson et al. Reference Knutson, Tracy, Ritzi, Moran, Royer and Deloach2019).
Alternatively, climate change can on occasion improve biological control efficacy. The RRSRU scientists found that in mixed-grass prairie, elevated CO2 greatly increased growth and seed production of C. diffusa, but also increased seed consumption by the biological control agent Larinus minutus Hochhut (Reeves et al. Reference Reeves, Blumenthal, Kray and Derner2015). This weevil, introduced in 1991 to control multiple Centaurea spp. (Kashefi and Sobhian Reference Kashefi and Sobhian1998), colonized the experimental plots from the surrounding prairie. Increased seed consumption appeared to be caused by earlier C. diffusa flowering with elevated CO2, leading to a better phenological match with L. minutus. Detailed knowledge of the field biology and ecology of biological control agents, beyond those typically derived from quarantine studies required to obtain release permits, will be necessary to predict the effects of climate change on biological control agents (Reeves Reference Reeves2017).
Restoration and Invasive Plants
Restoration has become a major part of invasive plant management on rangelands, simply because killing or removal of invasive plants can open niches for reinvasion. With persistent seedbanks and relative growth rates 7 to 10 times higher than natives, invasive plants are able to establish very quickly. Removing invasive plants is an important part of sustainable management, but in cases where there are inadequate numbers of desirable plants to quickly reoccupy the site, restoration is required.
Just as physics provides the scientific principles for engineering, ecology provides the scientific principles for invasive plant management (Radosevich et al. Reference Radosevich, Holt and Ghersa1997). It is widely understood that species richness and diversity within a plant community improve the ecological function by promoting ecological processes that are central to the health of the system (Chapin et al. Reference Chapin, Zavaleta, Eviner, Naylor, Vitousek, Reynolds, Hooper, Lavorel, Sala, Hobbie, Mack and Diaz2000). More recent research also shows that species richness and diversity are critical for maintaining invasion resistance. The scientists at the Range and Meadow Forage Management Research Unit (RMFMRU) in Burns, OR, created a sophisticated series of plots representing various levels of species richness and diversity in a system that allowed, using Spitters’ (Reference Spitters1983) methodology, the calculation of the degree of niche partitioning among the invader and desirable species (Sheley and Carpinelli Reference Sheley and Carpinelli2005). As species richness and diversity increased, the density of spotted knapweed [Centaurea stoebe L. ssp. micranthos (Gugler) Hayek] decreased. This clearly shows that highly rich and diverse systems are more resistant to invasion because more niches are filled with desired species. The RMFMRU scientists and colleagues developed methods for assessing which plant functional groups appear most critical to invasion resistance based on traits of invasive weeds (Pokorny et al. Reference Pokorny, Sheley, Zabinski, Engel, Svejcar and Borkowski2005; Sheley and James Reference Sheley and James2010).
An ecologically based invasive plant management (EBIPM) framework has been developed by the RMFMRU scientists to be used as a guide for invasive plant management (Sheley et al. Reference Sheley, Mangold and Anderson2006, Reference Sheley, James, Smith and Vasquez2010). The framework provides states with ecological principles as predictors of the direction of the transition based on how the imposed management influences ecological processes. The overview is valuable for moving invasive plant management from misapplied treatments attacking symptoms to management programs that address the underlying cause of invasion, retrogression, and succession (Bard et al. Reference Bard, Sheley and Jacobsen2003).
Successful restoration requires an understanding of ecological mechanisms, processes, and conditions that favor desired species establishment and growth over invasive plants. The EBIPM has helped managers make decisions about how best to manage the ecological processes with a focus on invasive plants (Sheley et al. Reference Sheley, James, Smith and Vasquez2010). The five-step process leads managers through a series of steps that use ecosystem assessment information to identify the “causes” of successional dynamics in the system. Based on research by scientists at RMFMRU and elsewhere, the link has been made from causes to those mechanisms, processes, and conditions that need to be repaired to foster a trajectory toward desirable plant communities. The EBIPM guide shows how tools are used to repair ecological processes and mechanisms, rather than simply employing tactics in an approach more suitable for agricultural systems that have massive disturbances and complete community replacement. In practice, EBIPM can improve restoration by around 10% to nearly a 70% chance of success (Sheley et al. Reference Sheley, Mangold and Anderson2006).
Over the past couple of decades, ecologists and managers have been increasingly focused on managing for a desired plant community, rather than removing undesirable plant species. Complex interrelationships among various components within ecosystems create multiple indirect responses to vegetation management that are very difficult to predict. This creates a strong need to manage invasive plants within the context of the entire ecosystem (Seastedt et al. Reference Seastedt, Hobbs and Suding2008). Invasive plant management must become more integrated within a holistic and systems approach that facilitates problem solving and the attainment of goals rather than practice-based outcomes. Management must assess the complex interrelationship among ecosystem components and processes and design management strategies that influence the underlying ecological cause of invasion and dominance by invaders with predictable outcomes.
