Introduction
Notosuchia is a group of extinct, mostly terrestrial crocodyliforms. The presence of several morphological features suggests that they had an erect posture: the prominent crest overhanging the acetabulum observed in Chimaerasuchus paradoxus (Wu and Sues Reference Wu and Sues1996), Notosuchus terrestris (Pol Reference Pol2005), Araripesuchus tsangatsangana (Turner Reference Turner2006), Baurusuchus albertoi (Nascimento and Zaher Reference Nascimento and Zaher2010), and Stratiotosuchus maxhechti (Riff and Kellner Reference Riff and Kellner2011); the straight-shafted long bones described in Anatosuchus minor and Araripesuchus spp. (Sereno and Larsson Reference Sereno and Larsson2009); the slender limb bones with muscular insertions close to the joints reported in Malawisuchus mwakasyungutiensis (Gomani Reference Gomani1997); and the tight/stable knee joint shown in Pissarrachampsa sera (Godoy et al. Reference Godoy, Bronzati, Eltink, Marsola, Cidade, Langer and Montefeltro2016). Among extant tetrapods, only endotherms (mammals and birds) show an upright stance. This last feature has been proposed to be linked to endothermy, because it contributes to the efficiency of the respiratory system (Carrier Reference Carrier1987). Thus, according to this morphological evidence, we can reasonably hypothesize that Notosuchia were endothermic. However, Cubo et al. (Reference Cubo, Sena, Aubier, Houee, Claisse, Faure-Brac, Allain, Andrade, Sayão and Oliveira2020) concluded that they were primitively ectothermic using two proxies: resting metabolic rate (RMR) and red blood cell size (RBCsize).
RMR is the minimal consumption of oxygen over time per unit of body mass measured under postabsorptive conditions during the period of normal activity of the daily cycle in resting, nonreproductive specimens (Andrews and Pough Reference Andrews and Pough1985; Montes et al. Reference Montes, Le Roy, Perret, de Buffrénil, Castanet and Cubo2007). RMRs of extant endotherms are at least one order of magnitude higher than those of extant ectotherms of similar body mass, because the mechanisms of thermogenesis operating in the former are costly in terms of energy (Clarke and Pörtner Reference Clarke and Pörtner2010; Legendre and Davesne Reference Legendre and Davesne2020). RMRs inferred by Cubo et al. (Reference Cubo, Sena, Aubier, Houee, Claisse, Faure-Brac, Allain, Andrade, Sayão and Oliveira2020) for Notosuchia were significantly lower than the threshold separating ectotherms from endotherms.
Within extant tetrapods, RBCsize is lower in endotherms (mammals and birds) than in ectotherms (Amphibia, Squamata, Testudines, and Crocodylia) (Hartman and Lessler Reference Hartman and Lessler1964; Snyder and Sheafor Reference Snyder and Sheafor1999; Soslau Reference Soslau2020). It has been suggested that the acquisition of lungs together with the subsequent evolution of the cardiovascular system was the driving force explaining the evolution of vertebrate RBCsize (Snyder and Sheafor Reference Snyder and Sheafor1999). In endotherms, thermogenetic mechanisms use a huge amount of oxygen, producing high RMRs. Considering that “Smaller capillaries [and smaller RBCs] are associated with increased potential for diffusive gas exchange” (Snyder and Sheafor Reference Snyder and Sheafor1999: 189), these features may have been positively selected in endotherms. Huttenlocker and Farmer (Reference Huttenlocker and Farmer2017) found that RBCsize values are related to, and can be inferred from, bone vascular canal diameter. Cubo et al. (Reference Cubo, Sena, Aubier, Houee, Claisse, Faure-Brac, Allain, Andrade, Sayão and Oliveira2020) inferred notosuchian RBCsize values using this last relationship and concluded that they were significantly higher than the threshold separating ectotherms from endotherms.
To sum up, both proxies (RMR and RBCsize) suggest that Notosuchia were ectothermic organisms. Considering that paleohistological evidence (suggesting low RMR, large RBCsize, and ectothermy) is not congruent with morphological evidence (suggesting an erect posture, cursoriality, and endothermy), the thermophysiological status (i.e., either ectothermic or endothermic) of Notosuchia deserves further analysis.
