Introduction
Food is a primary requirement to live. Yet, even when food is abundantly available, food could also be used as a powerful tool to increase mental well-being. Stress-related mental disorders such as mood or anxiety disorders are the most prevalent (10 to 25 % women and 5 to 12 % men are being diagnosed each year(1)), and burdensome, psychiatric disorders. They are characterised by low mood states and cognitive impairments such as reduced learning and memory(1). Thus, the exploitation of resilience or mood/cognition-enhancing food is of extreme value. Yet, whereas pharmacological manipulations have been abundantly applied, surprisingly little is known about the effects of food on mood and cognition.
The monoamine systems are strongly tied to stress resilience and the (patho)physiology of mood and mood disorders. As such, tricyclic antidepressants (inhibiting serotonin and noradrenaline reuptake) and selective serotonin reuptake inhibitors (SSRI) are frequently prescribed drugs in the treatment of these disorders. To a limited extend these agents also inhibit dopamine reuptake. Whereas antidepressant drugs are considered as healthy drugs, their uncontrolled intake is not without risks. For instance, at a young age these drugs may affect brain development and increase suicide(Reference Olivier, Chan and Snoeren2). Furthermore, triple monoamine reuptake inhibition corresponds to the function of cocaine, which is a powerful psychostimulant with abuse potential. In addition to monoamine reuptake inhibitors, also drugs that inhibit the breakdown of monoamines are used to improve mood, particularly monoamine oxidase A (MAO-A) inhibitors. However, these agents are also associated with substantial side effects. A major risk is the serotonin syndrome; too high levels of serotonin are toxic. Hence, whereas monoamine reuptake inhibitors and MAO-A inhibitors improve mood, particularly in individuals with a low mood state, their use is not without risks.
To improve mood by other means, food with high tryptophan (Trp) availability and modest monoamine reuptake and MAO-A inhibition functional profile may be effective. The serotonin precursor Trp is an essential amino acid that in mammals can only be derived from food(Reference Wurtman, Hefti and Melamed3). Therefore, Trp levels in food appear to be an essential link in the relationship between food intake and our mood state. Accordingly, high-Trp-containing foods (for example, chicken, soyabeans, cereals, tuna, nuts and bananas) are presented on the Internet as food to improve mood. Furthermore, sometimes unknowingly, the Trp content of food has been increased. For instance, Neolithic Mesopotamia-cultivated chickpeas contained more Trp than wild varieties(Reference Kerem, Lev-Yadun and Gopher4). Another example is the treatment of maize with alkali by various cultures in America, which enhances the bioavailability of Trp(Reference Katz, Hediger and Valleroy5, Reference Morris and Sands6). More recently, research into α-lactalbumin has revealed that this whey-derived protein has the highest Trp content of all proteins found in food and therefore is added to infant milk formulas(Reference Heine, Radke and Wutzke7). Finally, an even more promising source of Trp is egg protein hydrolysate(Reference Mitchell, Slettenaar and Quadt8). Pharmacological studies have confirmed that products that increase Trp levels increase serotonin levels in the brain, and apparently modulate processing in the neurocircuits regulating mood to enhance mood(Reference Markus, Firk and Gerhardt9, Reference Silber and Schmitt10). Trp depletion, on the other hand, is well known to affect mood negatively(Reference Booij, Van der Does and Riedel11). Hence, controlling the amount of Trp in food has substantial effects on mood regulation and well-being, although data as discussed in the present review indicate that the relationship between Trp levels and mood is less straightforward than we tend to think. Foods having triple monoamine reuptake and MAO-A inhibitor function have been less extensively investigated, even though they may offer another natural source to boost mood. Indeed, these foods have significant anti-depressive and anxiolytic effects in rats which are comparable with the effects of antidepressant reference drugs(Reference Mechan, Fowler and Seifert12).
