Introduction
The substantial increase in screen time exposure among young children is a growing concern in the electronic media age. Screen-based media has exploded over the past 20 years, fundamentally altering the way humans interact with one another and transforming the way a child learns and explores the environment, exacerbated by the recent COVID-19 pandemic lockdowns. An estimated 90% of children under the age of two are regularly exposed to electronic media (Brown, Reference Brown2011). Infants are on average exposed to 2 to 3 h of screen time per day (Chen & Adler, Reference Chen and Adler2019), far exceeding the existing recommendation by the World Health Organization (WHO).
Excessive screen time during childhood has been associated with behavioral and cognitive outcomes (Christakis, Ramirez, Ferguson, Ravinder, & Ramirez, Reference Christakis, Ramirez, Ferguson, Ravinder and Ramirez2018; Kirkorian, Pempek, Murphy, Schmidt, & Anderson, Reference Kirkorian, Pempek, Murphy, Schmidt and Anderson2009; Zimmerman, Christakis, & Meltzoff, Reference Zimmerman, Christakis and Meltzoff2007), including the socio-emotional competence (Kerai, Almas, Guhn, Forer, & Oberle, Reference Kerai, Almas, Guhn, Forer and Oberle2022). Previous research has demonstrated a negative link between screen time and the social-emotional function of preschoolers (del Pozo-Cruz et al., Reference del Pozo-Cruz, Perales, Parker, Lonsdale, Noetel, Hesketh and Sanders2019). However, most studies on screen time have been focused on children at preschool or school-going ages. Notably, existing findings might not translate directly to infants, as the first two years of life represent a time period of expeditious pace of brain development (Gilmore, Knickmeyer, & Gao, Reference Gilmore, Knickmeyer and Gao2018). Our study addressed this knowledge gap by focusing specifically on the outcomes associated with screen time in infancy.
Early childhood represents a crucial period of brain development characterized by experience-dependent synaptic plasticity, which is fundamental for brain network maturation. Individuals are especially susceptible to environmental stimuli during such highly sensitive periods of brain development (Woodard & Pollak, Reference Woodard and Pollak2020). Previous studies reveal significant associations between screen time exposure and neural development. Increased screen time in early childhood is associated with a decline in the integrity of white matter tracts known to support language and literacy skills (Hutton, Dudley, Horowitz-Kraus, DeWitt, & Holland, Reference Hutton, Dudley, Horowitz-Kraus, DeWitt and Holland2020). Increased screen time utilization in childhood has also been linked to changes in global white matter microstructure (Rodriguez-Ayllon et al., Reference Rodriguez-Ayllon, Derks, van den Dries, Esteban-Cornejo, Labrecque, Yang-Huang and Muetzel2020). However, the neural mechanisms underlying the established association between screen time and later socio-emotional competence remain to be examined.
The organization of brain networks is a hallmark of postnatal brain development, and significant network topology evolution is predicted to occur during early childhood (Fransson, Åden, Blennow, & Lagercrantz, Reference Fransson, Åden, Blennow and Lagercrantz2011). The organization of brain networks and their complex interactions supports neurodevelopmental function (Akarca et al., Reference Akarca, Vértes, Bullmore, Baker, Gathercole, Holmes and Astle2021). While the brain can be conceptualized as multiple modules with distinct functions, higher order neural functions, such as social and emotional processing, arise from the spatiotemporal dynamics of the entire brain network (Avena-Koenigsberger, Misic, & Sporns, Reference Avena-Koenigsberger, Misic and Sporns2018) and span multiple modules. The interactions between modules are supported by the large-scale, non-modular anatomical and functional brain architecture (Pessoa, Reference Pessoa2018). Therefore, our network-based approach could potentially provide further insight on the neural substrate for the association between early childhood screen time and socio-emotional development, and supplement previous findings using simple functional connectivity (Horowitz-Kraus & Hutton, Reference Horowitz-Kraus and Hutton2018) and diffusion tractography (Hutton et al., Reference Hutton, Dudley, Horowitz-Kraus, DeWitt and Holland2020) between specific regions.
The generation of an emotional response involves the perception of stimuli, the deployment of attention, and the appraisal of significance, taking into account both positive and negative valence (Silvers, Buhle, & Ochsner, Reference Silvers, Buhle, Ochsner, Ochsner and Kosslyn2013). Positive valence leads to approach and consummatory behaviors, while negative valence leads to defensive and avoidance behaviors. Dysregulation of the valence circuit underlies various psychopathologies, including those intimately related to emotional dysregulation, such as depression and anxiety. The generation of affective valence is highly dependent on brain regions that are major nodes of the reward and emotion processing networks, such as the amygdala, hippocampus, and nucleus accumbens. Aside from the assignment of valence, top-down control processes governed by the cognitive control network are also critical for successful emotional regulation. The cognitive control network implements emotion regulation strategies such as situation modification, inhibiting prepotent responses, attention selection, cognitive change (the way one thinks about an emotional stimulus), updating information in working memory, shifting mental sets, and response modification (Silvers et al., Reference Silvers, Buhle, Ochsner, Ochsner and Kosslyn2013). We therefore focused on the topological properties of the cognitive control, emotion processing, and reward processing networks as potential mechanisms linking screen time exposure to socio-emotional competence. In addition to allowing for a novel mediational analysis of candidate mechanisms, our study provided a unique opportunity to examine the sustained influence of screen time exposure in infancy on neuroanatomy later in childhood.
We postulate that excessive screen time is an unfavorable environmental factor that alters the expected trajectory of socio-emotional development. Socio-emotional development in children refers to the development of skills to effectively interact with others and their environment (Rose-Krasnor, Reference Rose-Krasnor1997). Specifically, the skills can be broadly classified under the following: correct communication, identification of emotional states, perspective regulation, emotional regulation, and social problem solving empathy (Halberstadt, Denham, & Dunsmore, Reference Halberstadt, Denham and Dunsmore2001; Rubin, Bukowski, & Parker, Reference Rubin, Bukowski, Parker, Damon, Lerner and Eisenberg2007). In our study, we utilized the emotional regulation index (ERI) of the Behavior Rating Inventory of Executive Function, 2nd Edition (BRIEF-2), as a specific measurement of emotional dysregulation, and the Social Emotional Assets and Resilience Scales, parent-reported (SEARS) as a general measure of socio-emotional competence, which broadly encompasses the range of skills above. Reduced socio-emotional competence has been linked to lower peer acceptance (Denham, McKinley, Couchoud, & Holt, Reference Denham, McKinley, Couchoud and Holt1990), aggressive behaviors (Eisenberg, Fabes, Guthrie, & Reiser, Reference Eisenberg, Fabes, Guthrie and Reiser2000), and externalizing problems (Groh, Fearon, van IJzendoorn, Bakermans-Kranenburg, & Roisman, Reference Groh, Fearon, van IJzendoorn, Bakermans-Kranenburg and Roisman2017). Furthermore, it is also a common transdiagnostic feature of many mental health disorders (Biele, Overgaard, Friis, Zeiner, & Aase, Reference Biele, Overgaard, Friis, Zeiner and Aase2022; Thomson et al., Reference Thomson, Richardson, Gadermann, Emerson, Shoveller and Guhn2019).