The approach used by Cubo et al. (Reference Cubo, Sena, Aubier, Houee, Claisse, Faure-Brac, Allain, Andrade, Sayão and Oliveira2020) to perform these inferences can be improved in two ways. First, notosuchian thermophysiological status inferred using RBCsize is based on the quoted relationship between RBCsize and bone vascular canal diameter (Cubo et al. Reference Cubo, Sena, Aubier, Houee, Claisse, Faure-Brac, Allain, Andrade, Sayão and Oliveira2020). This relationship was tested by Huttenlocker and Farmer (Reference Huttenlocker and Farmer2017) using a rather small sample size (14 extant tetrapod species). Here we tested this relationship using a more comprehensive sample of extant tetrapods (31 species) and phylogenetic generalized least-squares regression (PGLS). Second, RBCsize estimations were performed using phylogenetic eigenvector maps (PEMs), and this method excludes a fraction of phylogenetic information. This is because PEM generates a high number of eigenvectors (n − 1, with n being the number of terminal taxa analyzed), thus requiring a selection procedure to compile a subset of eigenvectors to avoid model overfitting (Guénard et al. Reference Guénard, Legendre and Peres-Neto2013; Legendre et al. Reference Legendre, Guénard, Botha-Brink and Cubo2016). Here we inferred the thermophysiology of Notosuchia using phylogenetic logistic regression (PLR) (Ives and Garland Reference Ives and Garland2010; Tung Ho and Ané Reference Tung Ho and Ané2014), a method that overcomes this problem, because it includes all (instead of a fraction) of the phylogenetic information.
Material and Methods
Phylogenies in Figure 1 and Supplementary File 1 contain the tetrapod samples used in this study. Topologies were taken from Pyron and Wiens (Reference Pyron and Wiens2011) for amphibians; Meredith et al. (Reference Meredith, Janečka, Gatesy, Ryder, Fisher, Teeling, Goodbla, Eizirik, Simão, Stadler, Rabosky, Honeycutt, Flynn, Ingram, Steiner, Williams, Robinson, Burk-Herrick, Westerman, Ayoub, Springer and Murphy2011), Zurano et al. (Reference Zurano, Magalhães, Asato, Silva, Bidau, Mesquita and Costa2019), Kumar et al. (Reference Kumar, Hallström and Janke2013), and Upham et al. (Reference Upham, Esselstyn and Jetz2019) for mammals; Ast (Reference Ast2001) and Villa et al. (Reference Villa, Abella, Alba, Almécija, Bolet, Koufos, Knoll, Luján, Morales, Robles, Sánchez and Delfino2018) for Varanus; Man et al. (Reference Man, Yishu, Peng and Xiaobing2011) for crocodiles; Prum et al. (Reference Prum, Berv, Dornburg, Field, Townsend, Lemmon and Lemmon2015) for birds; and Pol et al. (Reference Pol, Nascimento, Carvalho, Riccomini, Pires-Domingues and Zaher2014) for Notosuchia. Both phylogenies were dated using Time Tree of Life (http://www.timetree.org). When the ages of two successive nodes collapsed, we arbitrarily added 1 Myr in between the more-inclusive and less-inclusive nodes to facilitate the graphic visualization of the topology. For Notosuchia, nodes were dated according to the last appearance datum (LAD) of the oldest fossil included in each node taken from the Paleobiology Database (https://paleobiodb.org). The age of the node Notosuchia (113 Myr) corresponds to the LAD of Malawisuchus mwakasyungutiensis. The age of the node Armadillosuchus–Baurusuchus (100.5 Myr) corresponds to the LAD of Chimaerasuchus paradoxus. The age of the node Iberosuchus–Baurusuchus (83.6 Myr) corresponds to the LAD of Comahuesuchus brachybuccalis, Pehuenchesuchus enderi, Cynodontosuchus rothi, and Wargosuchus australis. Finally, the age of the node Stratiotosuchus–Baurusuchus (66 Myr) corresponds to the LAD of these taxa. The latter (Stratiotosuchus–Baurusuchus), as well as Armadillosuchus arrudai, come from the Adamantina Formation, the age of which is still debated. We follow the hypothesis of a Campanian–Maastrichtian age proposed by some authors (e.g., Gobbo-Rodrigues et al. Reference Gobbo-Rodrigues, Petri and Bertini1999; Batezelli Reference Batezelli2017).

Figure 1. Phylogenetic relationships among extant taxa used to construct the thermophysiology inference model and the extinct Notosuchia for which we performed paleobiological inferences. Sources of topology and divergence times are cited in the main text. Scale on the right: geologic time in millions of years (Myr).
