INTRODUCTION
Radiocarbon (14C) datasets have been used in archaeology not only to build regional chronologies but also to study population dynamics, settlement changes, and the correlations between changes in environments and culture (e.g., Anderson et al. Reference Anderson, Goodyear, Kennett and West2011; Crema et al. Reference Crema, Habu, Kobayashi and Madella2016). A large number of 14C dates are readily available in site reports, leading to an increase of radiocarbon studies in Korean archaeology since the 2010s. Popular topics include subsistence changes (Bae et al. Reference Bae, Bae and Kim2013), temporal variabilities of hunter-gatherer sedentism (Ahn et al. Reference Ahn, Kim and Hwang2015), settlement changes during state formations (Park et al. Reference Park, Wright and Kim2017), and population dynamics (Oh et al. Reference Oh, Conte, Kang, Kim and Hwang2017).
In Korea, a considerable number of shell dates is associated with the shell middens dating to the Neolithic period (ca. 8000–3500 cal BP). Over 600 shell middens have been documented in Korea, a large percentage (46%) belonging to the Neolithic period (Ha Reference Ha2010), and some of well-stratified shell middens have provided key data on establishing the regional chronology. Although marine shells from these Neolithic shell middens are frequently dated materials, counting 120 or more, their 14C dates are often underutilized or used without proper calibration accounting for the marine reservoir effect (MRE). The MRE contributes to the disparity between the 14C ages obtained from terrestrial and marine samples. Several factors contribute to this gap, especially upwelling, which occurs when an “older” carbon upwells from the deep ocean and is taken in by marine organisms (Soares and Martins Reference Soares and Martins2010). Recently, Heaton et al. (Reference Heaton, Köhler, Butzin, Bard, Reimer, Austin, Bronk Ramsey, Grootes, Hughen, Kromer, Reimer, Adkins, Burke, Cook, Olsen and Skinner2020) presented Marine20, an update to the internationally agreed marine radiocarbon age calibration curve. Marine20 models the global influence of the MRE by simulating the ocean/atmosphere/biosphere box-model of the global carbon cycle while also integrating the ice core data on the observed changes in CO2. The regional MRE, however, can deviate from the global model due to the variations in the fluvial influx and ocean upwelling (Smittenberg et al. Reference Smittenberg, Hopmans, Schouten, Hayes, Eglinton and Damsté2004; Cook et al. Reference Cook, Ascough, Bonsall, Hamilton, Russell, Sayle, Scott and Bownes2015). By offsetting these impacts, the regional correction value (ΔR) can adjust the global pattern of the MRE and thus be used to calibrate the radiocarbon age of marine organisms correctly.
Utilizing marine shell dates in Korea has been difficult in part due to the lack of ΔR values. Although several values are available from the surrounding regions (Kuzmin et al. Reference Kuzmin, Burr and Jull2001; Southon et al. Reference Southon, Kashgarian, Fontugne, Metivier and Yim2002; Shishikura et al. Reference Shishikura, Echigo and Kaneda2007; Yoneda et al. Reference Yoneda, Uno, Shibata, Suzuki, Kumamoto, Yoshida, Sasaki, Suzuki and Kawahata2007; Hirabayashi et al. Reference Hirabayashi, Yokoyama, Suzuki, Miyairi and Aze2017), only two ΔR values are available in Korea (Kong and Lee Reference Kong and Lee2005) (Table 1). The provenience of shells used in Kong and Lee’s (Reference Kong and Lee2005) study, however, is only known as the south coast without further details. As ΔR values can be highly variable in coastal regions (Kuzmin et al. Reference Kuzmin, Burr and Jull2001; Butler et al. Reference Butler, Scourse, Richardson, Wanamaker, Bryant and Bennell2009; Thornalley et al. Reference Thornalley, McCave and Elderfield2011; Napolitano et al. Reference Napolitano, Fitzpatrick, Clark and Stone2019), previously published values from surrounding regions may not be applicable to the Korean Peninsula. Without additional applicable ΔR values, marine shell dates have a limited value contributing to the Neolithic chronology. Therefore, the chronology is often built exclusively upon pottery typology and 14C dates on charcoal. The Bibongri shell midden site (35°24′38″N, 128°38′44″E) is one of such cases in southeastern Korea (Figure 1). As a multi-component site (7800–2800 cal BP), Bibongri provides a wealth of information on subsistence and material culture throughout the Neolithic periods with well-preserved organic remains (Supplementary Material 1) (Gimhae National Museum 2008, 2012). By adding ΔR values and 14C dates, several studies in eastern Eurasia reassessed the regional settlement patterns and the time depth of the sites, including the Neolithic Boisman 2 site in Primorye (Jull et al. Reference Jull, Kuzmin, Lutaenko, Orlova, Popov, Rakov and Sulerzhitsky1994; Kuzmin et al. Reference Kuzmin, Orlova, Sulerzhitsky and Jull1994, Reference Kuzmin, Richards and Yoneda2002).
Table 1 ΔR values within 823 km proximity to the study area, calibrated based on Marine20.

* Calculated by subtracting 14C age of marine sample and atmospheric 14C age using the calibration curve used in the publication.