Excessive screen time also reduces the quality and quantity of parent–child interactions (Kostyrka-Allchorne, Cooper, & Simpson, Reference Kostyrka-Allchorne, Cooper and Simpson2017) by limiting opportunities for verbal and nonverbal social exchanges that promote cognitive, emotional, and social development in young children (Hollenstein, Tighe, & Lougheed, Reference Hollenstein, Tighe and Lougheed2017). These results suggest that the adverse influence of excessive screen time exposure could arise by displacing time for reading and play-based activities that enhance social-emotional skills. Furthermore, reading time and screen time show opposite effects on seed-based functional connectivity maps (Horowitz-Kraus & Hutton, Reference Horowitz-Kraus and Hutton2018). The American Academy of Pediatrics (AAP) recommends that parent–child reading begin as soon as possible after birth for long-term cognitive and social-emotional benefits (High et al., Reference High, Klass, Donoghue, Glassy, DelConte, Earls and Williams2014). Parent–child reading is also a strong predictor of brain development (Mustard, Reference Mustard2006). Neuroimaging-based research has provided neurobiological support for the link between parent–child reading and activation in brain areas supporting complex language and social-emotional integration in preschool children (Hutton et al., Reference Hutton, Phelan, Horowitz-Kraus, Dudley, Altaye, DeWitt and Holland2017). Using near-infrared spectroscopy, Ohgi et al., demonstrated that mother–child engagement during picture-book reading was consistently associated with increased neural activity in the child's frontal lobes, which are critical for emotion regulation (Ohgi, Loo, & Mizuike, Reference Ohgi, Loo and Mizuike2010). Parent–child shared reading has also been shown to enhance widespread functional connectivity in the child's brain (Hasegawa et al., Reference Hasegawa, Takahashi, Ikeda, Yoshimura, Hiraishi, Nobukawa and Kikuchi2021). Moreover, significantly higher neural activation was observed during picture-book reading than during video viewing (Ohgi et al., Reference Ohgi, Loo and Mizuike2010). For these reasons, we explored whether parent–child reading may have the capacity to mitigate the effects of screen time on brain network development.
Our analyses capitalized on our deeply-phenotyped large population-based birth cohort study, Growing Up in Singapore Towards healthy Outcomes (GUSTO). Extensive measures of screen time exposure were collected between the ages of 1 and 4 years, followed by diffusion MRI at age 6 years and assessments of socio-emotional competence (BRIEF and SEARS) at age 7 years. At the age of 3, data on the quantity of parent–child reading was collected. Diffusion MRI was used to compute measures of network topology based on the graph-theoretical model. The graph-theoretical model can reliably quantify brain networks with a small number of neurobiologically meaningful measures (Achard, Salvador, Whitcher, Suckling, & Bullmore, Reference Achard, Salvador, Whitcher, Suckling and Bullmore2006; Hagmann et al., Reference Hagmann, Cammoun, Gigandet, Meuli, Honey, Wedeen and Sporns2008; He, Chen, & Evans, Reference He, Chen and Evans2007; Sporns & Zwi, Reference Sporns and Zwi2004) and is used extensively in neuroimaging research as a methodological tool to examine brain network topology (Bullmore et al., Reference Bullmore, Barnes, Bassett, Fornito, Kitzbichler, Meunier and Suckling2009; Hagmann et al., Reference Hagmann, Cammoun, Gigandet, Meuli, Honey, Wedeen and Sporns2008; Sporns, Chialvo, Kaiser, & Hilgetag, Reference Sporns, Chialvo, Kaiser and Hilgetag2004). To our knowledge, our study is the largest cohort exploring the downstream neural effects of screen time exposure in infancy. Figure 1 depicts both the design of our study and the analytical approaches.
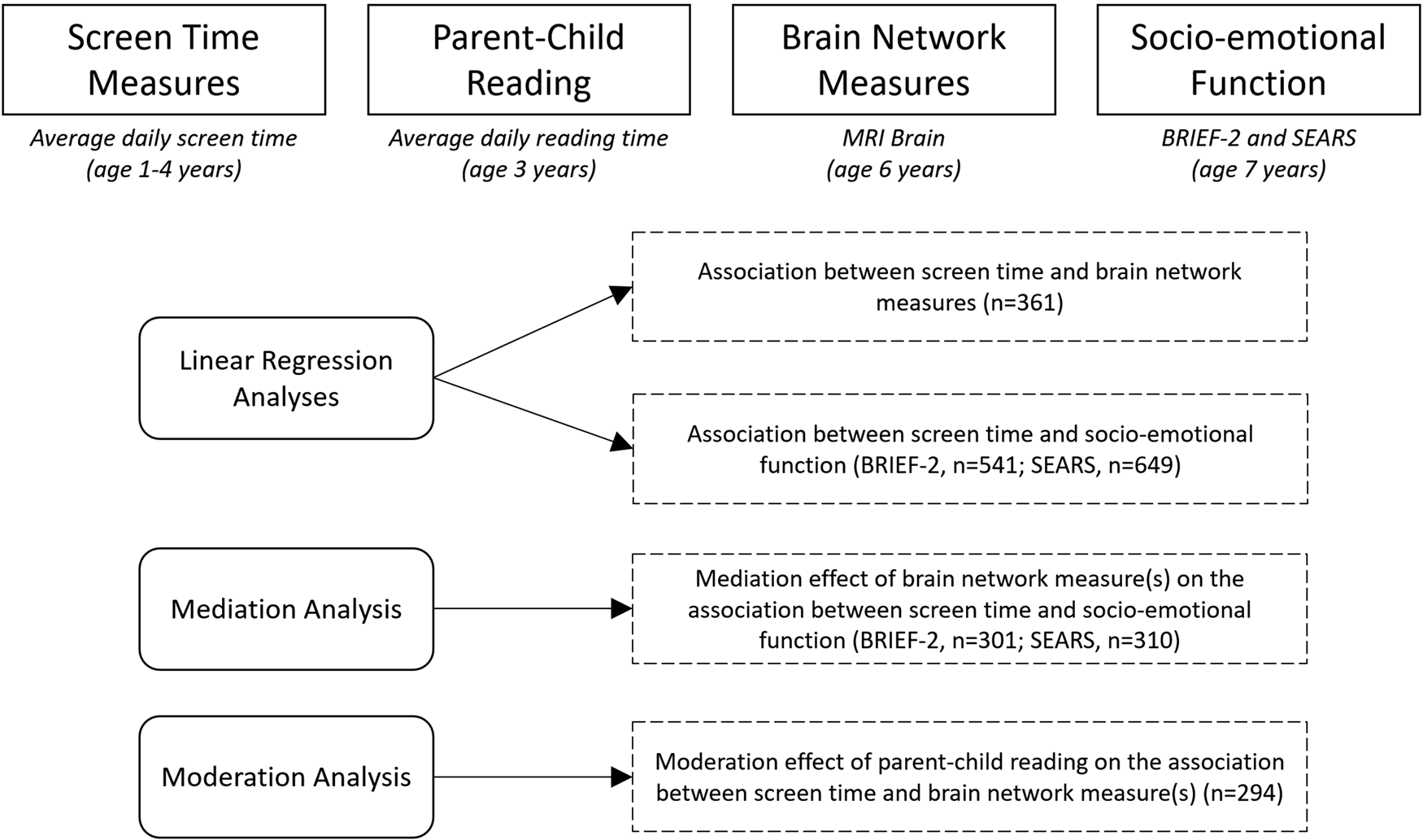
Figure 1. Study design and analytical approach. We collected screen time measures at 1–4 years of age and measures of parent–child reading at age 3. An MRI brain was performed at age 6, from which measures of brain network topology were computed. This is followed by evaluation of socio-emotional competence at age 7 using the BRIEF-2 and SEARS parental questionnaires. Note: BRIEF-2: Behavior Rating Inventory for Executive Function-Version 2, SEARS: Social Emotional Assets and Resilience Scales, n = number of participants.