Testing the Relationship between RBCsize and Bone Vascular Canal Diameter Using PGLS
Supplementary File 1 contains the sample (31 species of extant tetrapods) and the phylogeny (topology and divergence times) used to test the relationships between the response variables (RBCwidth and RBCarea) and the explanatory variables (femoral vascular canal diameter and femoral cross-sectional area including the medullary cavity). Thin sections of extant taxa are curated at the Vertebrate Hard Tissue Collection of the Museum national d'Histoire naturelle, Paris, and are available on request to the curator (D. Germain). RBCwidth (defined as RBC minimum diameter) and RBCarea (either published values or values computed using maximum and minimum published diameters and assuming an ellipse) were taken from the literature (Supplementary File 2). Femoral vascular canal diameters (white arrowheads in Fig. 2) were computed as Canharmean and Canmin, as defined by Huttenlocker and Farmer (Reference Huttenlocker and Farmer2017). Canharmean, Canmin, and femoral cross-sectional area were either quantified in this study or taken from Huttenlocker and Farmer (Reference Huttenlocker and Farmer2017) (data available in Supplementary File 2).

Figure 2. Transverse thin section, lateral side, of the femur of Araripesuchus wegeneri Buffetaut, Reference Buffetaut1981, observed in cross-polarized light with lambda wave plate. The thin section was made from a partial femur (MNHN.F.GDF660) from the Aptian of Gadoufaoua (Niger), and is curated at the Museum national d'Histoire naturelle (MNHN) (Paris, France). The cortex is made of lamellar-zonal bone. It is composed of three zones formed at moderate growth rate and containing vascular canals (white arrowheads) included in primary osteons, and three annuli formed at low growth rates and made of parallel fibered bone (black arrowheads). Periosteum is on the top and medullary cavity on the bottom. The continuous black line occurring near the medullary cavity is an artifact. Scale bar, 0.5 mm.
The method of ordinary least-squares regression makes the assumption of no covariance between residuals obtained from the regression equation (i.e., the off-diagonals of the variance–covariance matrix are expected to contain zeros) (Symonds and Blomberg Reference Symonds, Blomberg and Garamszegi2014). In analyses using interspecific data, this assumption is not verified because of the hierarchical, shared phylogenetic history among terminal taxa (i.e., closely related species are more similar than expected by chance). PGLS (Grafen Reference Grafen1989; Martins and Hansen Reference Martins, Hansen and Martins1996; Rohlf Reference Rohlf2001; Symonds and Blomberg Reference Symonds, Blomberg and Garamszegi2014) overcomes this problem by using a variance–covariance matrix in which off-diagonals correspond to the phylogenetic history shared by the two species under comparison. Symonds and Blomberg (Reference Symonds, Blomberg and Garamszegi2014) described PGLS as a “weighted regression” in which data points corresponding to closely related species are “downweighted.” We ran PGLS using the function pgls of the package caper (Orme et al. Reference Orme, Freckleton, Thomas, Petzoldt, Fritz, Isaac and Pearse2013) in R (R Development Core Team 2008).
Inferring the Thermophysiology of Notosuchia Using PLR
Figure 1 shows the phylogenetic relationships among extant taxa (46 species of tetrapods) used to construct the thermophysiology inference model (to infer the probability of being endothermic) and the extinct Notosuchia for which we performed paleobiological inferences. This model was constructed using femoral vascular canal diameter (Canharmean and Canmin) and femoral cross-sectional area as explanatory variables. As noted earlier, femoral Canharmean, Canmin, and femoral cross-sectional area were either quantified in this study or taken from Huttenlocker and Farmer (Reference Huttenlocker and Farmer2017) (data available in Supplementary File 3). As before, thin sections of extant taxa are curated at the Vertebrate Hard Tissue Collection of the Museum national d'Histoire naturelle, Paris. Data for Notosuchia are taken from Cubo et al. (Reference Cubo, Sena, Aubier, Houee, Claisse, Faure-Brac, Allain, Andrade, Sayão and Oliveira2020): Araripesuchus wegeneri, Armadillosuchus arrudai, Baurusuchus sp., Iberosuchus macrodon, and Stratiotosuchus maxhechti. Considering that the model was constructed using femora of extant species, we performed inferences only for those Notosuchia for which data for femora were available. Sample size was smaller for PGLS analyses, because data for RBCsize were not available for many species analyzed in PLR analyses. PLR is a generalized linear model explaining the probability of occurrence of the state “presence” of a binary response (dependent) variable (here the “presence of endothermy”) using continuous explanatory (independent) variables when residual variation of the former variable is phylogenetically structured (Ives and Garland Reference Ives and Garland2010). The regression coefficients computed do account for phylogenetic correlation; when data are not phylogenetically structured, these coefficients are those of standard logistic regression (Ives and Garland Reference Ives and Garland2010). PLR models contain two components. The first is controlled by parameters α (the transition rate) and μ (the asymptotic probability of being in state 1 [here the asymptotic probability of being endotherm]). Parameter α equals α1 + α0; α1 being the probability that the response variable switches from 0 to 1 in each small time increment when it evolves up a phylogenetic tree, whereas the α0 parameter is the probability that it evolves from 1 to 0 (Ives and Garland Reference Ives and Garland2010). The transition rate α is a measure of phylogenetic signal, because the larger the α, the quicker the evolutionary transitions and the lower the phylogenetic structure of data (Ives and Garland Reference Ives and Garland2010). In the second component, the probability of occurrence of the state “presence of endothermy” is modeled using values of the independent (explanatory) variable (here bone vascular canal diameter). Parameters α and μ are estimated using an iterative process in which μ is estimated given α, using the quasi-likelihood function, and α is estimated given μ, using least squares until convergence (Ives and Garland Reference Ives and Garland2010). Analyses were performed using the package phyloglm (Tung Ho and Ané Reference Tung Ho and Ané2014) in R (R Development Core Team 2008).