** ΔR values cited from original publications, based on Marine98 calibration (Stuiver et al. Reference Stuiver, Reimer, Bard, Beck, Burr, Hughen, Kromer, McCormac, van der Plicht and Spurk1998); Marine04 (Hughen et al. Reference Hughen, Baillie, Bard, Warren Beck, Bertrand, Blackwell, Buck, Burr, Cutler, Damon, Edwards, Fairbanks, Friedrich, Guilderson, Kromer, McCormac, Manning, Bronk Ramsey, Reimer, Reimer, Remmele, Southon, Stuiver, Talamo, Taylor, Van der Plicht and Weyhenmeyer2004); and Marine13 (Reimer et al. Reference Reimer, Bard, Bayliss, Beck, Blackwell, Ramsey, Buck, Cheng, Edwards, Friedrich, Grootes, Guilderson, Haflidason, Hajdas, Hatté, Heaton, Hoffmann, Hogg, Hughen, Kaiser, Kromer, Manning, Niu, Reimer, Richards, Scott, Southon, Staff, Turney and van der Plicht2013).
*** ΔR values based on Marine20 (Heaton et al. Reference Heaton, Köhler, Butzin, Bard, Reimer, Austin, Bronk Ramsey, Grootes, Hughen, Kromer, Reimer, Adkins, Burke, Cook, Olsen and Skinner2020), cited from the 14CHRONO Marine20 Reservoir Database website (http://calib.org/marine; accessed 2021 Mar 16). Calculated by subtracting 14C age of marine sample and atmospheric 14C age in IntCal20 (Reimer et al. Reference Reimer, Austin, Bard, Bayliss, Blackwell, Bronk Ramsey, Butzin, Cheng, Edwards, Friedrich, Grootes, Guilderson, Hajdas, Heaton, Hogg, Hughen, Kromer, Manning, Muscheler, Palmer, Pearson, van der Plicht, Reimer, Richards, Scott, Southon, Turney, Wacker, Adolphi, Büntgen, Capano, Fahrni, Fogtmann-Schulz, Friedrich, Köhler, Kudsk, Miyake, Olsen, Reinig, Sakamoto, Sookdeo and Talamo2020).
**** Uncertainty value (1σ) not presented in the original publication, thus calculated by the authors of this study. References cited: a) Kong and Lee Reference Kong and Lee2005, b) Southon et al. Reference Southon, Kashgarian, Fontugne, Metivier and Yim2002, c) Hirabayashi et al. Reference Hirabayashi, Yokoyama, Suzuki, Miyairi and Aze2017, d) Yoneda et al. Reference Yoneda, Uno, Shibata, Suzuki, Kumamoto, Yoshida, Sasaki, Suzuki and Kawahata2007.
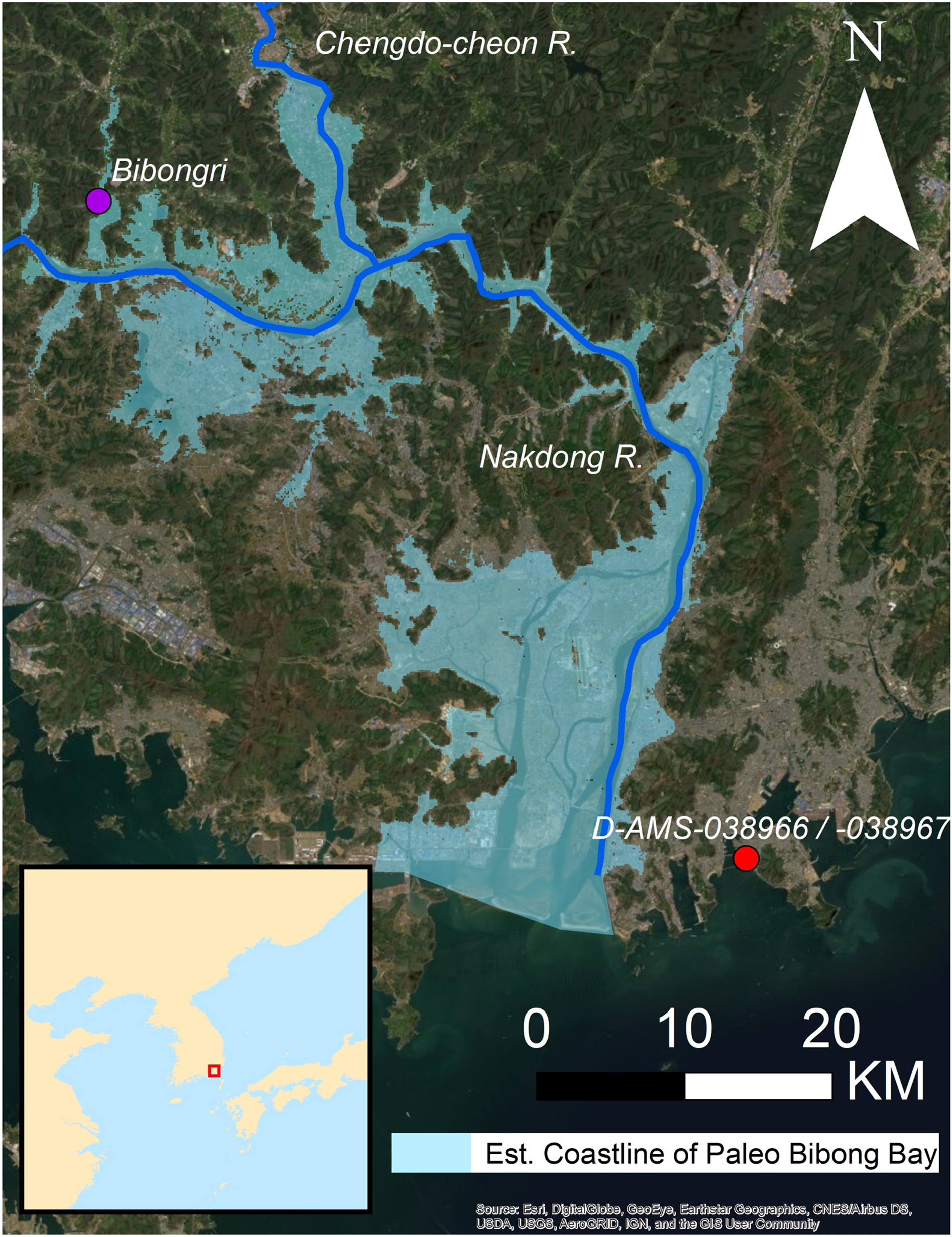
Figure 1 The provenience of pre-bomb shell sample and Bibongri archaeological site, and the estimated coastline of Paleo Bibong Bay, which is in a lighter shade than the river channel (modeled after the Gimhae National Museum 2012: 4).
Our study reports two new ΔR values. We calculate the weighted mean based on these new ΔR values and those published in Kong and Lee (Reference Kong and Lee2005) and apply to the calibration of archaeological shells from Bibiongri. Among several cultural layers identified at Bibongri, Shell Layer 1 is the thickest strata, revealing abundant artifacts and organic remains. By comparing the calibrated marine shell dates to those on charcoal and bone fragments from Shell Layer 1, we aim to address the time depth of this cultural layer.
METHODS
Specimen Used
