Introduction
The spicules of male nematodes rank as perhaps their best-known defining features, critically important in the taxonomy of the phylum Nematoda and valuable in distinguishing between genera in the phylum (Crofton, Reference Crofton1966; Kaufmann, Reference Kaufmann1996), as well as in the identification of individual species (Lichtenfels et al., Reference Lichtenfels, Pilitt and Le Jambre1988, Reference Lichtenfels, Pilitt and Hoberg1994). Nematode spicules can be easily studied because when the worms are cleared by appropriate media such as lactic acid, in preparation for microscopical examination, their spicules remain distinct and visible against the translucent background tissues of the worms. Hence, they can be assessed and measured easily and accurately, particularly using currently available digital photography and associated software. Although not all nematode genera possess spicules (e.g. Aspiculuris spp. and Myolaimus spp.), in those that do, spicules may vary between species in length and shape, and whether they are present as a pair or just as a single spicule. In species that have a pair of spicules, both may be of identical length and shape, and hence symmetrical, or radically different from one another in both respects (Högger and Bird, Reference Högger and Bird1974; Lichtenfels et al., Reference Lichtenfels, Pilitt and Le Jambre1988; Smales et al., Reference Smales, Harris and Behnke2009) and may even be atypical and malformed in some individual worms (Andrews, Reference Andrews1970; Roy and Beveridge, Reference Roy and Beveridge1997). Relatively few studies have explored the chemical composition of nematode spicules, but unlike the calcareous spicules of other invertebrate phyla (Kingsley, Reference Kingsley1984), those of nematodes are thought not to be comprised of calcium as their structural element. Instead, nematode spicules have been shown to consist of a sclerotized protein outer layer containing keratin and collagen, and protoplasmic inner layer with carbohydrates and lipids (Stringfellow, Reference Stringfellow1971; Clark and Shepherd, Reference Clark and Shepherd1977; Sood and Kaur, Reference Sood and Kaur1983). Spicules are innervated and have sensory functions (Lee, Reference Lee1973; Wang and Chen, Reference Wang and Chen1985; Emmons, Reference Emmons2008). During copulation, spicules are used to dilate the vulva of female worms against the inner hydrostatic pressure in the pseudocoelomic cavity, ensure subsequent attachment to the females, and then to facilitate transfer of the amoeboid spermatozoa (Barr and Garcia, Reference Barr and Garcia2006).
There has been a long debate about whether the dominant trichostrongyloid intestinal nematode Heligmosomoides polygyrus (Dujardin, Reference Dujardin1845) of the Palearctic wood and yellow-necked mice (Apodemus sylvaticus and A. flavicollis, respectively) and the morphologically similar, laboratory-maintained model species H. bakeri (both previously referred to in different papers as Nematospiroides dubius and Heligmosomoides polygyrus) are the same species or whether they are distinct species in their own right. The history of the isolation of the latter was documented in some detail by Behnke et al. (Reference Behnke, Keymer and Lewis1991, Reference Behnke, Menge and Noyes2009) and Behnke and Harris (Reference Behnke and Harris2010), who also argued that based on accepted nomenclature rules, priority for the name H. polygyrus is with the naturally occurring parasite in Palearctic Apodemus spp. Evidence has been provided for some morphological differences between H. polygyrus and H. bakeri (Durette-Desset et al., Reference Durette-Desset, Kinsella and Forrester1972; Behnke et al., Reference Behnke, Keymer and Lewis1991), for differences in protein synthesis (Abu-Madi et al., Reference Abu-Madi, Reid, Lewis and Hominick1994a), host specificity (Quinnell et al., Reference Quinnell, Behnke and Keymer1991), genomic differences (Abu-Madi et al., Reference Abu-Madi, Pleass and Lewis1994b, Reference Abu-Madi, Mohd-Zain, Lewis and Reid2000) and for base-pair sequence differences in nuclear and mitochondrial genes (Zaleśny et al., Reference Zaleśny, Hildebrand, Paziewska-Harris, Behnke and Harris2014; Harris et al., Reference Harris, Zaleśny, Hildebrand, Paziewska-Harris, Behnke, Tkach, Hwang and Kinsella2015). The laboratory-maintained species is H. bakeri, having been classified earlier as a subspecies (H. polygyrus bakeri) by Durette-Desset et al., (Reference Durette-Desset, Kinsella and Forrester1972) based on morphology and then raised to species level by Cable et al. (Reference Cable, Harris, Lewis and Behnke2006) following molecular phylogenetic analysis of key genes, including the ribosomal DNA internal transcribed spacers (ITS) and the mitochondrial cytochrome c oxidase I (COI) gene. Despite arguments encouraging acceptance of their separate species status (Behnke et al., Reference Behnke, Menge and Noyes2009; Behnke and Harris, Reference Behnke and Harris2010), this has been strongly disputed (Maizels et al., Reference Maizels, Hewitson and Gause2011). However, recently, the genomes of both H. polygyrus and H. bakeri have been sequenced (Stevens et al., Reference Stevens, Martinez-Ugalde, King, Wagah, Absolon, Bancroft, Gonzalez de la Rosa, Hall, Kieninger, Kloch, Pelan, Robertson, Pedersen, Abreu-Goodger, Buck and Blaxterunder review), and it is now clear that indeed their genomes differ sufficiently for them to be unequivocally regarded as 2 distinct species, with a common ancestor estimated at 1.1–6.4 million years ago (assuming an average of six generations and one generation per year, respectively; Stevens, pers. com.).