The aim of the present review is to provide an update about foods influencing the serotonergic system that have potential to increase stress resilience and thereby our well-being. Rather than applying pharmacological drugs when pathology has developed, prevention by healthy foods may decrease the burden associated with mood disorders. An important consideration is the heterogeneity of mood disorders, caused by genetic or other biological factors. Along with the fact that there are large individual differences in vulnerability to mood disorders, there are large individual differences in responsivity to antidepressant agents(Reference Kroeze, Zhou and Homberg13), as well as in responsivity to serotonergic food components. These individual differences play a leading role in our discussion of the effects of serotonergic foods on mood. In sum, the present review discusses the serotonergic system, and the influence of serotonergic food components on mood and cognition in healthy, genetically vulnerable and clinically depressed and recovered populations, as well as (genetic) rodent models.
The serotonergic system
Serotonin, or 5-hydroxytryptamine (5-HT), is an ancient monoamine, as even some unicellular organisms are able to synthesise it. Furthermore, it is found in basically all animal species and various plants such as stinging nettles and walnuts. Apart from its roles in the cardiovascular and digestive systems, 5-HT is an important neurotransmitter with a wide variety of actions in different brain regions. Because 5-HT as a hydrophilic molecule is unable to cross the blood–brain barrier, it is synthesised in the brain, specifically the serotonergic neurons in the raphe nuclei itself, by the enzyme tryptophan hydroxylase 2 (TpH2). TpH2 is also expressed in myenteric neurons in the gut(Reference Neal, Parry and Bornstein14). TpH2 is distinct from tryptophan hydroxylase 1 (Tph1), the enzyme that is responsible for the 5-HT system in the peripheral system, mainly the gut. The synthesis of 5-HT in the brain takes place in the axon terminals of serotonergic neurons where TpH2 exerts the first rate-limiting step. TpH2 hydroxylates l-tryptophan and forms 5-hydroxytryptophan. This molecule is subsequently decarboxylated by aromatic l-amino acid decarboxylase to form 5-HT. The 5-HT molecules are stored in vesicles in the serotonergic neurons. Upon an action potential, 5-HT is released from axon terminals of serotonergic neurons into the synaptic cleft where it binds to its receptors on the postsynaptic neuron. Its activity is terminated by the serotonin transporter (5-HTT) in the membrane of the presynaptic neuron that transports 5-HT back into the cell. This allows recycling of 5-HT, although stored/released 5-HT is also degraded by MAO-A. Fine-tuning of the postsynaptic response to the release of 5-HT is achieved through a host of different receptors and cells and/or brain area-specific receptor profiles, thereby facilitating the different roles that 5-HT plays in physiological and behavioural functions.
Serotonergic cell bodies are located in the raphe nuclei, a cluster of nuclei in the brain stem projecting to almost all brain regions(Reference Azmitia, Whitaker-Azmitia, Bloom and Kupfer15), including lateral cortical regions, the amygdala and the hypothalamus, which are all involved in processing emotions and stress. Because TpH2, 5-HTT and MAO-A are expressed in these and other brain areas(Reference Gutknecht, Kriegebaum and Waider16–Reference Torres, Gainetdinov and Caron18), agents acting through TpH2, 5-HTT and MAO-A will have a global effect on 5-HT neurotransmission.
Although the precise role of 5-HT in mood disorders such as depression, obsessive-compulsive disorder and anxiety is not fully understood, it is evident that the 5-HT system is perturbed in these disorders. The synthesis, release, reuptake and/or metabolism of 5-HT(Reference Van Praag, Korf and Puite19, Reference Malison, Price and Berman20), as well as the functionality of the 5-HT1A and 5-HT2 serotonin receptors(Reference Cowen, Power and Ware21, Reference Sargent, Kjaer and Bench22), are to different extents affected in anxiety and depression. The general view is that there is an inverse relationship between depression and 5-HT levels, based on the antidepressive properties of serotonin-enhancing drugs(Reference Nutt23). In terms of food, a positive association between the consumption of maize, which contains less Trp compared with other food staples, and homicide rates has been found(Reference Mawson and Jacobs24). Moreover, another cross-national study revealed a negative correlation between dietary Trp and suicide rates(Reference Voracek and Tran25).