Results
Testing the Relationship between RBCsize and Bone Vascular Canal Diameter Using PGLS
We used PGLS to test the relationships between RBCwidth and RBCarea and the explanatory variables femoral vascular canal diameter (computed as Canharmean and Canmin) and femoral cross-sectional area (data available in Supplementary File 2). Shapiro-Wilk normality tests showed that residuals of PGLS regression of RBCarea to Canharmean + bone cross-sectional area and the regression RBCarea to Canmin + bone cross-sectional area do not follow a normal distribution (p-values of 0.0007557 and 0.001896, respectively). Thus, we performed a log transformation of all variables. After log transformation, residuals of all four PGLS regressions (RBCarea and RBCarea to the explanatory variables bone cross-sectional area and either Canmin or Canharmean) do follow a normal distribution. All four of these PGLS regressions were significant and, in each regression, both explanatory variables (bone cross-sectional area and either Canmin or Canharmean) were significant (Table 1).
Table 1. Testing the relationship between the dependent variables used to quantify red blood cell size (RBCsize; RBCwidth and RBCarea) and the explanatory variables femoral vascular canal diameter (computed either as Canmin or Canharmean) and femoral cross-sectional area using phylogenetic generalized least-squares regression. *p-value < 0.05; **p-value < 0.01; ***p-value < 0.001.

Inferring the Thermophysiology of Notosuchia Using PLR
We used PLR to construct models aimed at computing the probability of being endothermic using paleohistological features (data available in Supplementary File 3). When Canharmean was used as the explanatory variable, we obtained a model with a transition rate α of 0.00144, an intercept estimate of 6.04 (p-value = 0.004) and an estimate for the coefficient of Canharmean of −0.45 (p-value = 0.001). The negative sign of the Canharmean coefficient indicates that the probability of being endothermic decreases as vascular canal diameter increases. Figure 3 shows the distribution of probabilities of being endothermic as a function of Canharmean variation. The corresponding equation is:

or

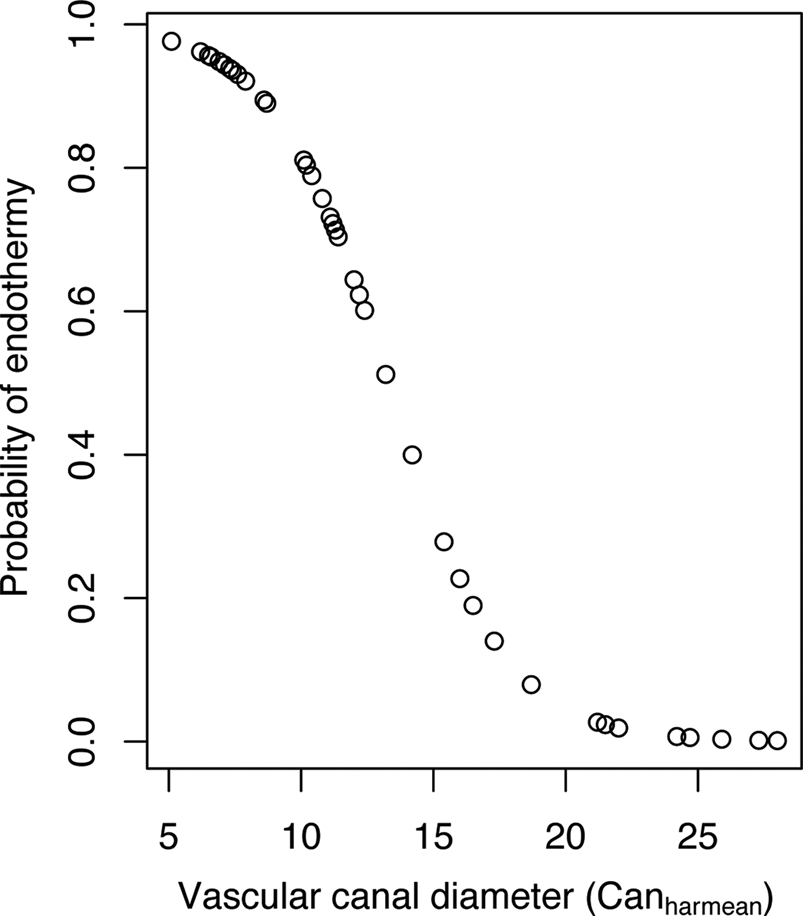
Figure 3. Distribution of probabilities of being endothermic inferred for our sample of extant tetrapods using a phylogenetic logistic regression model that includes femoral vascular canal diameter (computed as Canharmean) as the explanatory variable.