Some earlier work has indicated that the spicules of H. polygyrus are longer than those of H. bakeri (e.g. compare data in Genov and Jančev (Reference Genov and Jančev1981) for H. polygyrus with Durette-Desset et al. (Reference Durette-Desset, Kinsella and Forrester1972) for H. bakeri). In view of the importance of spicules in the mating behaviour of nematodes, and the likelihood that differences in spicule length may contribute to the reproductive separation of species, the aim of the current work was to assess quantitatively the range of variation in spicule lengths in each of these 2 species. For this purpose, the spicule lengths of laboratory-passaged H. bakeri were compared with those of H. polygyrus from wood mice that had been sampled in different parts of the UK and on a more limited scale from other European locations, as well as with isolates of another recognized Heligmosomoides species.
Materials and methods
Sources of worms for measurement
This work is based on a range of isolates of Helgmosomoides spp., some derived from our own fieldwork in different localities across the British Isles and others provided by collaborators in other institutions abroad. Heligmosomoides bakeri worms of the strain referred to hereafter as the Australian strain were obtained from Carolyn Behm in 2012. This strain was acquired from Colin Dobson in the 1980s (C. Behm, pers. com.), who originally obtained the strain from the Wellcome Foundation Research Laboratories in London during his PhD studentship at Sheffield University in the UK (Dobson, Reference Dobson1961). The strain hereafter referred to as the Canadian strain was obtained from Marilyn Scott in 2012 and acquired originally from Mike Sukhdeo in the late 1980s (M. Scott, pers. com.). Mike Sukhdeo in turn had obtained infective larvae from John Wetzel of the Ayerst Research Labs New York (Sukhdeo et al., Reference Sukhdeo, O'Grady and Hsu1984). Although earlier ancestry of this line is unknown, it is most likely to have originated either from the Wellcome Foundation or from Spurlock in the USA (Spurlock, Reference Spurlock1943). The worms referred to hereafter as the USA strain were from Debbie Kristan whose cultures at the time (2012) were derived from 2 sources: from Mike Sukhdeo (as above) and from Nedim Ince and Joseph Urban (Beltsville Human Nutrition Research Center, USA). The Beltsville strain was obtained from Lis Eriksen at the Royal Veterinary and Agricultural University in Copenhagen around 1983. Although uncertain, it is likely that this isolate also came originally from the Wellcome Foundation. The Nottingham strain was acquired in 1975 directly from the Wellcome Foundation. It was first maintained for 2 years at Glasgow University and thereafter at Nottingham University until 2014, without any enrichment from other lineages of this species.
The worms and their spicules were measured in 3 separate sessions over 3 successive years (2012, 2013 and 2014), and accordingly, we refer below to Sessions 1–3. The available background details for each batch of worms are given in Table S1. All the material used here had been frozen at −80°C prior to measurement of the spicules, although the duration of freezing was variable, as were also the preservatives used for fixation, the duration of fixation and storage at −80°C (Table S1). The oldest sample was from Australian wild house mice, originally isolated in 1982 and preserved in formalin at room temperature for over 30 years. This isolate was sent to Nottingham in 2012 when it was transferred to 70% ethanol and frozen a day after arrival. Measurements were carried out in the period 2012–2014.