There is also evidence that genetic variation in the serotonergic system increases vulnerability to anxiety- and depression-related disorders(Reference Gordon and Hen26–Reference Maes, Meltzer, Bloom and Kupfer29). A common polymorphism in the promoter region of the 5-HTT (SLC6A4) gene (abbreviated: 5-HTTLPR; serotonin-transporter-linked polymorphic region) is associated with trait anxiety(Reference Lesch, Bengel and Heils30), and in the context of (early-life) stress, this polymorphism increases risk for depression(Reference Caspi, Sugden and Moffitt31). Paradoxically, it is the low-activity short (s) allelic variant that increases vulnerability. Although human studies could not provide evidence, studies employing 5-HTT knockout mice and rats have revealed that reduced 5-HTT expression is associated with increased synaptic 5-HT levels(Reference Kalueff, Olivier and Nonkes32). Hence, whereas SSRI-induced increases in 5-HT levels reduce depressive symptoms, genetically induced constitutive high 5-HT levels appear to increase these symptoms. Furthermore, whereas 5-HTTLPR s-allele carriers are at risk for depression if exposed to stress, those that are depressed suffer from decreased responsivity to SSRI. Intuitively, decreased 5-HTT expression leaves a smaller window of opportunity for SSRI to exert their maximum effects.
In 1998, gene mapping of the X-chromosomal MAO-A gene revealed a functional polymorphism in the upstream promoter region, consisting of a variable number of tandem repeats (u-VNTR). The different lengths of these variants was found to influence protein transcription and thus enzymic activity(Reference Sabol, Hu and Hamer33). Others linked the alleles which confer high enzymic activity (three, four and five repeats) to several anxiety- and depression-related disorders(Reference Deckert, Catalano and Syagailo34–Reference Yu, Tsai and Hong37), as well as reduced responsiveness to antidepressant treatment(Reference Yu, Tsai and Hong37, Reference Domschke, Braun and Ohrmann38).
Finally a number of different TpH2 SNP have been reported in several ethnical cohorts which are thought to cause, through either lower enzymic activity or loss of function, lower extracellular 5-HT levels. Zhang et al. (Reference Zhang, Gainetdinov and Beaulieu39) showed that an 80 % loss of function TpH2 G1463A SNP found in a geriatric sample in North Carolina (USA) was more prevalent in the cohort diagnosed with unipolar depression compared with the matching control cohort. Another study revealed in Taiwanese Han Chinese subjects a possible association between bipolar disorder and TpH2 C2755A, which leads to a reduction of 5-HT synthesis when expressed in cells(Reference Lin, Chao and Chen40), and TpH2 Pro206Ser (conferring a 30 % reduction in enzymic activity) was found to be significantly higher expressed in a cohort of German patients suffering from bipolar disorder compared with controls(Reference Cichon, Winge and Mattheisen41). In addition to these SNP, the study on Taiwanese Han Chinese also reported that the − 703G/ − 473A haplotype (a TpH2 promoter variant associated with bipolar disorder) resulted in reduced transcription of the TpH2 gene as well as reduced affinity for the transcription factor POU3F2(Reference Lin, Chao and Chen40).
Because 5-HTT, Thp2 and/or MAO-A polymorphisms affect the functioning of the serotonergic system, it is to be expected that they influence the effects of serotonergic food components, either through reduced capacity to convert Trp into 5-HT (due to reduced TpH2 function), reduced sensitivity of the serotonergic system to elevated 5-HT levels (due to reduced 5-HTT function) or enhanced degradation of 5-HT (due to elevated MAO-A activity).