Ives and Garland (Reference Ives and Garland2010: p. 17) stated that “we assume that if μi <$\;\bar{{\rm \mu }}$, then trait Y will evolve toward 0; […] Conversely, if μi >
$\bar{{\rm \mu }}$, then trait Y will evolve toward 1,” where
$\bar{{\rm \mu }}$ is the mean probability of being endotherm in our sample. Thus, we considered that
$\bar{{\rm \mu }}$ is the cutoff probability, so that an inferred probability higher than
$\bar{{\rm \mu }}$ would be evidence for endothermy. Conversely, a probability lower than
$\bar{{\rm \mu }}$ would be evidence for ectothermy. When Canharmean was used as the explanatory variable,
$\bar{{\rm \mu }}$ = 0.59. To evaluate the predictive power of the model, we constructed a contingency table in which we inferred the thermometabolic regime of each extant species of the sample using its Canharmean. Lines contain predictions (0, inferred ectothermy; 1, inferred endothermy) and columns contain true states (0, observed ectothermy; 1, observed endothermy):

The specificity (the ratio of quantity of true 1 inferred as 1 on the quantity of true 1; Sp = 27/(27 + 2)) equals 0.931. The sensitivity (the ratio of quantity of true 0 inferred as 0 on the quantity of true 0; Se = 14/(14 + 3)) equals 0.824. The classification error (the ratio (quantity of true 0 inferred as 1 + quantity of true 1 inferred as 0)/total; error = (3 + 2)/(14 + 2 + 3 + 27)) equals 0.109. This classification error is quite low, so we used the cutoff probability of 0.59 to perform paleobiological inferences using Canharmean as the explanatory (predictor) variable.
When Canmin was used as the explanatory variable, we obtained a model with a transition rate α of 0.00052, an intercept estimate of 2.58 (p-value = 0.032), and an estimate for the coefficient of Canmin of −0.49 (p-value = 0.018). Figure 4 shows the distribution of probabilities of being endothermic as a function of Canmin variation. The corresponding equation is:

or
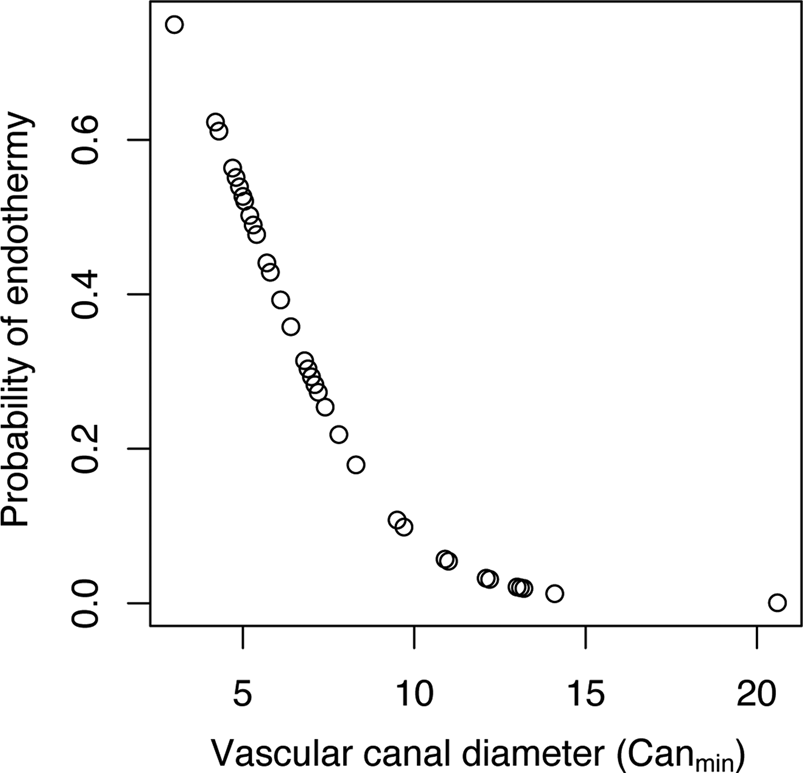
Figure 4. Distribution of probabilities of being endothermic inferred for our sample of extant tetrapods using a phylogenetic logistic regression model that includes femoral vascular canal diameter (computed as Canmin) as the explanatory variable.