Procedures used for measurement of spicules
After thawing, individual worms were carefully placed onto a glass slide using fine watch-makers forceps and cleared with 1–2 drops of lactic acid. The worms that had been preserved in formalin took a longer time to clear. The slide was then examined in an Olympus Light Microscope and photomicrographed at × 20, using a high-resolution optic camera and saved in digital format. The worms were measured on photographs using the Image J application, each spicule being measured 4 times and averaged. Each batch of photographed worms was also accompanied by a photograph of a calibration slide (1 mm), and the measurement of this was used to convert the number of counted pixels on photographs of worms to the corresponding length in millimetres. Since there is no consistent difference between each of the 2 spicules of individual worms of these species, and variation is only minimal, in the range 0.36–6.86% (Musah-Eroje et al., Reference Musah-Eroje, Burton and Behnke2021a), we treated each spicule as an independent datum. Spicule lengths are given as mean ± standard error of the mean (s.e.m.). Measurements are in millimetres (mm) and some instances in micrometres (μm), as stated.
Statistical analysis
Where possible all datasets were tested for goodness of fit to the Gaussian distribution using bespoke software in Excel based on Elliott (Reference Elliott1977). We also calculated the index of dispersion (I = variance/mean ratio) and the index of discrepancy (D; Poulin, Reference Poulin1993) as additional guides for the pattern of distribution of the data. Where relevant we fitted GLM models in IBM SPSS version 28 (1 New Orchard Road, Armonk, New York 10504-1722, USA) and tested residuals for goodness of fit to the Gaussian distribution. Since some datasets were limited numerically, and a Gaussian distribution could not be tested for, nor assumed, analysis in these cases was based on non-parametric models (Mann–Whitney U test and Kruskal–Wallis test for which the test statistic is H).
Results
Spicule length of Heligmosomoides bakeri in mice maintained in Nottingham
The mean lengths of spicules and associated summary statistics, recorded from the H. bakeri strain, maintained in Nottingham over the 3 sessions are summarized in Table 1. The overall mean spicule length was 0.518 ± 0.0011 (n = 494). Despite the very similar mean values obtained in the 3 sessions, differing only by 9 μm, there was a significant difference between these 3 means (F 2, 491 = 5.416, P = 0.005), although it only accounted for 1.8% of the variance. The relatively low value obtained in Session 1, differing from that obtained in Session 2 (Tukey's HSD MRT, P = 0.003), arose through some of the lower values derived from the 11-day-old worms. The distribution of spicule lengths, in 100 μm length classes, differed significantly from that expected of a Gaussian distribution (Fig. 1a; χ 211 = 33.6, P < 0.001), although the values of both I and D indicated this distribution model as the most appropriate (Fig. 1a).
Table 1. Summary statistics for length of spicules of Heligmosomoides bakeri