In this study we investigated the interplay between screen time usage, brain network topology, and later socio-emotional competence. Specifically, we conceptualized alterations in brain network topology as a plausible pathway that links screen time in infancy with later socio-emotional competence. We also examined whether parent–child reading time could play a role as a moderator in this relation. We hypothesized that (1) screen time in infancy would be significantly associated with brain network topology and socio-emotional competence in later childhood, (2) brain network topology would significantly mediate the link between screen time in infancy and socio-emotional competence at age 7, and (3) the quantity of parent–child reading would significantly moderate the relationship between screen time in infancy and brain network topology.
Methods
Participants
Participants were recruited from GUSTO, an ongoing population-based, prospective cohort with the aim of understanding developmental influences on long-term health outcomes (Soh et al., Reference Soh, Tint, Gluckman, Godfrey, Rifkin-Graboi, Chan and Saw2014). Women aged 18 years or older across all socioeconomic backgrounds were recruited in their first trimester of pregnancy from two main public hospitals in Singapore between June 2009 and December 2010 (Soh et al., Reference Soh, Tint, Gluckman, Godfrey, Rifkin-Graboi, Chan and Saw2014). Mother–child dyads were followed throughout and after pregnancy. The study was approved by the SingHealth Centralized Institutional Review Board and the National Health Group Domain Specific Review Board. Mothers signed an informed consent prior to enrollment in the cohort, and all children assented to the study at 7 years of age. The study adhered to the Strengthening the Reporting of Observational Studies in Epidemiology (STROBE) guidelines for reporting cohort studies (Von Elm et al., Reference Von Elm, Egger, Altman, Pocock, Gøtzsche and Vandenbroucke2007). All children involved in this study were healthy individuals with no pre-existing neurodevelopmental conditions. Only children with gestational age at birth ⩾34 weeks, birth weight ⩾2000 g, and a 5-minute Apgar score ⩾8 were included to minimize potential birth complication effects on brain development. Baseline characteristics of study variables are provided in Table 1. Briefly, screen time usage data was collected at ages 1, 2, 3, and 4 years, parent–child reading time was collected at age 3 years, MRI data was collected at age 6 years and measures of socio-emotional competence at age 7 years.
Table 1. Baseline characteristics of study variables
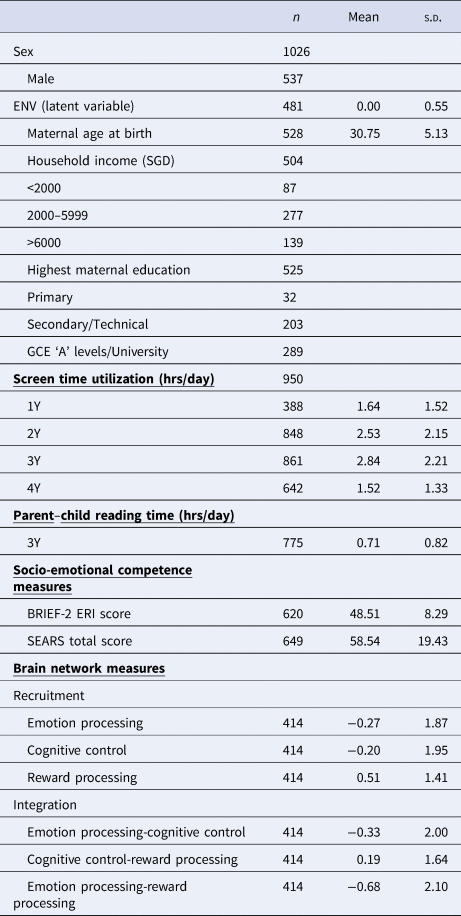
Note: ENV, environment latent variable; BRIEF-2, Behavior Rating Inventory for Executive Function-Version 2; ERI, Emotional Regulation Index; SEARS, Social Emotional Assets and Resilience Scales; n, number of participants.
Screen time measures
Screen time exposure was assessed at ages 1, 2, 3, and 4 years. Data on the total amount of parent-reported screen viewing time in a typical week was collected. The WHO recommends no screen time for children under 2 years of age. On this premise, we averaged the normalized screen time at 1 and 2 years to generate our primary exposure variable, screen time utilization under age 2, which will hereafter be referred to as screen time in infancy. Average normalized screen time at 3 and 4 years was used as a control variable given the strength of correlation between screen time measures across timepoints (r = 0.40–0.51) (Bernard et al., Reference Bernard, Padmapriya, Chen, Cai, Tan, Yap and Müller-Riemenschneider2017).
Screen time data were available for at least one time point for 950 participants. K-nearest neighbors (K-NN) data imputation method was used to generate estimates of the missing data. There are a total of 387 participants with one missing time point and 317 participants with two missing time points. The detailed breakdown of the number of participants for each timepoint is shown in Table 1. The K-NN model is a widely used method to impute missing values and is based on the identification of ‘k’ samples in the dataset that are similar. Specifically, we calculated the Euclidean distance between each participant using their screen time data. We then replaced the missing screen time timepoint with the average of the corresponding value from the k samples with the shortest Euclidean distance from the participant (k-nearest neighbor). We used a k hyperparameter of 31, approximately the square root of our sample size (Devroye, Gyorfi, & Lugosi, Reference Devroye, Gyorfi and Lugosi2014).
Measure of parent–child reading
Parent–child reading time was extracted from a questionnaire administered at age 3. Parents reported average reading time during a typical weekday and a weekend. A weighted average of the two reported values was used to calculate the average daily parent–child reading time.
Socio-demographic data
Socio-demographic data assessing environmental quality were collected from parental questionnaires and used to derive a latent environment variable (ENV) used in subsequent analyses as a covariate of no interest (Rakesh, Whittle, Sheridan, & McLaughlin, Reference Rakesh, Whittle, Sheridan and McLaughlin2023; Tooley, Bassett, & Mackey, Reference Tooley, Bassett and Mackey2021). A confirmatory factor analysis was performed with the Lavaan package in R (Rosseel, Reference Rosseel2012) to construct a latent environment variable (ENV) from three observed variables: (1) household income, (2) maternal education, and (3) maternal age at recruitment. All three variables significantly contributed to the latent variable (all p < 0.001), with standardized estimates as shown in online Supplementary Figure 1. These variables chosen were common demographic variables indicative of socio-economic status. This factor has been used in previous studies in the GUSTO cohort (Huang et al., Reference Huang, Tint, Lee, Ngoh, Gluckman, Chong and Tan2023).