Tryptophan
Since 5-HT cannot cross the blood–brain barrier due to its hydrophilic properties the synthesis of the neurotransmitter takes place in the axon terminals of serotonergic neurons. The precursor Trp is absorbed from food into the bloodstream. When a meal containing protein is consumed a large proportion of Trp entering the portal vein is metabolised in the liver, which does not happen with the other large neutral amino acids (LNAA)(Reference Ross, Fernstrom and Wurtman42). Thus the amount of Trp entering the circulation is much less compared with other LNAA, if the amounts consumed are the same. However, the secretion of insulin in response to the intake of carbohydrates in the meal reduces the plasma concentrations of all LNAA except Trp, which binds to albumin and thereby can circulate throughout the body(Reference Yuwiler, Oldendorf and Geller43, Reference Fernstrom, Larin and Wurtman44). Whether a meal increases the ratio of plasma Trp levels compared with the plasma levels of other LNAA (the TRP:LNAA ratio) depends on the amount of protein digested and the release of insulin(Reference Fernstrom and Wurtman45, Reference Fernstrom and Faller46). When Trp in the circulation reaches the brain capillaries it is transported across the blood–brain barrier. Here Trp has to compete with other LNAA for active transport across the cell membranes of the epithelial cells lining the capillaries. Therefore, not the plasma level of Trp alone, but the TRP:LNAA ratio determines its rate of transport across the blood–brain barrier(Reference Fernstrom and Wurtman45). Once inside the brain, Trp is taken up by the serotonergic cells of the raphe nuclei and via two enzymic reactions it is converted into 5-HT (see the section on the serotonergic system).
Acute tryptophan depletion
Since there is a direct relationship between dietary Trp and brain 5-HT synthesis, the supply of 5-HT in the brain can be exhausted by decreasing the availability of Trp. A standardised procedure to drastically decrease the levels of 5-HT is acute tryptophan depletion (ATD). In short, a diet low in Trp is eaten by the subjects for 24 h, after which they fast overnight and during the test day except for a shake which contains all amino acids excluding Trp. This in itself causes a drop in the plasma TRP:LNAA ratio. Furthermore, protein synthesis is stimulated by the intake of high-protein foods or drinks. A protein-rich low-carbohydrate diet results in a decreased amount of Trp in the brain since the TRP:LNAA ratio in normal protein is very low. Therefore, a protein-rich low-carbohydrate diet results in more competition for the transport system over the blood–brain barrier and consequently less transport of Trp into the brain. As a consequence, Trp needed for protein synthesis during the diet and test day must be supplied by the reserves in the body. Inevitably these reserves will be drained to the point that they cannot maintain plasma Trp levels, causing the TRP:LNAA ratio to drop even further, to about 15 % within 5·5 h. Brain Trp levels drop to 5 % several hours later, which hampers brain 5-HT synthesis(Reference Carpenter, Anderson and Pelton47–Reference Williams, Shoaf and Hommer49). The effects of ATD in healthy volunteers and vulnerable subjects are described in the following sections.
Effects of acute tryptophan depletion on mood and cognitive functions in healthy subjects
Mood
In line with the increased incidence of depression in women, it has been reported that the mood of healthy female volunteers but not of healthy male volunteers dropped after ATD (about 80–90 % reduction in plasma Trp)(Reference Ellenbogen, Young and Dean50, Reference Smith, Clifford and Hockney51). In male subjects, effects of ATD on mood were either small(Reference Smith, Pihl and Young52, Reference Young, Smith and Pihl53) or fully absent (about 70–90 % reduction in plasma Trp)(Reference Ellenbogen, Young and Dean50, Reference Smith, Clifford and Hockney51, Reference Abbott, Etienne and Franklin54, Reference Danjou, Hamon and Lacomblez55). positron emission tomography (PET) imaging showed that there may be differences in 5-HT synthesis and metabolism between the sexes, which could explain the difference in response to ATD(Reference Nishizawa, Benkelfat and Young48). Other studies reported that the performance of men in the Stroop Color Word Test, testing selective attention and response inhibition, improved following ATD (about 60–80 % reduction in plasma Trp)(Reference Schmitt, Jorissen and Sobczak56, Reference Scholes, Harrison and O'Neill57). However, the effects of ATD on recognition of emotional faces are inconsistent; some observed a decrease in recognition of facial emotions following ATD (no complete data on plasma Trp) in women(Reference Harmer, Rogers and Tunbridge58), others found an increase in the recognition of happy faces by women (plasma Trp reduced by 55 %)(Reference Hayward, Goodwin and Cowen59), and still others reported no effects in both men and women following a Trp reduction of 65 %(Reference Van Der Veen, Evers and Deutz60, Reference Cools, Calder and Lawrence61). Thus, whereas ATD impairs mood in women, there are conflicting results concerning the effects of ATD on the recognition of emotional faces.