a These 21-day-old worms were from the same dataset as that reported for this age class by Musah-Eroje et al. (Reference Musah-Eroje, Burton and Behnke2021a).
b Age was not recorded.
c These 21-day-old worms were from different batches measured on different occasions.
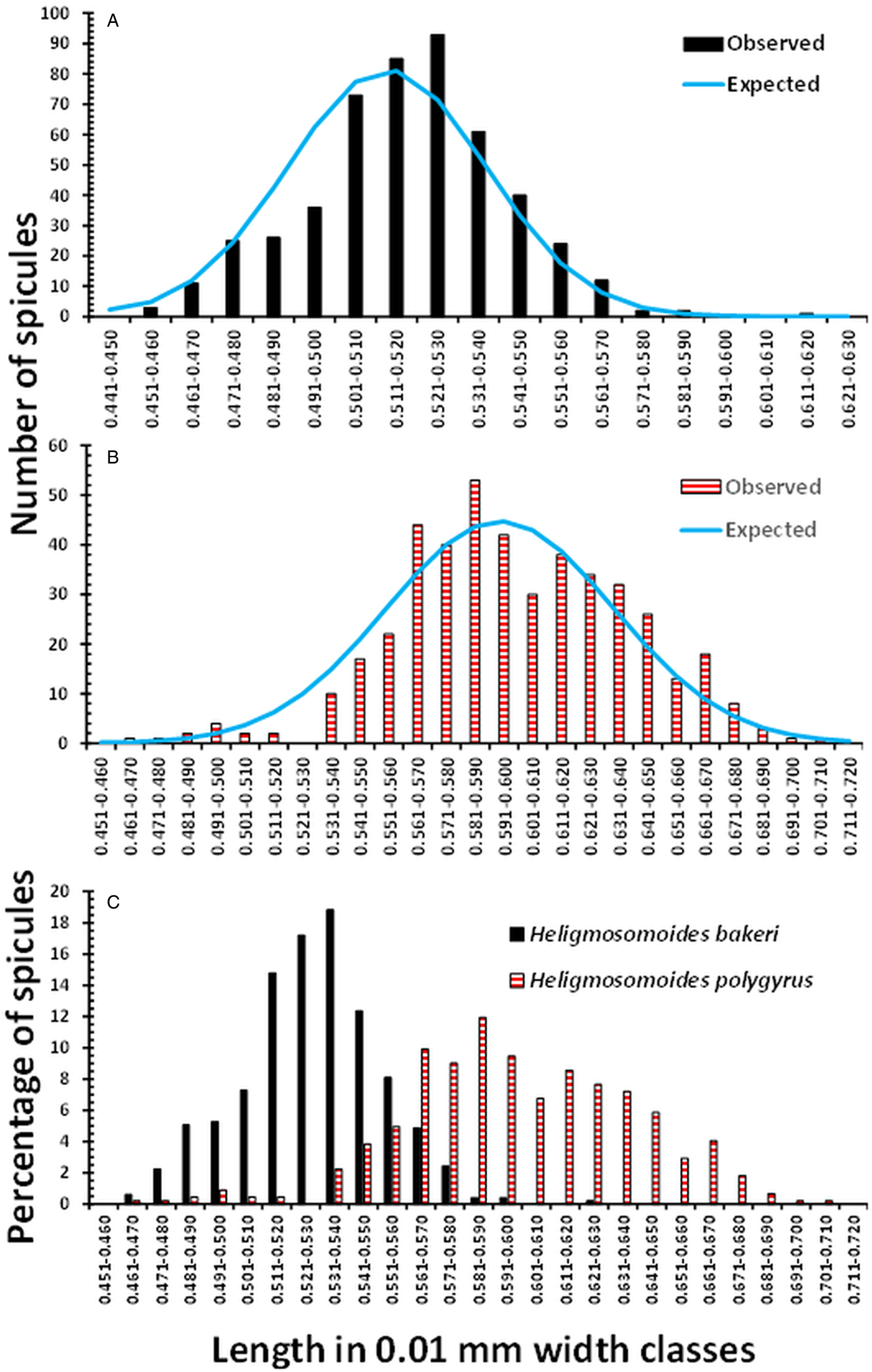
Figure 1. Frequency distribution of the lengths of spicules of H. bakeri (Nottingham strain) and H. polygyrus (isolates from the British Isles). (a) Observed values of spicule lengths of H. bakeri worms from all 3 sessions (n = 494, I = 0.001, and D = 0.183) and those expected of the Gaussian distribution. (b) Observed values of spicule lengths of H. polygyrus from 11 localities in the British Isles (combined data, n = 444, I = 0.003, and D = 0.154) and those expected of the Gaussian distribution. (c) Comparison of the distribution of the lengths of spicules of H. bakeri and H. polygyrus expressed as the percentage of the total of each species, to compensate for the difference in sample sizes.
Spicule length of Heligmosomoides polygyrus from different locations in the British Isles
The mean lengths and associated summary statistics for spicules of worms measured from wood mice trapped in eleven localities in the British Isles are shown in Table 2. The overall mean length of spicules from worms from all 11 sites combined was 0.598 ± 0.0019 mm (n = 444), with a range from 0.465 to 0.710 mm, and a distribution that differed significantly from that expected of the Gaussian distribution (Fig. 1b; χ 218 = 40.7, P = 0.002). However, as we found with H. bakeri (above), the values of both I and D (Fig. 1) indicated that a normally distributed pattern was appropriate.
Table 2. Summary statistics for lengths of spicules of Heligmosomoides polygyrus from Apodemus sylvaticus sampled in various counties in the British Isles

a Theses isolates were from wood mice caught in several locations in Mid Ireland as follows: Rhode, Offally; Vicarstown, Laois; Brackagh, Offally; Slieve Bloom Mts, Laois.
Because of limited sample sizes from some locations, only the spicule lengths of worms from Norfolk (χ 23 = 2.53, P = 0.47), Staffordshire (χ 27 = 6.64, P = 0.47), Dorset (χ 27 = 10.59, P = 0.158) and Nottinghamshire (χ 211 = 21.10, P = 0.032) could be tested for goodness of fit to the Gaussian distribution. While the first 3 showed good concordance to the Gaussian distribution, the spicule lengths of worms from mice trapped in Nottinghamshire deviated significantly. However, in all 4 cases, the values of I (0.002, 0.001, 0.003 and 0.003, respectively) and D (0.244, 0.232, 0.201 and 0.166, respectively) indicated that the Gaussian distribution was acceptable. The lowest mean value was for the spicules of mice from Dorset recorded in Session 1, although the mean value obtained in Session 2 was higher (Table 2). The longest mean spicule length was for the spicules of the mice from Mid. Ireland, representing a 12.6% (71 μm) increase in mean length, relative to the value for spicules from Dorset mice recorded in Session 1. The difference in spicule lengths between the eleven isolates was significant (F 10, 443 = 11.233, P < 0.001), accounting for 18.8% of the variance in this model.