MRI acquisition
Participants underwent magnetic resonance imaging (MRI) of the brain at age 6 utilizing a 3-Tesla scanner (Magnetom Skyra; Siemens, Germany). Diffusion-weighted images were acquired using a single-shot echo planar imaging (EPI) sequence with the following imaging parameters: field of view = 192 × 192 mm2, voxel size = 2 mm isotropic, repetition time = 8200 ms, echo time = 85 ms, flip angle = 90 degrees, 30 non-collinear directions, b values = 0, 1000 s/mm2, acceleration factor = 3. Three-dimensional T1-weighted images were acquired using a Magnetization-Prepared Rapid Gradient-Echo (MP-RAGE) Sequence with the following imaging parameters: repetition time = 2000 ms, echo time = 2.08 ms, inversion time = 877 ms, field of view = 192 × 192 mm2, voxel size = 1 mm isotropic, acceleration factor = 3.
Selection of regions of interest (ROIs)
Core brain regions of the emotion processing network (amygdala, hippocampus) (Bird & Burgess, Reference Bird and Burgess2008; Fanselow & Dong, Reference Fanselow and Dong2010), the reward processing network (nucleus accumbens, orbital frontal cortex, anterior cingulate cortex) (Jia et al., Reference Jia, Macare, Desrivières, Gonzalez, Tao, Ji and Ziesch2016) and the cognitive control network (dorsolateral prefrontal cortex, lateral posterior parietal cortex) (Niendam et al., Reference Niendam, Laird, Ray, Dean, Glahn and Carter2012) were selected as ROIs, giving a total of 14 bilateral ROIs. These ROIs were selected to focus on the core regions of the networks, avoid regions with heterogeneous and overlapping function and to emphasize subcortical-cortical connectivity. This approach is based on the knowledge that children exhibit stronger subcortical-cortical and weaker cortico-cortical connectivity compared to adults (Menon, Reference Menon2013). However, it is crucial to acknowledge that the anterior cingulate cortex, as a critical region that underlies cognitive, social, and emotional development, is intimately related to all three networks under investigation in our study, with established connections with all other ROIs.
Construction of structural connectivity matrix
Diffusion datasets were preprocessed using tools implemented in FMRIB's Software Library (FSL, v6.0) (Smith et al., Reference Smith, Jenkinson, Woolrich, Beckmann, Behrens, Johansen-Berg and Matthews2004). Within-voxel probability density functions of the principal diffusion direction were estimated using Markov Chain Monte Carlo sampling in FSL's BEDPOSTX tool (Behrens, Berg, Jbabdi, Rushworth, & Woolrich, Reference Behrens, Berg, Jbabdi, Rushworth and Woolrich2007). A spatial probability density function was then estimated using FSL's PROBTRACKX tool (Behrens et al., Reference Behrens, Woolrich, Jenkinson, Johansen-Berg, Nunes, Clare and Smith2003). The Mindboggle 101 atlas (Klein et al., Reference Klein, Ghosh, Bao, Giard, Häme, Stavsky and Keshavan2017) was used to delineate the set of predetermined ROIs. Pairwise structural connectivity was obtained from the total number of tractography streamlines. A 14 × 14 structural connectivity matrix was generated and used to compute measures of brain network topology (see below). For each pairwise structural connectivity, we regressed out the effect of ROI volumes.
Computation of brain network topology
A tuned Louvain community detection algorithm (Blondel, Guillaume, Lambiotte, & Lefebvre, Reference Blondel, Guillaume, Lambiotte and Lefebvre2008) was used to segment ROIs into community clusters. This analysis generates a group assignment for each ROI used to create an allegiance matrix for the cognitive control (CC), emotion processing (EP), and reward processing (RP) networks. This analysis was iterated 100 times to generate an average allegiance matrix with stable estimates of network recruitment and network integration (Fig. 2). The recruitment coefficient is defined as the probability of a region being assigned to the same community as other regions from the same network, while the integration coefficient is defined as the probability of a region being assigned to the same community as regions from another network. In terms of interpretation, high network recruitment indicates greater communication of ROIs within the network itself, suggesting a higher degree of modularity and activity of the network. A high level of network integration indicates a higher level of communication between the ROIs of the two networks, suggesting a lower degree of modularity and higher integration between the two networks.
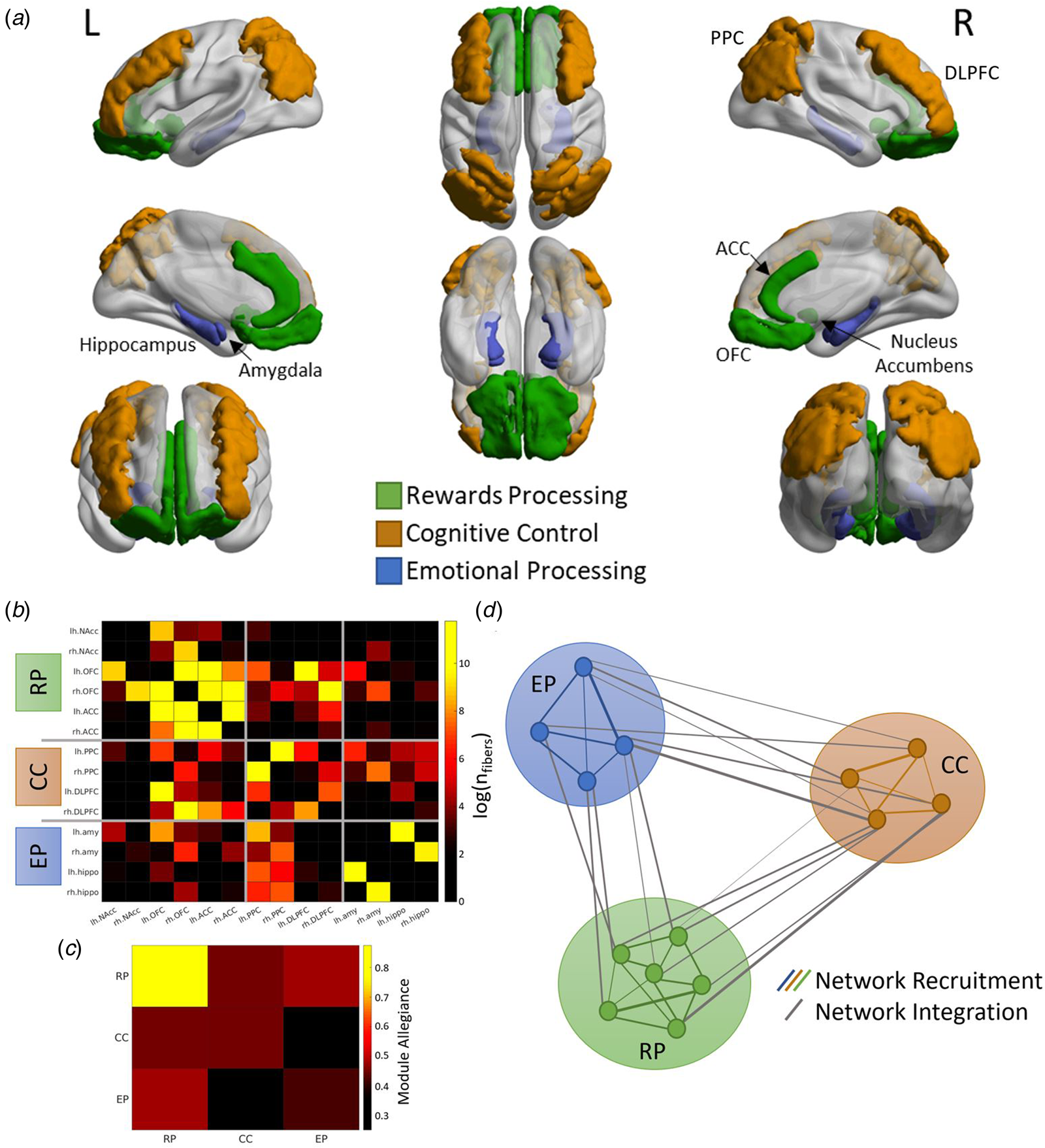
Figure 2. Extraction of network measures (A) Fourteen regions-of-interest (ROIs) were identified a priori, representing major nodes of the emotion processing (amygdala, hippocampus), reward processing (nucleus accumbens, orbital frontal cortex; OFC, anterior cingulate cortex; ACC), and cognitive control (dorsolateral prefrontal cortex; DLPFC, posterior parietal cortex; PPC) networks. (B) Structural connectivity matrix was generated using the total number of tractography streamlines between each pair of ROIs. (C) The ROIs were grouped into three networks (RP; reward processing, CC; cognitive control, EP; emotion processing) which were used to generate the allegiance matrix. The diagonal elements represent the network recruitment coefficient and the off-diagonal elements represent the network integration coefficient. (D) A graphical illustration of the network recruitment and network integration measures. Each circle represents a ROI, and the thickness of the lines represents the probability of the two ROIs being clustered together by the Louvain community detection algorithm.