We obtained one pre-bomb blue mussel (Mytilus edulis) specimen, live-collected at the Busan Bay of South Gyeongnam Province, South Korea on May 29, 1938 (Table 2; Figure 1). The bivalve specimen was preserved in an 80% aqueous solution of ethanol in an air-tight container at the Fisheries Science Museum located in Busan, South Korea.
Table 2 Two 14C dates from a pre-bomb shell specimen and their ΔR values.

* The δ13C values in this table were calculated using isotope ratio mass spectrometry (IRMS) at the Stable Isotope Laboratory, University of Oregon.
** Calculated by subtracting 14C age of marine sample and atmospheric 14C age in IntCal20 (Reimer et al. Reference Reimer, Austin, Bard, Bayliss, Blackwell, Bronk Ramsey, Butzin, Cheng, Edwards, Friedrich, Grootes, Guilderson, Hajdas, Heaton, Hogg, Hughen, Kromer, Manning, Muscheler, Palmer, Pearson, van der Plicht, Reimer, Richards, Scott, Southon, Turney, Wacker, Adolphi, Büntgen, Capano, Fahrni, Fogtmann-Schulz, Friedrich, Köhler, Kudsk, Miyake, Olsen, Reinig, Sakamoto, Sookdeo and Talamo2020).
Blue mussels inhabit rocky, low, intertidal shores, sheltered harbors, open coasts with hard substrates, or any place with dense masses suitable for their body attachment (Al-Dabbas et al. Reference Al-Dabbas, Hubbard and McManus1984; Hong Reference Hong2006; Lee et al. Reference Lee, Mok, Son, Oh, Kim, Lee, Lee and Kim2006). This marine mollusk is widely used for measuring local pollution levels because of its immobile living habit that results in an exclusive intake of nutrients from the local seawater. Its lifespan varies from three to 24 years, depending on the habitat environment (Seed Reference Seed1969; Thiesen Reference Thiesen1973). Most specimens curated at the Fisheries Science Museum between 1905 to 1943 were collected from the harbor of Busan, including the blue mussel specimen used in this study.
Shell Preparation
We cleansed the specimen through soaking and gently brushing with deionized water. The exterior organic layers (periostracum) were etched using 10% hydrochloric acid solution and air-dried for 36 hr to remove foreign substances and diagenetically altered carbonate. The shell was microscopically inspected for an intact terminal edge. Axial locations of the terminal growth margin were carefully sampled by hand with 0.05 mm carbide tip and drill bit at the University of Oregon Island and Coastal Archaeology Laboratory (Figure 2). Two sets of particles were sampled from the same specimen (NFRDI-MS-IS-0000078), constituting the two AMS submissions (D-AMS-038966 and D-AMS-038967) (Table 2). The radiocarbon date measurement was processed with the NEC Pelletron 500 kV AMS device at the DirectAMS (D-AMS) facility (see Zoppi Reference Zoppi2010).

Figure 2 Pre-bomb shell specimen (Mytilus edulis, D-AMS-038966 and -038967) used in this study. Arrows show spots sampled for analysis.