Cognition
Apart from mood, ATD has been found to affect a number of cognitive functions in healthy individuals. Memory consolidation was impaired when the learning of a list of words took place during the Trp-depleted state (about 60–80 % reduction in plasma Trp), whereas the recall of word lists learned before the ATD procedure was not affected(Reference Schmitt, Jorissen and Sobczak56, Reference Riedel, Klaassen and Deutz62). Moreover, McAllister et al. (Reference McAllister-Williams, Massey and Rugg63) showed that ATD leading to a plasma Trp reduction of 85 % significantly impaired source memory recall. In sum, research consistently shows memory impairment following ATD.
Effects of acute tryptophan depletion on mood and cognitive functions in vulnerable subjects
Mood
In vulnerable and subclinical subjects more consistent evidence has been found pointing towards a lowering of mood and alterations in cognitive functioning in response to ATD. For instance, healthy subjects with a multigenerational family history of affective disorders showed a reduction in mood as measured the by the Profile of Mood States scale following ATD (about 70–90 % reduction in plasma Trp)(Reference Benkelfat, Ellenbogen and Dean64, Reference Klaassen, Riedel and van Someren65). The same is true for patients who are remitted from a depressive episode and responded to SSRI treatment (Trp plasma reduced by about 50–90 %)(Reference Delgado, Charney and Price66–Reference Smith, Fairburn and Cowen69); however, see Leyton et al. (Reference Leyton, Young and Blier70). In remitted depressed patients, ATD impaired attention to positive stimuli (about 55–64 % reduction in plasma Trp)(Reference Booij, Van der Does and Haffmans71) and decreased the recognition of fearful and happy faces (plasma Trp reduced by 55–84 %)(Reference Hayward, Goodwin and Cowen59, Reference Merens, Booij and Haffmans72). Furthermore, in otherwise healthy women as well as remitted major depression patients carrying the 5-HTTLPR ss or ls genotype it was found that ATD significantly lowered mood compared with l-allele carriers (about 70–85 % reduction in plasma Trp)(Reference Neumeister, Konstantinidis and Stastny73–Reference Walderhaug, Magnusson and Neumeister75). Interestingly, men carrying the same genotype did not experience a lower mood, but instead showed increased impulsivity after ATD (plasma Trp reduced by about 75–85 %)(Reference Walderhaug, Magnusson and Neumeister75, Reference Walderhaug, Herman and Magnusson76). When ATD (77 % reduction in plasma Trp) was combined with a stressor, mood deteriorated even further in 5-HTTLPR ss subjects, while no further reduction was found in individuals homozygous for the l allele(Reference Firk and Markus77). In summary, a vulnerable serotonergic system, whether due to genetic background or a (family) history of affective disorders, seems to enhance the mood-deteriorating effects of ATD.
Cognition
Subclinical subjects tend to suffer from instable cognitive function, which increases the risk of cognitive impairments when cognitive capacities are too low to properly deal with environmental challenges, like stress, but also unhealthy food. In their pooled analysis of nine separate ATD trials, Sambeth et al. (Reference Sambeth, Blokland and Harmer78) discovered that the memory impairment effect of ATD (63–97 % reduction in plasma Trp) was more prominent in women than in men(Reference Sambeth, Blokland and Harmer78). ATD (plasma Trp reduced by 69 %) also lowered incentive motivation in 5-HTTLPR s-allele carriers(Reference Roiser, Blackwell and Cools79). In remitted depressed patients, ATD impaired immediate verbal recall as well as immediate and delayed recall of lists of spoken words (plasma Trp reduced by 55–71 %)(Reference Hayward, Goodwin and Cowen59, Reference Porter, Phipps and Gallagher80, Reference Roiser, Muller and Clark81). However, it improved attention to neutral stimuli (about 55–64 % reduction in plasma Trp)(Reference Booij, Van der Does and Haffmans71).