We normalized these network topology measures using the mean values from 10 000 iterations with randomly permuted structural connectivity matrices to account for differences in the number of regions in each network (Finc et al., Reference Finc, Bonna, He, Lydon-Staley, Kühn, Duch and Bassett2020). Six network measures were calculated for each participant and used in subsequent analyses; (1) emotion processing network recruitment, (2) cognitive control network recruitment, (3) reward processing network recruitment, (4) emotion processing-cognitive control network integration, (5) cognitive control-reward processing network integration, and (6) emotion processing-reward processing network integration.
Measures of socio-emotional competence
We utilized two measures to capture two different aspects of socio-emotional competence. The ERI of the BRIEF-2 was used as a measure of emotional dysregulation and the SEARS total score was used as a general measure of socio-emotional competence.
Behavior Rating Inventory of Executive Function-2nd Edition (BRIEF-2; parent-report form) was administered at age 7 years (n = 620). BRIEF-2 is a rating scale that assesses everyday behaviors reflecting executive functions across the school-age span (ages 5–18) and contains 63 items within nine clinical scales. The ERI, is a subscale of BRIEF-2 and comprises of the Emotional Control and Shift scales. Elevated ERI scores indicate higher levels of emotional dysregulation.
Social Emotional Assets and Resilience Scales-Parent (SEARS; parent-report form) was also administered at age 7 years (n = 649). In contrast to BRIEF-2, which focuses on areas of difficulties and deficits, SEARS is a strength-based measure that focuses on social and emotional strengths. SEARS consists of 41 items, which are answered on a 4-point Likert scale. The SEARS total score was used in our analysis as a general measure of socio-emotional competence.
Statistical analysis
All statistical analyses were performed in Matlab R2022a. Sex, latent environment factor, and averaged normalized screen time at 3 and 4 years were included as covariates of no interest for all analyses. DTI motion parameters were included as covariates of no interest for all analyses involving brain network measures.
(i) Linear regression
We fitted a linear regression model to investigate the associations between (i) screen time in infancy and brain network topology (recruitment and integration coefficients) and (ii) screen time in infancy and socio-emotional competence in later childhood (BRIEF-2 ERI and SEARS total score). For multiple comparison purposes, Bonferroni correction of p-values was performed over six network measures. Brain network measures that were significantly associated with screen time were further examined in (ii) and (iii). Partial eta squared values, $\eta _p^2$, were also reported for significant terms in the linear regression as an estimate of effect size.
(ii) Mediation analysis
Using the M3 toolbox (Shrout & Bolger, Reference Shrout and Bolger2002) in Matlab, a standard three-variable mediation analysis was carried out to investigate the extent to which the association between screen time in infancy and socio-emotional competence in later childhood (BRIEF-2 ERI and SEARS total score) is mediated by the brain network measure(s) from (i). The bias-corrected significance of the mediation was estimated using a bootstrap method with 10 000 resamplings. Before the mediation analysis, the effect of the covariates of no interest was regressed.
(iii) Moderation analysis
We fitted a linear regression model with added interaction terms between screen time in infancy and parent–child reading time to investigate whether the quantity of parent–child reading had a moderating effect on the association between screen time in infancy and brain network topology. Partial eta squared value, $\eta _p^2$, for the interaction term was reported as an estimate of effect size.
(iv) Moderated mediation analysis
A moderated mediation analysis was carried out using the lavaan toolbox (Rosseel, Reference Rosseel2012) in R. We tested for the moderating effect of parent–child reading time on the role of brain network topology as a mediator between screen time in infancy and later socio-emotional competence. The bias-correct bootstrap percentile was estimated using a bootstrap method with 10 000 resamplings and post-hoc analysis using simple slopes was carried out for significant moderating effect. These results are presented in online Supplementary Table 2.
Results
Association between screen time in infancy and brain network topology
Linear regression analysis revealed that screen time in infancy was significantly associated with emotion processing-cognitive control network integration (β = 0.381, p = 0.005, $\eta _p^2$ = 0.024) at age 6 years. There was no significant association between screen time in infancy and the rest of the brain network measures (Fig. 3). Screen time was correlated with reward processing-cognitive control network integration (β = 0.238, p = 0.04, $\eta _p^2$
= 0.008), but this finding did not survive correction for multiple comparisons. Therefore, only the emotion processing-cognitive control network integration measure was used in subsequent analyses.