Calculation of ΔR and the Weighted Mean ΔR
We followed the standard procedure of calculating ΔR, used by Kong and Lee (Reference Kong and Lee2005) and other studies (Phelan Reference Phelan1999; Yoshida et al. Reference Yoshida, Tatsuaki, Kunikita, Miyazaki, Sasaki, Yoneda and Matsuzaki2010; Nakanishi et al. Reference Nakanishi, Takemura, Matsuyama, Shimoyama, Hong and Okuno2017; Panich et al. Reference Panich, Schneider and Engel2018). First, we selected a marine shell specimen from the pre-bomb (pre-1950) era to avoid the “bomb effect,” which increased the global atmospheric 14C levels (Nydal Reference Nydal1968; Levin et al. Reference Levin, Kromer, Schoch-Fischer, Bruns, Münnich, Berdau, Vogel and Münnich1985).




where

Equations (1)–(4) discuss the method of deriving ΔR (Jull et al. Reference Jull, Burr and Hodgins2013). ΔR is the difference between the reservoir age of marine sample and that of the global ocean age model, the Marine20 calibration curve in this study (Heaton et al. Reference Heaton, Köhler, Butzin, Bard, Reimer, Austin, Bronk Ramsey, Grootes, Hughen, Kromer, Reimer, Adkins, Burke, Cook, Olsen and Skinner2020). We used the Calib website (QUB 2021a) for the calculation of ΔR. Then we calculated a weighted mean to combine multiple ΔRs into a single value while placing a more weight on the value with a lower error range. The weighted mean is derived by using the equation on the Calib website (see Bevington Reference Bevington1969, QUB 2021b). We apply the weighted mean ΔR to the archaeological shell samples from the Bibongri Site for the 14C age calibration. The calibration was made with OxCal 4.3.2 (Bronk Ramsey Reference Bronk Ramsey2009, Reference Bronk Ramsey2017; Bronk Ramsey and Lee Reference Bronk Ramsey and Lee2013), based on IntCal20 atmospheric curve and Marine20 marine curve (Heaton et al. Reference Heaton, Köhler, Butzin, Bard, Reimer, Austin, Bronk Ramsey, Grootes, Hughen, Kromer, Reimer, Adkins, Burke, Cook, Olsen and Skinner2020; Reimer et al. Reference Reimer, Austin, Bard, Bayliss, Blackwell, Bronk Ramsey, Butzin, Cheng, Edwards, Friedrich, Grootes, Guilderson, Hajdas, Heaton, Hogg, Hughen, Kromer, Manning, Muscheler, Palmer, Pearson, van der Plicht, Reimer, Richards, Scott, Southon, Turney, Wacker, Adolphi, Büntgen, Capano, Fahrni, Fogtmann-Schulz, Friedrich, Köhler, Kudsk, Miyake, Olsen, Reinig, Sakamoto, Sookdeo and Talamo2020).
Bibongri Site
Bounded by steep hills (400 m asl) to the north and west, Bibongri is located at the bottom of the foothills, overlooking an alluvial plain (3.8 m asl) along the Chengdo-cheon river, a tributary of the Nakdong River (Figure 3). Although Bibongri is located inland today, it sat on a widened bay called Paleo Bibong throughout the Neolithic period and thus Neolithic inhabitants faced an inner bay environment (Gimhae National Museum 2008; Williams et al. Reference Williams, Dellapenna and Lee2013). Bibongri’s coastal landscape is well documented by several studies. Sea-level and sedimentological studies exhibit that human activities at Neolithic Bibongri were largely affected by seawater dynamics (Hwang Reference Hwang2008, Reference Hwang2012; Hwang et al. Reference Hwang, Kim and Yoon2013). Over 90% of diatoms identified from Shell Layer 1 belong to marine species, including Coscinodiscus sp., Nitzschia cocconieformis, and Nitzschia granulata (Hwang Reference Hwang2008, Reference Hwang2012). Marine shell species, including pacific oyster (Magallana gigas) and blood cockle (Tegillarca granosa), are also abundant in Shell Layer 1 (Gimhae National Museum 2008; Kaneko Reference Kaneko2008). After the site was abandoned, Bibongri transformed into a low-lying wetland along with sea-level regression and inundation from the Cheongdo-cheon River. As a result, organic materials were well preserved in multiple layers of silty mud, shell, and alluvial sediments.

Figure 3 (a) An aerial view of the Bibongri site, showing Shell Layer 1’s surface, and (b) a schematic stratigraphic profile (Gimhae National Museum 2008: 14, 193). Stratigraphic layer numbers outside the brackets are copied from the Gimhae National Museum (2008), and numbers inside the brackets are copied from the Gimhae National Museum (2012).