Again we evaluated the quality of the model by constructing a contingency table in which we inferred the thermophysiological regime of each extant species of the sample using its Canmin. We used a cutoff probability of $\bar{{\rm \mu }}$ = 0.32 (the mean probability of being endothermic in our sample), so that an inferred probability lower than 0.32 is evidence for ectothermy and a probability higher than 0.32 is evidence for endothermy:

The specificity (the ratio of quantity of true 1 inferred as 1 on the quantity of true 1; Sp = 20/(20 + 9)) equals 0.690. The sensitivity (the ratio of quantity of true 0 inferred as 0 on the quantity of true 0; Se = 15/(15 + 2)) equals 0.882. The classification error (the ratio (quantity of true 0 inferred as 1 + quantity of true 1 inferred as 0)/total; error = (2 + 9)/(15 + 9 + 2 + 20)) equals 0.239. This classification error is quite high, so we recomputed a new cutoff probability of 0.22 using the receiver operating characteristic curve. Then we constructed a new contingency table in which we inferred the thermophysiological regime of each extant species of the sample using its Canmin and considering that an inferred probability higher than 0.22 is evidence for endothermy:

With this contingency table, the classification error [the ratio (quantity of true 0 inferred as 1 + quantity of true 1 inferred as 0)/total; error = (2 + 1)/(15+ 1 + 2 + 28)] equals 0.0652. This classification error is quite low, so we used the cutoff probability of 0.22 to perform paleobiological inferences using Canmin as the explanatory (predictor) variable.
We used these equations and cutoff probabilities and femoral Canharmean and Canmin values published by Cubo et al. (Reference Cubo, Sena, Aubier, Houee, Claisse, Faure-Brac, Allain, Andrade, Sayão and Oliveira2020) for Notosuchia to compute the probability of these taxa being endotherms (Table 2).
Table 2. Inferring the probability of endothermy for the sample of Notosuchia analyzed in this study using femoral vascular canal diameters (computed either as Canmin or Canharmean) as explanatory variables and phylogenetic logistic regressions. Vascular canal diameters for Notosuchia were taken from Cubo et al. (Reference Cubo, Sena, Aubier, Houee, Claisse, Faure-Brac, Allain, Andrade, Sayão and Oliveira2020).

Discussion
Notosuchia is an extremely diversified group of crocodyliforms. This diversity is particularly striking regarding their diet, suggesting that they occupied various ecological niches (Carvalho and Bertini Reference Carvalho and Bertini1999; Iori and Carvalho Reference Iori and Carvalho2018). Uruguaysuchidae (Pol et al. Reference Pol, Nascimento, Carvalho, Riccomini, Pires-Domingues and Zaher2014), the most basal notosuchians, of which Araripesuchus wegeneri (in our sample) is a representative, range from the Aptian (Araripesuchus gomesii) to the Maastrichtian (Araripesuchus tsangatsangana) (Price Reference Price1959; Turner Reference Turner2006). Several species of this group have been inferred as being omnivorous, or even insectivorous, based on their dental complexity (Sereno and Larsson Reference Sereno and Larsson2009; Soto et al. Reference Soto, Pol and Perea2011; Nieto et al. Reference Nieto, Degrange, Sellers, Pol and Holliday2021) and postcranial remains suggest that they had an erect posture (see “Introduction”). Uruguaysuchids were smaller than Sphagesauridae (Carvalho et al. Reference Carvalho, de Gasparini, Salgado, de Vasconcellos and Marinho2010; Godoy et al. Reference Godoy, Benson, Bronzati and Butler2019). Armadillosuchus (also sampled by us) belongs to the large-bodied sphagesaurids group (Melstrom and Irmis Reference Melstrom and Irmis2019). The diagnosis of this clade is based on its peculiar dentition morphology (Price Reference Price1950). They show extremely complex manducatory systems, with evidence of “chewing” mechanisms, dental wear, and propalinal movements (e.g., see Ősi Reference Ősi2014; Iori and Carvalho Reference Iori and Carvalho2018). The foraging abilities of some notosuchians, such as Armadillosuchus, Mariliasuchus, or Malawisuchus, to locate food or water have led some authors to propose the presence of