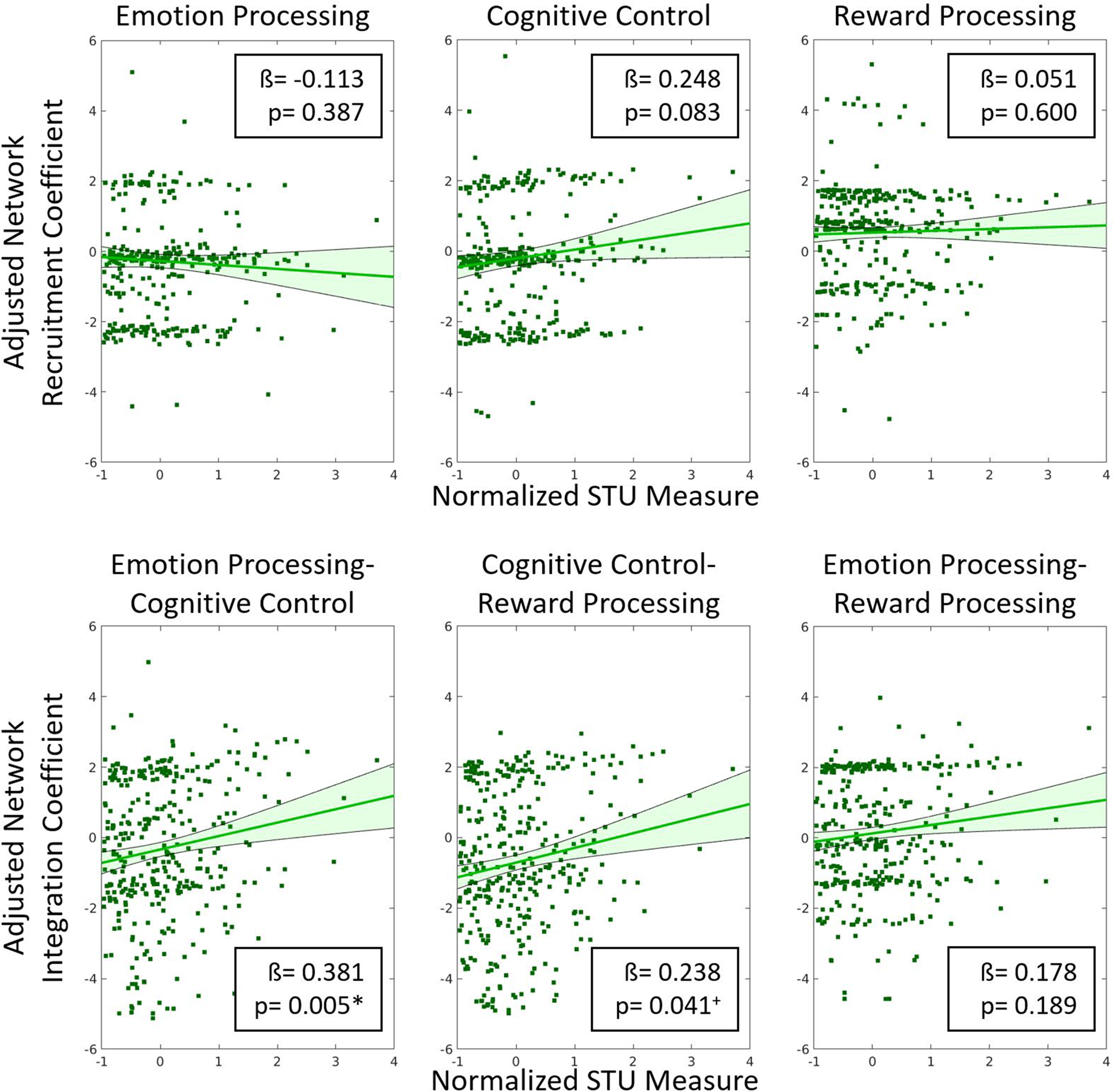
Figure 3. Residual plots showing the correlation between adjusted brain network integration and recruitment coefficients and the normalized screen time utilization (screen time) measure. All covariates were regressed out to generate the adjusted network measures. Beta coefficients and p-values are included in the insets. Our results show that only emotion processing-cognitive control network integration was significantly correlated with the normalized screen time measure. + indicates p < 0.05 (uncorrected) * indicates p < 0.008 (Bonferroni correction for multiple comparisons).
Association between screen time in infancy and later socio-emotional competence
Linear regression analysis revealed that screen time did not significantly predict BRIEF-2 ERI (β = 0.589, p = 0.186) (online Supplementary Table 1), suggesting the absence of a significant direct effect of screen time in infancy on emotional dysregulation in later childhood. However, our mediation analysis revealed that emotion processing-cognitive control network integration significantly mediated the effect of screen time on BRIEF-2 ERI (p = 0.036) (Fig. 4).
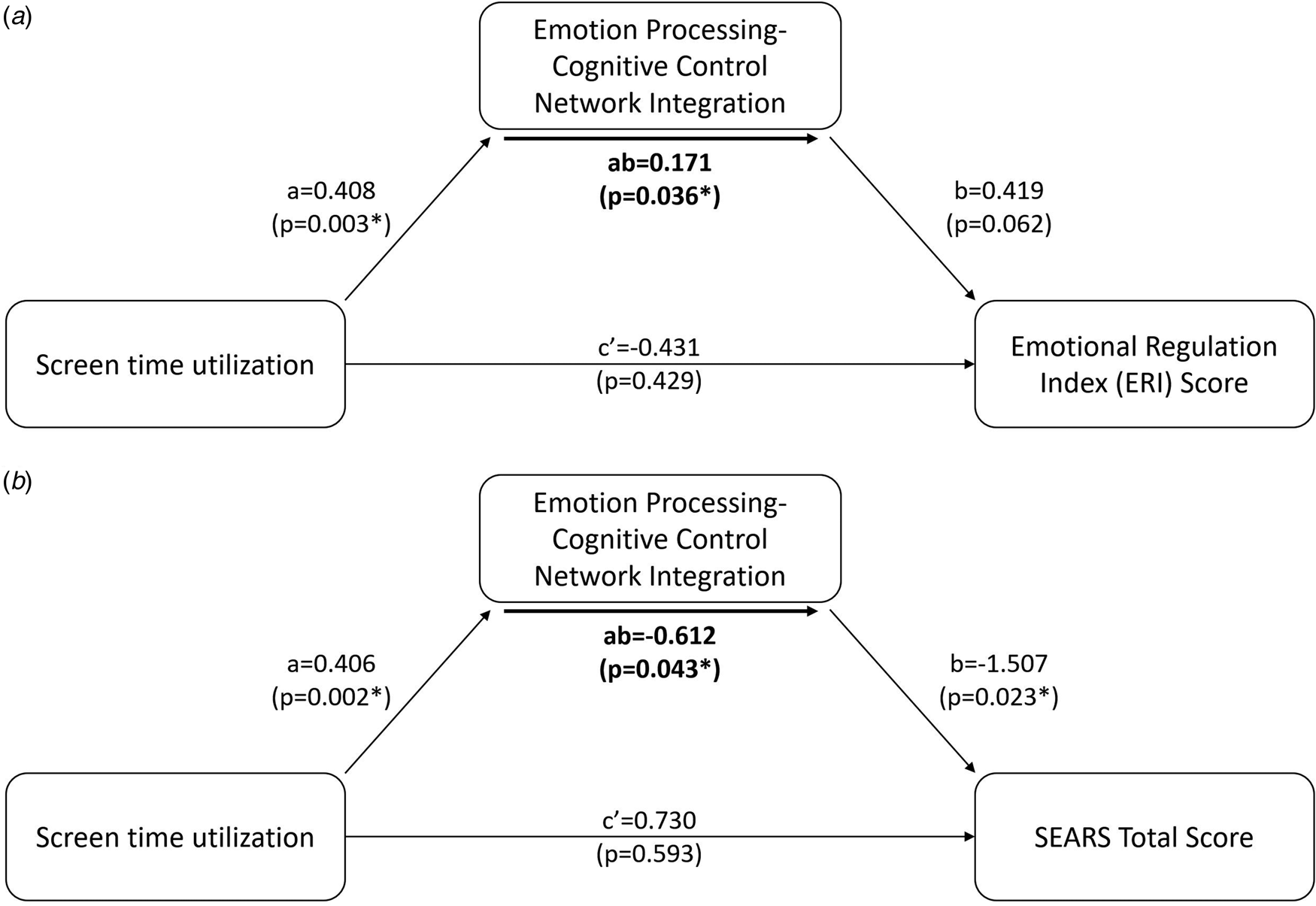
Figure 4. Mediation analysis revealed that the integration between emotional processing and cognitive control networks significantly mediates (p = 0.0362) the relationship between screen time and BRIEF-2 Emotional Regulation Index (ERI) score at 7 years (Panel A). Mediation analysis also revealed that the integration between emotional processing and cognitive control networks significantly mediates (p = 0.043) the relationship between screen time and SEARS total score at 7 years (Panel B). Specifically, increased screen time leads to increased emotion processing-cognitive control network integration. In turn, this leads to higher scores on the BRIEF ERI scale, indicating poorer emotion regulation abilities, and a lower SEARS total score, indicating decreased emotional resilience.