Bibongri’s high pH level of six shell layers preserved faunal remains in excellent condition, including at least 35 vertebrate and molluscan taxa. Two common species of mollusks are blood cockle (Tegillarca granosa) and pacific oyster (Magallana gigas) (Kaneko Reference Kaneko2008; Hwang et al. Reference Hwang, Kim and Yoon2013). Dybowski’s sika deer (Cervus nippon hortulorum) and Korean wild boar (Sus scrofa coreanus) are the most common mammalian taxa, similar to other Neolithic shell midden sites in Korea (Lee Reference Lee, Habu, Lape and Olsen2017). Other mammals, fish, and birds were also identified, including dog (Canis lupus familiaris), brown bear (Ursus arctos), raccoon dog (Nyctereutes procyonoides), Mongolian wolf (Canis lupus chanco), Siberian tiger (Panthera tigris tigris), wild water buffalo (Bubalus arnee), Korean ring-necked pheasant (Phasianus colchicus karpowi), duck (Anatidae sp.), redlip mullet (Planiliza haematocheilus), and spotted sea bass (Lateolabrax maculatus) (Kaneko Reference Kaneko2008). Artifacts made of organic materials are also preserved intact, including pine dugout canoe and a paddle, basketry, and wooden and bone tools (Gimhae National Museum 2008, 2012).
An earlier study shows domesticated millets became dietary sources by the Middle Neolithic period (ca. 5500–5000 cal BP) (Crawford and Lee Reference Crawford and Lee2003). More recent studies based on charred remains and grain impressions on pottery confirm the presence of both foxtail and broomcorn millets in the Early Neolithic context at Tongsamdong (Obata Reference Obata2013) and Bibongri (Lee et al. Reference Lee, Cho and Obata2019; Kwak et al. Reference Kwak, Obata and Lee2020). Both charred remains and Early Neolithic pottery with millet impressions from Shell Layer 1 at Bibongri push the introduction of millet to the Early Neolithic period (7700–5500 cal BP) (Lee et al. Reference Lee, Cho and Obata2019; Kwak et al. Reference Kwak, Obata and Lee2020). Floated sediments also yielded diverse edible or medicinal plant taxa, including acorn (Quercus cf. serrata, Q. cf. glauca), wild walnut (Juglans sp.), apricot (Prunus armeniaca or P. mandshurica), wild cherry (P. tomentosa), wild grape (Vitis sp.), spicewood (Lindera sp.), hop (Humulus sp.), dogwood (Cornus sp.), and aster (Asteraceae) (Lee Reference Lee2008, 2017). Overall, the periods when these shell layers accumulated represent a warmer span with seasonally abundant resources (Gimhae National Museum 2008).
Shell Layer 1 is the most thoroughly analyzed stratum at Bibongri since it is the thickest cultural layer and filled with artifacts and organic remains (Figure 3). Shell Layer 1 yielded ten 14C dates on charcoal, bone, and shells published in Gimhae National Museum (2008, 2012), and we added one more 14C date on shell (D-AMS-039265) (Table 3).
Table 3 14C dates from Shell Layer 1 at the Bibongri site.

* The δ13C values in this table were calculated using accelerator mass spectrometry (AMS), not isotope ratio mass spectrometry (IRMS).
** Based on two ΔR values derived from one pre-bomb shell sample in this study.
*** Based on four ΔR values derived from one pre-bomb shell sample in this study and two pre-bomb shell samples from Kong and Lee (Reference Kong and Lee2005).
**** This study used OxCal 4.3.2 for 14C calibration (Bronk Ramsey Reference Bronk Ramsey2009, Reference Bronk Ramsey2017; Bronk Ramsey and Lee Reference Bronk Ramsey and Lee2013). Charcoal and animal bone samples were calibrated using the IntCal20 atmospheric curve, and shell samples were calibrated using the Marine20 marine curve (Heaton et al. Reference Heaton, Köhler, Butzin, Bard, Reimer, Austin, Bronk Ramsey, Grootes, Hughen, Kromer, Reimer, Adkins, Burke, Cook, Olsen and Skinner2020; Reimer et al. Reference Reimer, Austin, Bard, Bayliss, Blackwell, Bronk Ramsey, Butzin, Cheng, Edwards, Friedrich, Grootes, Guilderson, Hajdas, Heaton, Hogg, Hughen, Kromer, Manning, Muscheler, Palmer, Pearson, van der Plicht, Reimer, Richards, Scott, Southon, Turney, Wacker, Adolphi, Büntgen, Capano, Fahrni, Fogtmann-Schulz, Friedrich, Köhler, Kudsk, Miyake, Olsen, Reinig, Sakamoto, Sookdeo and Talamo2020). Lab codes are listed in the at the Radiocarbon journal website (Radiocarbon 2021).
RESULTS AND DISCUSSION
Our two new ΔR values are considerably different from those reported by Kong and Lee (Reference Kong and Lee2005). However, they are generally within the ranges reported in the coastal regions of East Asia (Tables 1 and 2). Moderate variations in the ΔR values in close proximity are not uncommon (Yoshida et al. Reference Yoshida, Tatsuaki, Kunikita, Miyazaki, Sasaki, Yoneda and Matsuzaki2010; Panich et al. Reference Panich, Schneider and Engel2018). Several factors may have caused this difference, including handling error during the pre-laboratory and laboratory processes (Kim et al. Reference Kim, Wright, Lee, Lee, Choi, Kim, Ahn, Choi, Seong, Hyun, Hwang, Yang and Yang2016), an inherent variability in the measurement condition of each sample (Scott et al. Reference Scott, Gordon and Naysmith2007), and a possibility of exposure to the freshwater runoff depending on the geomorphology of the sample collection location (Ascough et al. Reference Ascough, Cook and Dugmore2005).