Effects of acute and (sub-)chronic tryptophan depletion in animal models
While the behavioural effects of ATD on human subjects are well documented, research on the effects of Trp depletion in animal models can offer further insight into the role of 5-HT in mood and cognitive processes and enable researchers to examine the effects of (sub-)chronic Trp depletion. For instance, sub-chronic Trp depletion (SCTD) (57 % reduction in plasma Trp, prefrontal cortex 5-HT levels reduced by 34 %) in Sprague–Dawley rats led to depression-related behaviour in the forced swim test as well as an increase in corticosterone, aldosterone, 5-HT receptor density and increased N-methyl-D-aspartate (NMDA) signalling(Reference Franklin, Bermudez and Murck82). In another study reward learning was impaired without an effect on motivation, assessed by effort discounting and reversal learning tasks, following SCTD (no data on plasma Trp levels, ventromedial frontal cortex 5-HT levels reduced to about 50 % of control) in Long Evans rats(Reference Izquierdo, Carlos and Ostrander83). SCTD (no data on plasma Trp levels, prefrontal cortex 5-HT levels reduced to 39 % of control) in Wistar rats also resulted in enhanced proneness to gamble and poor decision making in the rodent Iowa Gambling Task(Reference Koot, Zoratto and Cassano84). Interestingly, also in macaques performing a gambling task, ATD (about 65 % reduction in plasma Trp) increased risky decision making(Reference Long, Kuhn and Platt85). Chronic Trp depletion in Lister hooded rats (40–50 % reduction of 5-HT levels in the hippocampus, frontal cortex and striatum) impaired object recognition in the novel object-recognition paradigm, which was reversed by administering the atypical antipsychotic risperidone(Reference Jenkins, Elliott and Ardis86). ATD studies in rats have also revealed that the effects of ATD (about 50 % reduction in plasma Trp) on hippocampal and frontal cortex 5-HT levels were more pronounced in homozygous serotonin transporter knockout Wistar rats compared with heterozygous knockout rats and wild-type controls(Reference Olivier, Jans and Korte-Bouws87). Finally, the circadian rhythm of locomotor activity as well as the sleep–wake cycle were found to be disturbed in Sprague–Dawley rats by ATD (99 % reduction in plasma Trp, 85 % decrease in 5-HT brain levels)(Reference Nakamaru-Ogiso, Miyamoto and Hamada88). In conclusion, animal studies have provided evidence that Trp depletion indeed lowers 5-HT levels in brain areas that are involved in several cognitive functions and mood, thus reinforcing the conclusions drawn from human ATD studies and providing further insight into the underlying processes. However, some care should be taken when reviewing these studies on Trp depletion in rats, as not all strains respond in the same manner to Trp depletion(Reference Jans, Korte-Bouws and Korte89).
Summary of acute tryptophan depletion
In healthy subjects the negative effects of ATD on memory are the most profound, while it seems to enhance selective attention and inhibition of response. Interestingly, the lowering of mood by ATD in healthy subjects is only consistently found in females. This sex effect may be a consequence of lower 5-HT synthesis in females than in males(Reference Nishizawa, Benkelfat and Young48), making females more vulnerable to manipulation of the serotonergic system. This is also true for subjects that have a vulnerable serotonergic system as a result of genetic or (family) history of affective disorder, as is seen in the enhanced lowering of mood and negative effects on several cognitive functions in these vulnerable subpopulations.