Comparison of the length of spicules of H. bakeri (Nottingham lab strain) and H. polygyrus from sites in the British Isles
Figure 1c compares the distribution of the spicule lengths of H. bakeri and H. polygyrus, expressed in percentage terms because of the difference in sample sizes. The distribution of H. bakeri spicule lengths spanned a more limited range than that of H. polygyrus (variance = 569 × 10−6 and 1569 × 10−6, respectively), as might be expected from a laboratory-maintained isolate of known age, compared to the wild, naturally breeding H. polygyrus isolates of unknown age. Although there was some overlap of lengths, the majority of spicules from H. polygrus were longer than those recorded from H. bakeri. We recorded 1 outlier among the H. bakeri from Nottingham, at 0.611 mm, and 2 with lengths of 0.589 and 0.580 mm in Session 3, but otherwise, the longest spicule was 0.577 mm. Consistent with the fitted Gaussian bell-shaped curve in Fig. 1a, taking this value as the longest reliable H. bakeri spicule, 69.4% of the spicules from H. polygyrus exceeded this value. Moreover, 97.7% of spicules were longer than the mean value of H. bakeri spicules (0.519 mm), and standard deviations (s.d.) did not overlap (for H. bakeri mean + 1 s.d. = 0.541 mm and for H. polygurus, mean – 1 s.d. = 0.559 mm). There was a highly significant difference between the lengths of H. bakeri spicules (Nottingham laboratory strain) and those from British Isles isolates of H. polygyrus (F 1, 936 = 1467.6, P < 2.2 × 10−16), accounting for 61.0% of the variance in this model.
Spicule length of Heligmosomoides bakeri in mice maintained in other laboratories
Table 1 also summarizes the results of measurements of the spicules of H. bakeri maintained in laboratory mice in 3 other laboratories, 1 in each of Australia, Canada, and the USA. The spicules from worms from the laboratory in California were measured separately in 2 sessions, but the means only differed by 7 μm (Table 1), and the difference was not significant (F 1, 38 = 2.18, P = 0.15). We compared the data for spicules from all 4 laboratories (with sessions combined), and there was a significant difference between the 4 laboratories (F 3, 572 = 4.094, P = 0.007), although it only accounted for 1.6% of the variance. The mean values for worms from the other 3 laboratories were all higher than that for the worms from Nottingham, but significance was primarily attributable to the difference in mean values between the shortest (Nottingham) and the longest (Canada) which was only 22 μm (post hoc comparison between labs, Tukey's HSD, P = 0.014). However, when combined, the mean length of the spicules from the other laboratories was numerically 1.36% longer, their combined mean differing by 7 μm from the combined Nottingham mean (Table 1). This difference was significant (F 1, 574 = 6.371, P = 0.012) although it only accounted for 0.9% of the variance.
Spicule length of Heligmosomoides spp. from different locations abroad
Table 3 shows summary statistics for spicule lengths of a range of other isolates that we were able to incorporate into this study. These include H. bakeri recovered from wild house mice from Australia in 1982 and H. polygyrus isolates from wood mice from Portugal and Norway, and from yellow-necked mice from Italy.
Table 3. Summary statistics for lengths of spicules of Heligmosomoides spp. from Mus musculus and Apodemus spp. from various locations abroad and from Myodes glareolus from Anglesey in Wales

a The spicules from worms of these isolates were from wood mice from the island of Anglesey in Wales.
The mean value of the spicules from Australian wild house mice was the shortest in our study. The 2 means, from Sessions 1 and 2, differed marginally (F 1, 36 = 4.642, P = 0.038) and in both sessions were low even relative to our combined value for H. bakeri (0.519 mm). The combined mean value for these spicules (0.480 ± 0.0055) was evidently smaller than any of the means of the other datasets in Table 1 (e.g. 7.46% shorter than the mean for the Nottingham datasets combined).