ΔR values of ours and Kong and Lee’s (Reference Kong and Lee2005) are applied to calculate the weighted mean ΔR. We report two weighted mean ΔR values of –83 ± 16 and –134 ± 100 (Table 3). The former is based on only the two values from our specimen, while the latter combines ours and Kong and Lee’s (Reference Kong and Lee2005) (Table 1). A larger variation of ΔR between ours and Kong and Lee’s (Reference Kong and Lee2005) contributes to a greater error range in the weighted mean ΔR of the latter.
Although Shell Layer 1 revealed the Yunggimun (appliqué) pottery, a typical style of the Initial Neolithic period (8000–7000 cal BP), a majority of pottery found are typical Early Neolithic wares with impressed, punctated, and incised decorations. Based on these pottery types and 14C dates on charcoal fragments (Table 3, Figure 4C), Shell Layer 1 was regarded as the Early Neolithic period (7000–5500 cal BP) (Gimhae National Museum 2008, 2012). As Early Neolithic type vessels were in use for over 1500 years, the pottery typology itself cannot answer specific diachronic questions, including how long inhabitants used the area and when it happened within an over 1,500 year span. We can address the occupational span of Shell Layer 1 by comparing ΔR adjusted dates on shells (Figure 4A, 4B) with calibrated dates on charcoal and animal bones (Figure 4C).
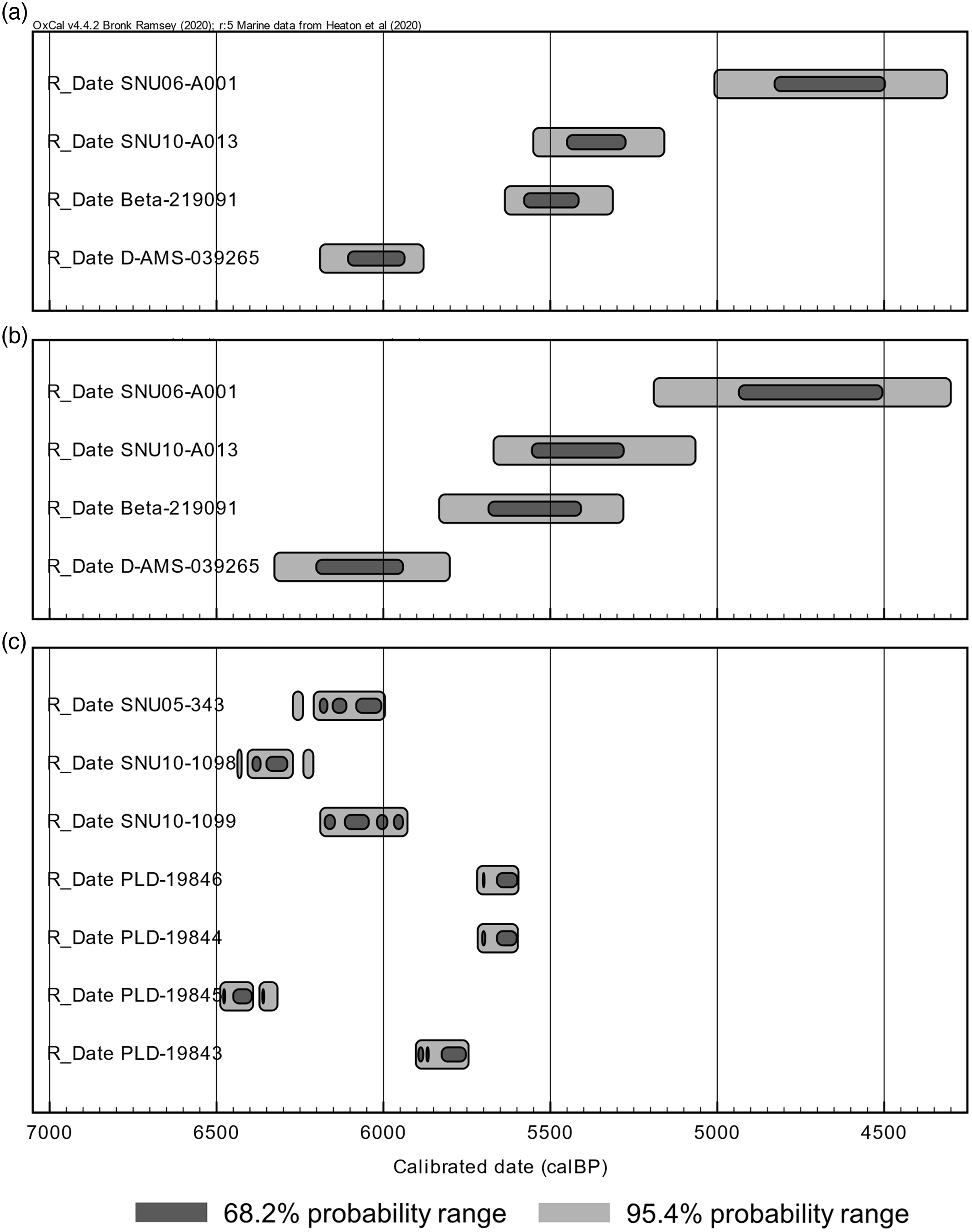
Figure 4 Comparison of 11 14C dates from Shell Layer 1 at the Bibongri site: (a) Dates on shells calibrated by the weighted mean ΔR value of –83 ± 16 derived from the pre-bomb shell in this study (see Table 2). (b) Dates on shells calibrated by the weighted mean ΔR value of -134 ± 100 derived from the pre-bomb shell in this study and Kong and Lee (Reference Kong and Lee2005). (c) Calibrated dates on charcoal and terrestrial animal bones. Calibration performed with OxCal 4.3.2 (Bronk Ramsey Reference Bronk Ramsey2009, Reference Bronk Ramsey2017; Bronk Ramsey and Lee Reference Bronk Ramsey and Lee2013), based on IntCal20 atmospheric curve and Marine20 marine curve (Heaton et al. Reference Heaton, Köhler, Butzin, Bard, Reimer, Austin, Bronk Ramsey, Grootes, Hughen, Kromer, Reimer, Adkins, Burke, Cook, Olsen and Skinner2020; Reimer et al. Reference Reimer, Austin, Bard, Bayliss, Blackwell, Bronk Ramsey, Butzin, Cheng, Edwards, Friedrich, Grootes, Guilderson, Hajdas, Heaton, Hogg, Hughen, Kromer, Manning, Muscheler, Palmer, Pearson, van der Plicht, Reimer, Richards, Scott, Southon, Turney, Wacker, Adolphi, Büntgen, Capano, Fahrni, Fogtmann-Schulz, Friedrich, Köhler, Kudsk, Miyake, Olsen, Reinig, Sakamoto, Sookdeo and Talamo2020).
The stable isotopic analysis on bones from Shell Layer 1, which were also dated, does not raise a concern of sample contamination, collagen degradation, and carbon-exchange issues. The atomic carbon to nitrogen ratios measured from the bone collagens are 3.1 to 3.3 (Kim Reference Kim2012). As the ratios of modern animals and humans range 2.9 to 3.6, if an archaeological sample falls within this range, that sample is regarded as reliable (DeNiro Reference DeNiro1985). Four bones’ collagen yields are around 13.2 weight percent collagen and are within the reliable range of 1.0 to 20.0 (van Klinken Reference Van Klinken1999).