Acute tryptophan supplementation
Whereas consumption of a high-protein low-carbohydrate diet lowers Trp levels(Reference Fernstrom90), consumption of a diet high in carbohydrates increases the Trp:LNNA ratio in blood and the brain(Reference Fernstrom, Larin and Wurtman44, Reference Fernstrom and Wurtman91). The intake of a high amount of carbohydrates triggers the release of insulin that in turn stimulates the uptake of LNAA into muscle tissue, with the exception of Trp, which is loosely bound by free albumin. Combined with a low intake of protein, the plasma TRP:LNAA ratio shifts in favour of Trp and therefore increases the transport of Trp over the blood–brain barrier. This method, however, only mildly elevates Trp levels in the brain, rarely exceeding an elevation of 25 % over baseline. Furthermore, most typical diets high in carbohydrates are high in protein as well, negating their effect on plasma Trp levels(Reference Yokogoshi and Wurtman92). A valid alternative is the administration of pure Trp via intravenous injection in which the precise elevation of pure Trp levels can be observed. This method, however, proves to be quite invasive and inconvenient as a regular event. Yet another approach is oral administration of a drink high in α-lactalbumin retrieved from whey which is high in Trp(Reference Heine, Radke and Wutzke7) and therefore can increase plasma Trp without increasing the amount of competing LNAA. In several studies, α-lactalbumin was administered as a chocolate-flavoured drink; thus this method is much more feasible for a continued administration and even rather pleasant for the test subject, although the increase in Trp via this method is limited and significantly lower than via intravenous injection(Reference Markus, Olivier and Panhuysen93, Reference Orosco, Rouch and Beslot94). More recently hydrolysed protein and egg protein hydrolysate in the form of a sweetened drink have been found to raise brain Trp up to 255 %, so oral administration does not necessarily produce a smaller increase in plasma Trp than pure intravenous injections(Reference Mitchell, Slettenaar and Quadt8, Reference Markus, Firk and Gerhardt9). Independent of the method, an increased amount of Trp in the brain results directly in an increase in 5-HT synthesis since the rate-limiting enzyme TpH2 is only half saturated under normal circumstances and 5-HTP aromatic l-amino acid decarboxylase is a highly active enzyme(Reference Markus, Firk and Gerhardt9).
In the last decades, there have been many studies on the effects of acute Trp supplementation (ATS) on mood and cognition, utilising either amino acid drinks containing pure Trp, α-lactalbumin, hydrolysed protein or egg protein hydrolysate. Because there is no standardised protocol for ATS, the obtained Trp:LNAA levels after digestion of the ATS mixture or diet can differ greatly between studies. Nonetheless, significant effects of ATS have been observed in both healthy and (sub)clinical populations. In the next sections modulation of various cognitive functions and mood by ATS in healthy and vulnerable subjects will be discussed.
Effects of acute tryptophan supplementation on mood and cognitive functions in healthy subjects
Mood
ATS seems to modulate emotional processing in healthy subjects, in a dose-dependent manner. An oral dose of 1·8 g Trp (600 % increase in TRP:LNAA) increased the recognition of fearful and happy faces in females(Reference Attenburrow, Williams and Odontiadis95). Murphy et al. (Reference Murphy, Longhitano and Ayres96) found increased recognition of happy facial expressions, and a decreased recognition of expressions of disgust after 14 d of 3 g Trp/d (no data on TRP:LNAA ratio) in healthy female subjects. In another study, drinks containing a hydrolysed egg-white protein, which increases plasma TRP:LNAA by 191–255 %, improved mood(Reference Markus, Firk and Gerhardt9). However, carbohydrate-rich protein-poor diets or drinks containing α-lactalbumin that increase plasma TRP:LNAA by 21–67 % did not have an effect on mood(Reference Markus, Firk and Gerhardt9, Reference Markus, Olivier and Panhuysen93, Reference Markus, Panhuysen and Tuiten97–Reference Booij, Merens and Markus100). Furthermore, unphysiologically high increases in TRP:LNAA ratios negatively affected mood, as was reported after an intravenous injection of 7 g pure Trp (1500 % increase in TRP:LNAA)(Reference Sobczak, Honig and Van Duinen101, Reference Sobczak, Honig and Schmitt102). Thus, there may be an inverted U-shaped relationship between TRP:LNAA ratios and mood in healthy subjects.