Future—ARS Weed Science Research
With the rich history of collaborative weed science research at ARS and its acknowledged contributions to the field, the obvious question is: “Where do we go from here?” The vision for the future of weed science at ARS is based on the past successes as well as initiatives both internal and external to the agency that contribute to the mission of delivering scientific solutions to national and global agricultural challenges.
The vision for ARS weed science is a high-level look at where research might go, considering many of the current and future challenges, such as slowing the spread of invasive plants in natural areas, overcoming weed resistance in cropping systems, and developing new and enhancing existing tools for sustainable management. The challenges in weed science are national and global in scope and negatively impact agroecosystems and natural areas. A vision for sustainable weed and invasive plant management includes three key areas: weed genomics, plant ecology, and application technology.
-
Weed genomics includes the topics of genome sequencing, adaptation/evolution, and epigenetics/gene editing. The direction for research would be to address: (1) the adaptation of stress response mechanisms employed by weeds for crop improvement, (2) the functioning of weed genomes in comparison to those of model species, and (3) the broad stress tolerance of weeds and invasive plants that have very little genetic variation (Sharma et al. Reference Sharma, Barney, Westwood and Haak2021). ARS weed genomics research would be strengthened in these areas by aligning with current efforts, such as the International Weed Genomics Consortium, which is co-led by academia and industry, and the USDA’s Breeding Insights initiative, which provides breeding software and technologies to enrich and speed up breeding pipelines.
-
Plant ecology includes the topics of phenology/morphology, plant competition, and seedling emergence alongside knowledge from many other related fields, such as soils, hydrology, herbivory, and microbiology. The agricultural knowledge gaps specific to weeds are the use of competitive crops and cultivars, alteration of planting density and spatial arrangement, summer fallow periods, weed seed predation, flooding, and biocontrol (Birthisel et al. Reference Birthisel, Clements and Gallandt2021). In natural areas, the research focus is often restoration and the development of management tools that recognize and leverage intraspecific variation and local adaptation (Baughman et al. Reference Baughman, Kulpa and Sheley2022). ARS initiatives on organic agriculture, climate change, and the USDA-wide working lands programs, such as the Regional Conservation Partnership Program, Environmental Quality Incentives Program, and Agricultural Conservation Easement Program, provide direct support for the development of farmer and producer practices that include weed ecology.
-
Application technology for agroecosystems and natural areas includes specific machines, such as sensors, cameras, computers, robotics, and UAS, and the applications of remote sensing, GPS, and other data-gathering/assessing platforms for AI/ML. The research on technology for weeds and invasive plants would bring together different disciplines, including weed biology, agronomy, computer science, engineering, and socioeconomics. Along with research on the technical details, human dimensions of application technology cannot be underestimated or overlooked in relation to adoption and implementation (Young et al. Reference Young, Pitla, Van Evert, Schueller and Pierce2017). A recent WSSA survey of members revealed that precision application technology was one of the highest-ranking topics related to weed control (Brainard et al. Reference Brainard, Haramoto, Leon, Kells, Van Wychen, Devkota, Jugulam and Barney2023). The National AI Initiative, which spans the entire federal government, seeks to accelerate AI research and application, and the National Science Foundation’s Harnessing the Data Revolution, which is enabling new modes of data-driven discovery to address fundamental questions at the frontiers of science and engineering, are two efforts in support of developments related to technology and big data in weed science.
A conceptual framework has been developed for ARS weed science showing the linkages of weed genomics, plant ecology, and application technology (Figure 5). The contribution to this framework by stakeholders and organizations, companies and industry, and academia and research institutions is a crucial aspect of ARS weed science, and many are strong research partners, which is a hallmark of the agency. With key areas and contributors known and identified, the potential topics to be addressed can be individual weed species, a weed population with certain characteristics or functions, and entire agricultural or natural ecosystems. With increasing scale and/or specificity, the research questions posed can take on increasing complexity; finding solutions will be challenging and require more diversified expertise.