We calibrated dates on archaeological shells with the two weighted mean ΔR values, one based on our samples only, –83 ± 16 (Figure 4A) and the other that combined ours and Kong and Lee’s (Reference Kong and Lee2005), –134 ± 100 (Figure 4B). Regardless to which of the two weighted mean ΔR values are used, charcoal and animal bone dates are several hundred years older than two shell dates (SNU10-A013, Beta-219091). The other shell date, D-AMS-039265, is roughly contemporaneous to charcoal and animal bone dates (Figure 4, Table 3). The shell date, SNU06-A001, is much younger than all other dates found in the same layer. Also, it has the least precision (i.e., large uncertainty), indicating the possibility that the sample was contaminated during the laboratory procedure or relocated from the layer above. Calibrated date ranges of archaeological shells increase by 100–200 years if we apply the combined weighted mean ΔR that includes values published by Kong and Lee (Reference Kong and Lee2005) (Figure 4B).
The potential impact of the old-wood effect should be examined (Schiffer Reference Schiffer1986; Nolan Reference Nolan2012). Arboreal taxa identified at Bibongri through wood charcoal analysis include sawtooth oak (Quercus acutissima Carruth.), Korean red pine (Pinus densiflora), mulberry (Morus sp.), chestnut (Castanea sp.), and maple (Acer sp.) (Lee and Oh Reference Lee and Oh2008). Pine and oak can live up to 1000 years under the right conditions. A previous study, however, suggests that the old-wood effect did not produce any consistent difference between the dates on annual seeds and wood charcoal from several Neolithic and Bronze period sites in Korea (ca. 8000–3500 BP) (Hwang et al. Reference Hwang, Kim, Lee, Lee, Song, Kim, Park, Yang, Yang, Kang, Oh, Ahn, Choi, Seong, Wright, Choi and Hyun2016). Contrary to the expectation of the old-wood effect, seeds appeared to be about 35 years older than charcoal on average. A contrasting pattern has been observed in historical sites (ca. 1700–1100 cal BP), where the dates on charcoal were older than those on seeds. In historical periods, thicker and older lumbers were likely used to build long-term houses, contributing to the old-wood effect (Hwang et al. Reference Hwang, Kim, Lee, Lee, Song, Kim, Park, Yang, Yang, Kang, Oh, Ahn, Choi, Seong, Wright, Choi and Hyun2016).
Schiffer (Reference Schiffer1986) first warned the old-wood effect by examining the dates from the southwest United States. However, Cook and Comstock (Reference Cook and Comstock2014) identified a much lesser degree of old-wood effects from the Fort Ancient sites (2200–1600 BP) across southwestern Ohio and southeastern Indiana. They found that the decomposition rates of wood are generally very high in the temperate climate there. That is, the availability of old wood is much lower in the temperate climate than in the arid zone. Southeastern Korea is in a temperate climate zone with distinct seasonal cycles of hot humid summer with monsoon, followed by dry cold winter. This climate condition may reduce the old-wood effect, similar to what Cook and Comstock (Reference Cook and Comstock2014) observed in the United States.