Linear regression analysis of the SEARS total score also showed no significant predictive value of screen time in infancy (β = −0.643, p = 0.529) (online Supplementary Table 1), again suggesting the absence of a significant direct effect of screen time on socio-emotional competence. Our mediation analysis again revealed that emotion processing-cognitive control network integration significantly mediated the effect of screen time in infancy on SEARS total score (p = 0.043) (Fig. 4).
In summary, our measures of emotional dysregulation and socio-emotional competence exhibited the same pattern. There was no significant direct effect of screen time, but a significant mediation effect where a higher level of screen time in infancy increased the integration between the emotion processing and cognitive control networks, which in turn resulted in higher emotional dysregulation and poorer socio-emotional competence.
Moderating effect of parent-child reading time
We found a significant moderating effect of parent–child reading time on the association between screen time in infancy and emotion processing-cognitive control network integration (β = −0.640, p = 0.005, $\eta _p^2$ = 0.028) (Fig. 5, Panel A). Specifically, parent–child reading time exhibited a buffering interaction effect on screen time exposure (Fig. 5, Panel B). At low levels of parent–child reading time, increased screen time in infancy is associated with greater integration between the emotion processing and cognitive control networks. Conversely, at high levels of parent–child reading time (1 standard deviation above the mean), increased screen time in infancy was unrelated to the degree of integration between the emotion processing and cognitive control networks.
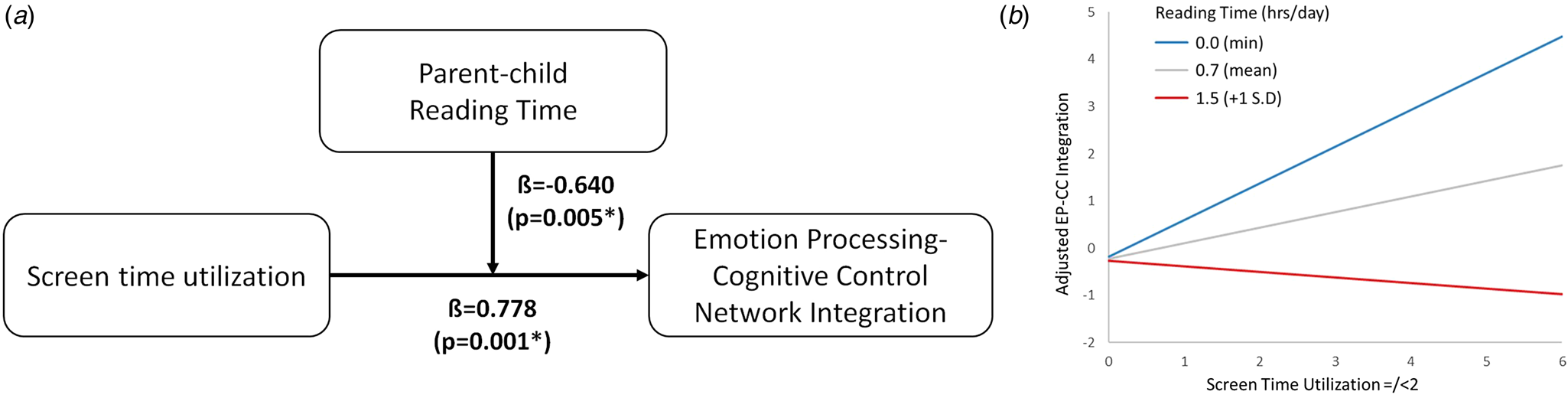
Figure 5. Parent–child reading shows a significant moderating effect (Panel A, p = 0.005) on the association between screen time and emotion processing-cognitive control network integration. At low levels of parent–child reading time, high levels of screen time increase the degree of emotion processing-cognitive control network integration (Panel B, blue; min). Conversely, at high levels of parent–child reading time, high levels of screen time do not increase the degree of emotion processing-cognitive control network integration (Panel B, red; one standard deviation above mean). −1 standard deviation was not plotted as there is no negative value for parent–child reading time.
Discussion
We provide novel evidence for an association between screen time exposure in infancy, brain network topology, and socio-emotional competence in later childhood. Our findings position the emotion processing-cognitive control network as a neural mechanism linking screen time exposure to alterations in socio-emotional development. Importantly, we found that this association is significantly moderated by parent–child reading, such that increased reading time buffers the influence of screen time exposure in infancy on the topological organization of the emotion processing and cognitive control networks. This finding is notable, as it suggests that encouraging parent–child reading may mitigate the effect of screen exposure on brain network development and socio-emotional competence.
Higher levels of screen time in infancy were found to be associated with a greater degree of integration of the emotion processing and cognitive control networks. Immediately after birth, the brain enters a consolidation phase characterized by prolonged myelination and competitive pruning (Huttenlocher, Reference Huttenlocher1984; Miller et al., Reference Miller, Duka, Stimpson, Schapiro, Baze, McArthur and Sherwood2012) in response to the new, complex environment. Both structural and functional brain networks evolve to highly efficient topological architectures in the first few years of life (Tymofiyeva et al., Reference Tymofiyeva, Hess, Ziv, Lee, Glass, Ferriero and Xu2013; van den Heuvel et al., Reference van den Heuvel, Kersbergen, de Reus, Keunen, Kahn, Groenendaal and Benders2015), where the structural network remains ahead and paves the way for the development of the functional network. The most dramatic topological change in early childhood is the establishment of long-range connections, which are closely related to the development of network integration (Tymofiyeva et al., Reference Tymofiyeva, Hess, Ziv, Lee, Glass, Ferriero and Xu2013). Neural circuits develop independently, but eventually merge to form an integrated connectome with topological patterns that allow for increasingly refined interactions between brain regions. The early development of the human brain network progresses from a tendency toward network segregation to network integration (Mills et al., Reference Mills, Goddings, Herting, Meuwese, Blakemore, Crone and Tamnes2016), which is supported by the ‘local to distributed’ developmental pattern (Vértes & Bullmore, Reference Vértes and Bullmore2015). Increased network integration can be regarded as a marker of brain network maturation. Environmental conditions can influence the pace of brain development as an adaptive response to match the demands of unfavorable developmental conditions (Roubinov, Meaney, & Boyce, Reference Roubinov, Meaney and Boyce2021; Teicher & Samson, Reference Teicher and Samson2016). The stressful extrauterine environment of premature birth has been linked to decreased modular segregation and an increased number of inter-module connections in 2-year-old toddlers (H. Huang et al., Reference Huang, Shu, Mishra, Jeon, Chalak, Wang and He2015), suggesting an element of accelerated network maturation. In the present context, excessive screen time may represent an unfavorable environmental condition, perhaps associated with inappropriate levels of stimulation, that disrupts typical network development and results in accelerated network integration. This could potentially undermine extended neuroplasticity, which is advantageous to cognitive development, including socio-emotional competence.