Spicule lengths of worms from Italy, Portugal and Norway differed significantly (H 2 = 48.8, P < 0.001). The longest H. polygyrus spicules were recorded for worms from Norwegian wood mice and the shortest for worms from Portuguese wood mice (especially those measured in Session 1). The difference between the spicule lengths of worms from these 2 countries was highly significant (U 80, 20 = 1561.0, P < 0.001, and differing in mean length by 100.8 μm). The spicules of worms from Italy were also significantly longer than those from Portugal (post hoc test with Bonferroni correction for multiple tests, P = 0.044, but differing in mean length by only 10.2 μm). However, all the mean values for the spicules of H. polygyrus among these continental isolates, and their combined value, (Table 3) were longer than that for our combined value for the laboratory-maintained strain of H. bakeri (overall mean differing by 70.9 μm, F 1,712 = 603.2, P < 2.2 × 10−16, R 2 = 0.458). Moreover, they were mostly similar to those of H. polygyrus from wood mice from localities in the British Isles, the overall means [combined value for worms from the British Isles (Table 2) vs that for combined value for worms from Norway, Portugal and Italy (Table 3)] differing by only 8.9 μm, albeit significantly (F 1,580 = 4.7, P = 0.031 and R 2 = 0.006).
Spicule length of Heligmosomoides glareoli from bank voles
We had a limited opportunity to measure the spicules of another species of Heligmosomoides, constrained by a small sample size. The summary stats are given in Table 3 and show that as expected the spicules of H. glareoli were almost twice as long as those of H. bakeri, as documented in the literature (combined mean = 1.098 ± 0.0071, n = 68). The 3 means obtained in the different sessions (Table 3) all exceeded 1 mm, but differed significantly (F 2, 65 = 8.028, P < 0.001), the model explaining 17.3% of the variance. The difference between sessions was attributable to the slightly longer measurements recorded in Session 3.
Discussion
The principal aim of the current work was to determine whether the lengths of the spicules of H. bakeri differ from those of H. polygyrus. Given the crucial function of nematode spicules in mating activity, a significant difference between the spicule lengths of these 2 species could lead to reproductive separation and isolation, and hence explain their separate species status. Indeed, our analysis revealed a clear significant difference between the spicule lengths of the Nottingham-maintained strain of H. bakeri and the pooled value of spicule lengths of worms from wood mice sampled in 11 different localities in the British Isles.
The data provided in this report for spicule lengths of the Heligmosomoides spp. that we measured and variation in these measurements, based on quantitative measurements (overall a total of 1264 spicules were measured) and associated analysis, contrast with information available in the public domain. For the most part, data in the literature either provide a mean spicule length or a range for a given species (Lichtenfels et al., Reference Lichtenfels, Pilitt and Hoberg1994) and without any information on how many spicules were measured. Thus, the extent of variation within the populations that were sampled has not always been taken into account.
The mean value we obtained for H. bakeri (0.519 mm) fits well with the range given by Durette-Desset et al. (Reference Durette-Desset, Kinsella and Forrester1972; 0.420–0.520 mm), although in our case the range was wider (0.451–0.611 mm). However, as pointed out earlier, the highest value we recorded (0.611 mm) was very much an outlier, and 0.577 mm would seem to be a more reliable upper limit. In comparison, the mean spicule length that we recorded for H. polygyrus isolates from wood mice from the British Isles was 0.598 mm. This value is very close to the 0.58 mm given by Dujardin (Reference Dujardin1845) for the spicules of Strongylus polygyrus ( = H. polygyrus) in the original description of this species and fits the range (0.54–0.6 mm) given by Baylis (Reference Baylis1928) for worms (which he referred to as Nematospiroides dubius) from wood mice from a locality near Oxford. Our results are also compatible with the range (0.516–0.620 mm) given by Genov and Jančev (Reference Genov and Jančev1981) and Genov (Reference Genov1984), and the mean provided by Schulz (Reference Schulz1926; 0.608 mm). As with H. bakeri, in our study, the recorded range of spicule lengths for H. polygyrus was wider (0.565–0.710 mm) than reported in these publications.