burrowing habits (Gomani Reference Gomani1997; Nobre et al. Reference Nobre, de Souza Carvalho, de Vasconcellos and Souto2008; Marinho and Carvalho Reference Marinho and Carvalho2009), a behavior that might play a role in thermoregulation (e.g., to search for a cooler shelter during dry periods, as in extant crocodilian species; Campos and Magnusson Reference Campos and Magnusson2013). This behavior has also been proposed for the sebecosuchian Baurusuchus salgadoensis (Vasconcellos and Carvalho Reference Vasconcellos and Carvalho2010). Sebecosuchia (to which Iberosuchus and Stratiotosuchus, also sampled by us, belong) were large predators with an erect posture (see “Introduction”) and cursorial abilities (Nascimento and Zaher Reference Nascimento and Zaher2010; Riff and Kellner Reference Riff and Kellner2011), feeding on large prey, including small sphagesaurids (Godoy et al. Reference Godoy, Montefeltro, Norell and Langer2014). Indeed, their ziphodont teeth (unicuspidated, laterally compressed with serrated carinae) associated with the biomechanical performances of their skull allowed sebecosuchians to effectively handle prey after wounding it (Montefeltro et al. Reference Montefeltro, Lautenschlager, Godoy, Ferreira and Butler2020). It is noteworthy that the inferred ectothermic sebecosuchians occupy a niche usually occupied by endothermic theropod dinosaurs (Benson et al. Reference Benson, Mannion, Butler, Upchurch, Goswami and Evans2013; Zanno and Makovicky Reference Zanno and Makovicky2013).
The ectothermic condition of Notosuchia suggested by Cubo et al. (Reference Cubo, Sena, Aubier, Houee, Claisse, Faure-Brac, Allain, Andrade, Sayão and Oliveira2020) is supported by the results of the present study using larger sample sizes of extant species and a more robust phylogenetic comparative method (PLR). First, the finding that RBCsize is related to bone vascular canal diameter (Huttenlocker and Farmer Reference Huttenlocker and Farmer2017) is supported by our results obtained using a sample size more than twice of that used by these authors. Thus bone vascular canal diameter can be used as a proxy to infer RBCsize, and then endothermy (because within tetrapods, RBCsize is lower in endotherms than in ectotherms; Snyder and Sheafor Reference Snyder and Sheafor1999). Huttenlocker and Farmer (Reference Huttenlocker and Farmer2017) included bone cross-sectional area (in addition to bone vascular canal diameter) as an explanatory variable in models aimed at explaining the variation of RBCsize. However, in their study, bone cross-sectional area did not improve the explanatory power of models and was not retained (Huttenlocker and Farmer Reference Huttenlocker and Farmer2017; Supplemental Information, “Analysis I, Training Data Set for Extant Taxa”). Unexpectedly, our analyses (using a larger sample size) showed that bone cross-sectional area significantly improves the explanatory power of models and is retained, together with bone vascular canal diameter, in models explaining the variation of RBCsize. The fact that the estimate for bone cross-sectional area is always negative (Table 1) indicates that RBCsize decreases as bone cross-sectional area increases. Second, notosuchian thermometabolism is inferred here using a larger sample of extant tetrapods (more than three times the sample used by Cubo et al. [Reference Cubo, Sena, Aubier, Houee, Claisse, Faure-Brac, Allain, Andrade, Sayão and Oliveira2020]) and logistic phylogenetic regressions (a method more reliable than those used in previous studies; see below). We are aware of the fact that Huttenlocker and Farmer (Reference Huttenlocker and Farmer2017) showed that histological changes reflect changes in VO2max better than changes in thermometabolism. However, we have found here that microstructural variation is linked to thermometabolism too. The models constructed to infer the probability of endothermy using vascular canal size as an explanatory (predictive) variable were highly significant, and the classification errors obtained are quite low (6.5% using Canmin). Thus we conclude that we can use these models confidently in paleobiological inference of thermometabolism.