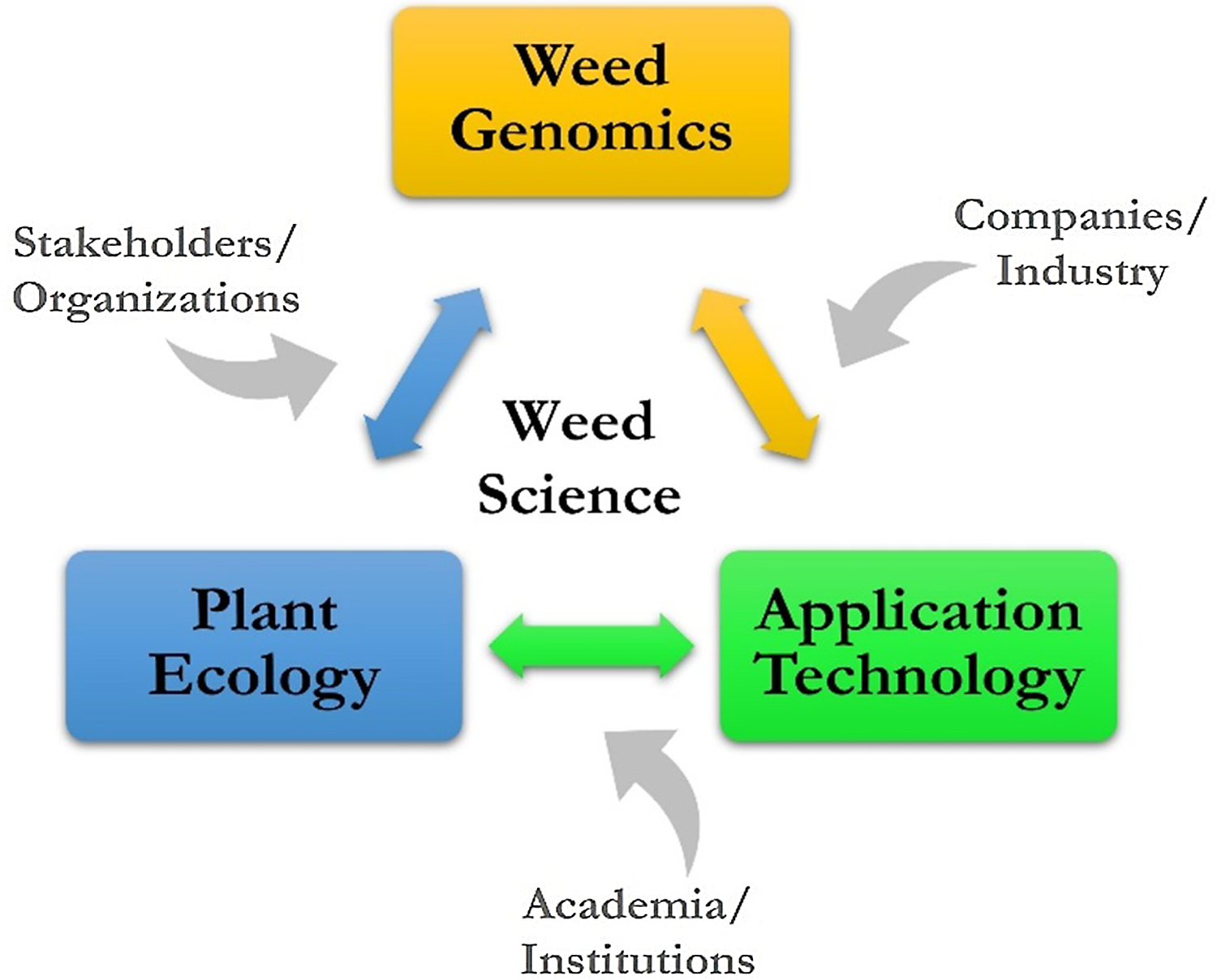
Figure 5. A conceptual framework providing context for addressing challenges for managing weeds in agroecosystems and natural areas.
The establishment of the framework that outlines the future of ARS weed science and being able to address any number of questions and external factors. For example, economics could be connected to application technology. Similarly, the human dimension aspect of acceptance or adoption possibly connects with research on plant ecology. The connections that could be made between external factors and key research areas depending on location, interest, and need are endless.
The current geographic distribution of weed scientists across ARS is broad and could expand and increase in the future. Regardless of whether ARS scientists are addressing weeds in agroecosystems or invasive plants in natural areas or both, they are advancing research in weed genomics, plant ecology, and application technology, and this will need to continue more than ever before through existing and new initiatives. By establishing and strengthening collaborations with public and private partners at local, state, national, and international levels, ARS weed science will be able to address the challenges surrounding invasive plant spread and development of weed resistance and harness new and better utilization of existing tools that will ultimately lead to sustainable weed management.
Acknowledgments
This paper is derived from talks given for this symposium with other USDA-ARS researchers. Mention of trade names or commercial products in this publication is solely for the purpose of providing specific information and does not imply recommendation or endorsement by the United States Department of Agriculture (USDA). USDA is an equal opportunity employer and provider. No conflicts of interest have been declared. The writing of this paper was partially funded by the U.S. Department of Agriculture Cooperative Agreement 58-6060-6-015 grant to the University of Mississippi.