The smaller variance of spicule lengths of H. bakeri compared with H. polygyrus is to be expected, given that this species has been passaged in laboratory mice for over 60 years. Ehrenford (Reference Ehrenford1954) isolated the species from wild Peromyscus maniculatus in California in 1950 and established a laboratory line (strain 50) which Spurlock then distributed to various laboratories including the Wellcome Foundation in London (Behnke et al., Reference Behnke, Keymer and Lewis1991). The strain maintained in Nottingham was obtained from the Wellcome Foundation in 1975 and has been passaged routinely since then, initially at Glasgow University, and from 1976 at Nottingham University in CFLP strain mice. Given the long period of laboratory maintenance, a degree of inbreeding and hence loss of genetic diversity might be expected. However, there is evidence for some degree of genetic variation within laboratory-maintained strains of H. bakeri because lines varying in their sensitivity to the host response, and capacity to cause chronic infections, can be created by selective breeding (Dobson and Tang, Reference Dobson and Tang1991; Tang et al., Reference Tang, Dobson and McManus1995). Chehresa et al. (Reference Chehresa, Beech and Scott M1997) found that separate passage of lines without mixing, resulted within 10 generations in distinct lines differing in aspects of their life histories, while Njoroge et al. (Reference Njoroge, Scott and Jalili1997) reported selection of lines for enhanced resistance to the anthelmintic drug ivermectin. Moreover, the recent whole genome sequencing of H. bakeri has confirmed that there is indeed an unexpectedly high degree of genetic diversity in the Edinburgh strain (acquired from Nottingham in the 1990s; Hewitson et al., Reference Hewitson, Filbey, Grainger, Dowle, Pearson, Murray, Harcus and Maizels2011) used for this work (Stevens et al., Reference Stevens, Martinez-Ugalde, King, Wagah, Absolon, Bancroft, Gonzalez de la Rosa, Hall, Kieninger, Kloch, Pelan, Robertson, Pedersen, Abreu-Goodger, Buck and Blaxterunder review). On this basis, it is perhaps not surprising that our analysis of spicule lengths of worms maintained in 4 laboratories, located on 3 continents, revealed significant variation between these strains, especially between the Nottingham and Canadian strains.
Our analysis showed that there was some variation in the spicule lengths of H. polygyrus from different regions of the UK and also between worms from hosts from 3 European countries. Variation in nematode morphology has been attributed to various factors in the past, including the age of nematodes (Bryant Reference Bryant1973). In preparation for the current analysis, we followed daily changes in spicule length during the development of H. bakeri after experimental infection and found that spicule length does vary somewhat during the development and growth of the worms (Musah-Eroje et al., Reference Musah-Eroje, Burton and Behnke2021a). Spicules are first detectable by microscopy on day 6 post infection (PI) when the worms are at a late L4 stage of development and moulting to the adult stage. Their length then increases to peak on day 7 PI before contracting by approximately 0.1 mm (from day 7 peak means of 0.59 and 0.61 mm in 2 experiments) until they stabilize by 14 days PI (0.51 and 0.53 mm; Musah-Eroje et al., Reference Musah-Eroje, Burton and Behnke2021a). Whilst it was not possible for ethical reasons to repeat such experiments in wood mice infected with H. polygyrus, the development of worms in this host/parasite combination is unlikely to differ radically. It is conceivable therefore that some of the shorter H. polygyrus spicules measured in the current work, whose length overlapped with the longer H. bakeri spicules, may have been from recently moulted adult worms. Nevertheless, given the longevity of both H. bakeri and H. polygyrus (Robinson et al., Reference Robinson, Wahid, Behnke and Gilbert1989; Gregory et al., Reference Gregory, Keymer and Clarke1990), and the known accumulation of worms with increasing host age (Elton et al., Reference Elton, Ford, Baker and Gardiner1931; Behnke et al., Reference Behnke, Lewis, Mohd Zain and Gilbert1999), the probability of selecting such worms in large scale sampling, as in the current work, is likely to be very low, although not improbable.
The H. polygyrus worms utilized in the current study were from wood mice trapped during fieldwork in various locations over a period of many years and had been preserved mostly in 80% ethanol and frozen at −80°C. It is possible that some of the variation detected in spicule length was attributable to differences arising from the use of different preservatives and associated variation in storage time at −80°C. In an earlier paper, we investigated how the use of standard preservatives might affect spicule length (Musah-Eroje et al., Reference Musah-Eroje, Burton and Behnke2021b) and concluded that the spicules of H. bakeri are robust and change very little in preservatives over time. The maximum change was 5.03% shrinkage in Hanks' balanced saline combined with freezing at −80°C, but shrinkage/expansion in other combinations of preservatives with storage at room temperature or freezing was less. The maximum period of storage in these experiments was 4 months, and it is conceivable that longer periods of storage may have generated greater shrinkage or expansion of spicule length. The H. bakeri worms from Australian wild M. domesticus, measured in the current work, had shorter spicules than the laboratory-maintained H. bakeri, but these worms had been stored in formalin for over 40 years, which may have accounted for the difference. Nevertheless, in our experiments storage in formalin, whether at room temperature or at −80° C, resulted in less than 3% change in length, albeit over periods of only 1 and 4 months.