Thermal paleophysiology is an emergent discipline (Cubo and Huttenlocker Reference Cubo and Huttenlocker2020). It has great potential resulting from the synergy between physiological studies aimed at deciphering the mechanisms of thermogenesis in extant amniotes (e.g., Bal and Periasamy Reference Bal and Periasamy2020; Jastroch and Seebacher Reference Jastroch and Seebacher2020; Grigg et al. Reference Grigg, Nowack, Bicudo, Pereira, Bal, Woodward and Seymour2021) and paleobiological inferences in extinct amniotes using phylogenetic comparative methods (e.g., Cubo et al. Reference Cubo, Le Roy, Martinez-Maza and Montes2012, Reference Cubo, Sena, Aubier, Houee, Claisse, Faure-Brac, Allain, Andrade, Sayão and Oliveira2020, Reference Cubo, Buscalioni, Legendre, Bourdon, Sanz and de Ricqlès2022; Legendre et al. Reference Legendre, Guénard, Botha-Brink and Cubo2016; Huttenlocker and Farmer Reference Huttenlocker and Farmer2017; Olivier et al. Reference Olivier, Houssaye, Jalil and Cubo2017; Fleischle et al. Reference Fleischle, Wintrich and Sander2018; Cubo and Jalil Reference Cubo and Jalil2019; Faure-Brac et al. Reference Faure-Brac, Amiot, de Muizon, Cubo and Lécuyer2021; Knaus et al. Reference Knaus, Van Heteren, Lungmus and Sander2021). Logistic phylogenetic regressions are the third step in efforts performed during the last decade to carry out reliable inferences of thermometabolic status in extinct amniotes. A decade ago, Cubo et al. (Reference Cubo, Le Roy, Martinez-Maza and Montes2012) inferred bone growth rates using bone histological features and multiple linear regressions tested for significance using permutations in order to circumvent the nonindependence of the observations due to the phylogeny. Considering that bone growth rates are significantly related to RMR in amniotes (Montes et al. Reference Montes, Le Roy, Perret, de Buffrénil, Castanet and Cubo2007), the former was used by Cubo et al. (Reference Cubo, Le Roy, Martinez-Maza and Montes2012) as a proxy to infer the thermometabolic status of extinct archosaurs. This method was used by Legendre et al. (Reference Legendre, Segalen and Cubo2013) to infer the bone growth rate and the thermometabolic status of Euparkeria. In a second step, Legendre et al. (Reference Legendre, Guénard, Botha-Brink and Cubo2016) adapted Guénard et al.'s (Reference Guénard, Legendre and Peres-Neto2013) PEMs to perform paleobiological inferences of RMR. This contribution represented significant methodological progress, because paleobiological inference models included the phylogeny (rather than circumventing its effects, as did the preceding method), assuming an evolutionary model (Molina-Venegas et al. Reference Molina-Venegas, Moreno-Saiz, Castro Parga, Davies, Peres-Neto and Rodríguez2018). PEMs have been widely used to infer the thermometabolic status of extinct amniotes (Legendre et al. Reference Legendre, Guénard, Botha-Brink and Cubo2016; Olivier et al. Reference Olivier, Houssaye, Jalil and Cubo2017; Fleischle et al. Reference Fleischle, Wintrich and Sander2018; Cubo and Jalil Reference Cubo and Jalil2019; Cubo et al. Reference Cubo, Sena, Aubier, Houee, Claisse, Faure-Brac, Allain, Andrade, Sayão and Oliveira2020, Reference Cubo, Buscalioni, Legendre, Bourdon, Sanz and de Ricqlès2022; Faure-Brac and Cubo Reference Faure-Brac and Cubo2020; Faure-Brac et al. Reference Faure-Brac, Amiot, de Muizon, Cubo and Lécuyer2021; Knaus et al. Reference Knaus, Van Heteren, Lungmus and Sander2021). Using logistic phylogenetic regressions is a new step in this sequence. This method improves upon the previous approach by using all of the phylogenetic information (rather than a fraction of it, as did PEMs in order to avoid model overfitting). An encouraging sign is that results are congruent in spite of the diversity of methods used to obtain them. Inferring the maximum metabolic rate of Notosuchia using the size of femoral nutrient foramina (Seymour et al. Reference Seymour, Smith, White, Henderson and Schwarz-Wings2012) would be the next promising step to fully understand the thermophysiology of these amazing crocodylomorphs.
Acknowledgments
We thank H. Lamrous (Sorbonne Université) for helping us with picture acquisition using bone thin sections at the Vertebrate Hard Tissue Collection of the Museum national d'Histoire naturelle (Paris). This study was partly funded by the project Emergences Sorbonne Université 2019 no. 243374 to J.C. The authors declare no competing interests.
Data Availability Statement
Data available from the Dryad and Zenodo Digital Repositories: https://doi.org/10.5061/dryad.80gb5mktb, https://doi.org/10.5281/zenodo.6795231.