Alterations in network topology lay the groundwork for an early infrastructure that is critical to the development of the brain and has the ability to predict cognitive and behavioral outcomes in later life (Thomason et al., Reference Thomason, Brown, Dassanayake, Shastri, Marusak, Hernandez-Andrade and Romero2014). Altered network topology in infants with intrauterine growth restriction is linked to socio-emotional and adaptive behaviors at age 2 (Batalle et al., Reference Batalle, Eixarch, Figueras, Muñoz-Moreno, Bargallo, Illa and Gratacos2012). Additionally, measures of network topology can predict both internalizing and externalizing behavior (Wee et al., Reference Wee, Tuan, Broekman, Ong, Chong, Kwek and Qiu2017). These findings lend credence to the idea that network topology could be a key early indicator of socio-emotional development. In the current study, we discovered that changes in the degree of integration of the emotion processing and cognitive control networks mediate the relation between screen time in infancy and socio-emotional competence at age 7. Specifically, a higher level of screen exposure increased the degree of integration between the emotion processing and cognitive control networks. A greater degree of integration between the two main brain networks essential for socio-emotional competence suggests that increased screen exposure in infancy hastens brain network maturation, possibly as a manifestation of accelerated brain development. This outcome, in turn, was associated with elevated emotional dysregulation and lower socio-emotional competence in later childhood. We thus provide a putative biological mechanism that links screen time in infancy with emotional dysregulation and socio-emotional competence. Our longitudinal study is also the first to elucidate this link between screen time in infancy, brain network topology and later socio-emotional competence in the same cohort, unifying previous findings linking screen time to brain changes (Horowitz-Kraus & Hutton, Reference Horowitz-Kraus and Hutton2018; Hutton et al., Reference Hutton, Dudley, Horowitz-Kraus, DeWitt and Holland2020) and to socio-emotional competence individually (del Pozo-Cruz et al., Reference del Pozo-Cruz, Perales, Parker, Lonsdale, Noetel, Hesketh and Sanders2019; Kerai et al., Reference Kerai, Almas, Guhn, Forer and Oberle2022; Liu et al., Reference Liu, Wu, Huang, Yan, Ma, Cao and Tao2021). These results demonstrate the enduring effect of screen time exposure in infancy.
As hypothesized, parent–child reading was found to significantly moderate the association between screen time in infancy and brain network development. It is intriguing to speculate that children with greater engagement in parent–child shared reading may be protected from the adverse effects of screen exposure. This is consistent with the concept of ‘biological embedding’, whereby enriching experiences during early childhood produce a long-term impact on brain network development (Hertzman, Reference Hertzman1999). Early life experiences are known to contribute to individual differences in susceptibility and resilience for a range of physical and mental health outcomes (Gur et al., Reference Gur, Moore, Rosen, Barzilay, Roalf, Calkins and Gur2019). Our findings are highly consistent with behavioral evidence, reinforce AAP recommendations, and highlight the importance of parent–child shared reading to promote healthy brain development and potentially mitigate the effects of screen time in infancy.
We acknowledge that the impact of screen time in infancy on brain development and later behavioral outcomes is not a simple, one-dimensional phenomenon, but rather one that needs to be conceptualized at multiple levels and in a broader context. For instance, the observed longitudinal association between screen time in infancy, brain network topology, and socio-emotional competence may be partly related to high levels of screen time replacing activities that are crucial for socio-emotional development, such as parent–child interaction and engagement in physical and imaginative play (Bauer, Gilpin, & Thibodeau-Nielsen, Reference Bauer, Gilpin and Thibodeau-Nielsen2021). It is possibly the balance or imbalance of screen time relative to a variety of other activities that is ultimately responsible for the effects of screen exposure on brain development. This does not imply that the amount of time spent on screen is unimportant. Rather, the duration of exposure must be considered along with a host of other variables, including its effect on other activities.
While our longitudinal study offers a unique opportunity to investigate the link between screen time in infancy, brain network maturation, and socio-emotional competence, some limitations should be considered when interpreting our findings. One of the most significant hurdles in longitudinal research involving screen utilization is that technology development is rapidly evolving and outpacing research. In our large, prospective study, screen time data were collected between 2010 and 2014. It is possible that screen time behaviors may have shifted over this time period owing to advances in technology. The utilization of parent-reported screen time measures is also vulnerable to reporting bias and inaccurate recollection. With the advances in technology, future studies could consider using screen time tracking apps or wearable devices for a more accurate and objective measure of screen time. A second limitation is the unidimensional focus on screen time without consideration for the content and context of viewing. Future research should attempt to disaggregate the effect of media content quality on brain development and socio-emotional competence. Furthermore, while we included an environmental latent variable to model for the impact of socio-economic status and maternal education, there are other potential confounding factors possibly linked to screen time exposure that were not accounted for in this study, including paternal education, parental mental health, living and childcare arrangements. Future studies could consider probing the effect of these factors on the relation between screen time, brain changes, and socio-emotional development. Finally, we only explored quantitative measures of parent–child reading but not qualitative factors such as verbal interactivity and engagement.
Conclusion
Our study provided novel evidence that screen time in infancy contributes to enduring individual differences in topological brain restructuring and that parent–child reading may influence brain network organization and mitigate the adverse effects of screen time. We also provided evidence that supports brain network topology as a potential biological pathway that links screen time in infancy with later socio-emotional competence. This discovery represents a promising path forward for understanding the impact of screen exposure in infancy on the development of psychopathologies related to socio-emotional competence. Finally, our study addressed the increasingly pressing public health issues of children's screen-based media consumption and reading, as well as the impact these activities have on brain development.
Supplementary material
The supplementary material for this article can be found at https://doi.org/10.1017/S0033291724000084.
Data availability statement
PH and APT had full access to all data in the study and take responsibility for the integrity of the data, the accuracy of the data analysis, and the decision to submit for publication. The data that support the findings of this study is available from the corresponding author and upon request through a GUSTO data sharing protocol overseen by the study executive committee.
Acknowledgements
The GUSTO research program was established through grants NMRC/TCR/004-NUS/2008 and NMRC/TCR/012-NUHS/2014 from the Singapore National Research Foundation under the Translational and Clinical Research Flagship and grant OFLCG/MOH-000504 from the Open Fund Large Collaborative Grant Programmes and administered by the Singapore Ministry of Health's National Medical Research Council, Singapore. Current funding is provided by the Singapore Institute for Clinical Sciences and the Brain-Body Initiative Strategic Research Program, Agency for Science Technology and Research, Singapore. MJM is supported by funding from the Toxic Stress Network of the JPB Foundation, USA, and the Jacobs Foundation, Switzerland. APT is supported by funding from the NMRC Transition Award (MOH-001273-00).
Authors’ contributions
APT, PH, and MJM conceptualized the study. XZL carried out the initial literature review. MVF assisted with data collection. ECL and MZLK processed the behavioral data. PH, SYC, ZMN, and ZYO processed the imaging data. APT, PH, and SYC analyzed the data and generated the results. APT, PH, and XZL wrote the paper with subsequent editing from MJM. MJM, PDG, and CYS obtained the funding for this research.
Competing interests
The authors declare the following financial interests/personal relationships which may be considered as potential competing interests: Yap-Seng Chong is part of an academic consortium that has received research funding from Abbott Nutrition, Nestec, and Danone. All other authors report no financial relationships with commercial interests.