Diatom analysis also indicates that Bibongri was influenced by saline seawater (Hwang Reference Hwang2008). Salty water is known to promote the decaying of wood (Shupe et al. Reference Shupe, Lebow and Ring2008). Wood probably had a very low chance to survive for a substantive period of time in a seasonally wet condition and a saline water influx at Bibongri. The old-wood effect is less likely to have occurred at Bibongri unless wood materials have a highly durable composition like those in the early historical period in Korea. Accordingly, we can conclude that the age difference between charcoal and shells unlikely resulted from an old-wood effect. The earliest date on charcoal (SNU10-1098) and the latest date on shell (SNU10-A013) are several hundred years apart at the 2 sigma level. The range suggests that Shell Layer 1 was accumulated over an extended period of time between 6400 and 5000 cal BP.
Our data show that the use of marine reservoir correction values (ΔR) on marine shells can improve our understanding of the site chronology. A chronology based heavily on a pottery typology can only suggest that an entire stratum over 1 m thick belongs to a singular “Early Neolithic” period. The stratigraphic sequence and the site’s specific duration of use cannot be understood exclusively based on pottery typology or uncalibrated radiocarbon years of marine and aquatic species. With an accurate calibration of marine dates and charcoal, we are able to understand the depth of time span through which multiple generations inhabited the area.
Bibongri is a multi-generational site used by those who shared the Early Neolithic pottery tradition over hundreds of years. Early Neolithic people likely dwelled at or revisited Bibongri to take advantage of its broad-spectrum resources over generations. Archaeobotanical and zooarchaeological studies conducted at the site reflect diverse seasonal marine and terrestrial resources throughout the year (Kaneko Reference Kaneko2008; Kwak et al. Reference Kwak, Obata and Lee2020). Bibongri presented an affluent environment during the period where these shell middens were used. Numerous artifacts that represent diverse activities were recovered from Shell Layer 1, including digging tools, stone knives, axes, adzes, whetstones, grinding stones, spears, fishing hooks, and net sinkers (Gimhae National Museum 2008, 2012). This diverse combination of tools and dwelling structures represent at least multi-seasonal settlements at Bibongri. Diverse seasonal resources, which are reflected in plant and animal remains, were attractive to Bibongri people; they were able to sustain themselves through fishing-shellfishing, hunting, wild plant harvesting, and millet cultivation during the Early Neolithic period (Kwak et al. Reference Kwak, Obata and Lee2020). A long tradition of such broad-spectrum resource use probably made Bibongri a vital cultural niche over several hundred years, similar to the findings on the east coast of Korea (Lee et al. Reference Lee, Cho and Obata2019).
CONCLUSION AND FUTURE DIRECTIONS
We added two additional ΔR values to the Pacific database by dating the pre-bomb (pre-1950) blue mussel shell (Mytilus edulis) from the southern coastal region of Korea. As a pioneering case study, we calibrated archaeological shell samples from Shell Layer 1 of the Bibongri Neolithic shell midden, where dates on charcoal are also available. By comparing the dates on charcoal samples with the ΔR calibrated shell dates, we demonstrate that Shell Layer 1 represents long-term human activities over several hundred years, and thus improves our understanding of the site chronology. Our Bibongri case study demonstrates that properly calibrated shell dates can play a key role in improving the chronological understanding of Neolithic shell midden in Korea. Moving forward, we will investigate the variation of ΔR across the Korean Peninsula and its contributing causes by reporting additional ΔR using pre-bomb shell samples and pairing them with the dates on terrestrial samples to build a fine-grained chronology of the Neolithic Period.
ACKNOWLEDGMENTS
This work was supported by the Laboratory Program for Korean Studies through the Ministry of Education of the Republic of Korea and Korean Studies Promotion Serive of the Academy of Korean Studies (AKS-2015-Lab-2250001). A special thanks to Hae-jin Chang at the Fisheries Science Museum (a branch of the National Fisheries Research and Development Institute) and Jung-Jin Kim at the Coastal Water Fisheries Resources Research Division of the National Institute of Fisheries Science for providing us with the pre-bomb bivalve specimens. We appreciate Hakjong Im (Gimhae National Museum) and Junggeun Lee (Jinju National Museum) for providing us with flotation samples and photos of the Bibongri site. Our gratitude goes out to Sangtaek Lim (Pusan National University) who helped us to receive modern shell specimens. We are grateful to Amira Ainis (University of Oregon) for training us in sample processing, and to Ilya Bindeman and Jim Palandri (University of Oregon) in isotopic analysis. We appreciate Geesoo Kong (Korea Institute of Geoscience and Mineral Resources) for guidance in accessing pre-bomb shell samples. We are also thankful to Scott Fitzpatrick (University of Oregon) for allowing us to prepare our samples in the Coastal and Island Archaeology Laboratory.
Supplementary material
To view supplementary material for this article, please visit https://doi.org/10.1017/RDC.2021.45