Among samples from the British Isles, the longest spicules of H. polygyrus were from wood mice from Scotland and Mid. Ireland, but the longest of all were from abroad in Norway, perhaps suggesting a latitudinal trend for longer spicules with more northerly latitudes. Some degree of between-locality variation is to be expected, as wood mouse populations across the UK and Europe are not entirely panmictic, with many local barriers to gene flow across the region, including rivers, cities, farmland and even the North Sea in the case of Norway. In W. Europe, H. polygyrus populations are known to consist of at least 5 distinct genetic variants represented by 5 clades based on differences in the mitochondrial cyt b gene (Nieberding et al., Reference Nieberding, Libois, Douady, Morand and Michaux2005, Reference Nieberding, Durette-Desset, Vanderpoorten, Casanova, Ribas, Deffontaine, Feliu, Morand, Libois and Michaux2008). Although worms from the United Kingdom were not included in these studies, those from Ireland and Denmark formed Clade 5, which differed significantly from Clade 1, comprising worms from Italy, and Clade 2, those from the Iberian Peninsula. It is therefore possible that to some extent the differences that we found in spicule length between isolates of H. polygyrus from localities in the British Isles, as well as between the isolates from the 3 European countries, were attributable to morphological differences between these clades, although the latter were not explored in the work of Nieberding et al. (Reference Nieberding, Libois, Douady, Morand and Michaux2005).
Both the mean value that we obtained for the spicule length of H. glareoli (1.098 mm) and the range (0.957–1.260 mm) fit well the ranges given by Baylis (Reference Baylis1928; 0.8–1.0 mm), Genov and Jančev (Reference Genov and Jančev1981), Genov (Reference Genov1984; 0.984–1.179 mm), and Biserkov et al. (Reference Biserkov, Genov and Hadjinikolova1998; 0.87–1.0 mm) and confirm that the spicule length of this species is almost twice those of H. bakeri and H. polygyrus.
In conclusion, in this paper, we have provided summary data (mean lengths and extent of variation in lengths) based on quantitative measurements of the spicules of male H. polygyrus (n = 582), and H. bakeri (n = 614) as well as H. glareoli (n = 68). While H. polygyrus and H. bakeri are morphologically very similar and cannot be readily distinguished by conventional microscopy, the spicules of male worms of these species differ significantly in length with only minimal overlap in measurements when large sample sizes are assessed. Although we cannot exclude entirely an environmental effect on spicule length (H. bakeri develop in Mus spp. while H. polygyrus in Apodemus spp., but see Quinnell et al., Reference Quinnell, Behnke and Keymer1991), we nevertheless consider that our results are compatible with other recorded differences between these isolates (see above) and especially the recent genomic data indicative of separate species status (Stevens et al., Reference Stevens, Martinez-Ugalde, King, Wagah, Absolon, Bancroft, Gonzalez de la Rosa, Hall, Kieninger, Kloch, Pelan, Robertson, Pedersen, Abreu-Goodger, Buck and Blaxterunder review). Since spicules play a vital role in reproduction, this difference in spicule length between the species therefore helps to consolidate evidence that indeed H. bakeri and H. polygyrus are 2 distinct species.
Supplementary material
The supplementary material for this article can be found at https://doi.org/10.1017/S0031182023000872.
Data availability statement
All the data analysed in the current paper can be made available on request to JMB.
Acknowledgements
We thank the following collaborators for provision of isolates for the current work: Marilyn Scott and Lisa Starr (McGill University, Montreal, Canada), Dave Spratt (CSIRO Ecosystem Sciences, Canberra, Australia), Carolyn Behm (Australian National University), Debbie Kristan (California State University, USA), Ian Montgomery (Queen's University, Belfast, N. Ireland), Les Chappell (University of Aberdeen, UK), Emma Gillingham and Sarah Perkins (University of Cardiff). We are grateful to Francis Gilbert, Phil Harris and Mike Kinsella for their insightful comments and advice on earlier versions of the manuscript for this paper.
Author's contributions
The project was conceived and designed by JMB. The laboratory work was carried out by LB, NK and MME. Statistical analysis was done by JMB. The article was written by J.M.B. and refined by contributions from all authors. All authors approved the final version of the manuscript.
Financial support
This research received no specific grant from any funding agency, commercial or not-for-profit sectors.
Competing interests
None.
Ethical standards
All animal procedures were carried out under UK Home Office licence number 40/3138 and under the regulations of the Animals (Scientific Procedures) Act 1986. Maintenance of animals conformed to local and Home Office Code of Practice (ISBN 9781474112390).