Cognition
Considering that memory consolidation and recall are impaired after ATD and in depression(Reference Schmitt, Jorissen and Sobczak56, Reference Riedel, Klaassen and Deutz62, Reference McAllister-Williams, Massey and Rugg63), ATS may enhance these cognitive functions. Indeed, it has been reported that an increase of 21 % in TRP:LNAA following the administration of two α-lactalbumin-rich drinks improved abstract visual memory in healthy subjects(Reference Booij, Merens and Markus100). However, Sobczak et al. (Reference Sobczak, Honig and Schmitt102) found that an intravenous injection of 7 g Trp had an adverse effect on performance in memory tests. Given that extreme elevation of TRP:LNAA (1500 %) caused significant sedation in the test subjects(Reference Sobczak, Honig and Schmitt102, Reference Luciana, Burgund and Berman103), these detrimental effects could well be due to sedation and not a direct effect of increased 5-HT levels. For instance, ATS (770 % increase in plasma free Trp, no data on TRP:LNAA ratio) has been found to increase reaction times(Reference Murphy, Longhitano and Ayres96, Reference Morgan, Parry and Arida104) and subjective fatigue(Reference Cunliffe, Obeid and Powell-Tuck105) in both healthy subpopulations. Thus, ATS-induced TRP:LNAA increases of 21–43 % have beneficial effects on memory functions, whereas higher increases impair memory, potentially due to sedative effects.
Effects of acute tryptophan supplementation on mood and cognitive functions in vulnerable subjects
Mood
In contrast to ATS effects on mood in healthy subjects, an increase in the plasma TRP:LNAA ratio of 41–48 % following ATS was sufficient to improve mood as well as vigor in high-stress-vulnerable subjects undergoing laboratory stress(Reference Markus, Olivier and Panhuysen93, Reference Markus, Panhuysen and Tuiten97). Other studies found that in women with premenstrual complaints, ATS (29 % increase in Trp:LNAA) improved mood during the premenstrual stage(Reference Sayegh, Schiff and Wurtman106, Reference Schmitt, Jorissen and Dye107). Furthermore, in 5-HTTLPR s-allele, but not l-allele, carriers, ATS (increase in plasma TRP:LNAA of 190 %) was found to have positive effects on mood and vigor after exposure to laboratory stress(Reference Markus and Firk108). It has also been reported that ATS (no data on TRP:LNAA levels) was without effect on mood in 5-HTTLPR s- and l-allele carriers, but lowered hypothalamic–pituitary–adrenal axis activity in individuals being homozygous for the s allele(Reference Cerit, Jans and Van der Does109). In addition, ATS reduced food intake in dieting females regardless of their 5-HTTLPR genotype, while increasing mood and emotional eating solely in l/l subjects (plasma TRP:LNAA ratio increased by 70 %)(Reference Markus, Verschoor and Smeets110). However, it has also been demonstrated that an α-lactalbumin-enhanced diet (21 % increase in TRP:LNAA) failed to modulate mood or vigor in recovered depressed subjects(Reference Merens, Booij and Markus98, Reference Booij, Merens and Markus100), and an increase of 21 % in TRP:LNAA following the administration of two α-lactalbumin-rich drinks had no effect on mood in recovered depressed patients(Reference Booij, Merens and Markus100). Perhaps these TRP:LNAA ratios of about 20 % are too low to improve mood in vulnerable subjects. Overall, ATS seems to have more profound effects on mood in vulnerable populations than in healthy subjects. Potentially, factors such as lower baseline levels of 5-HT and reduced capacity to clear released 5-HT from the synaptic cleft may help to clarify whether ATS has beneficial effects in individuals with mood disturbances.
Cognition
An increase of 21 % in TRP:LNAA following the administration of two α-lactalbumin-rich drinks improved abstract visual memory in both healthy controls and recovered depressed patients(Reference Booij, Merens and Markus100). Another study found that in women with premenstrual complaints, ATS improved memory for abstract figures (29 % increase in TRP:LNAA) and word recognition (6–25 % increase in TRP:LNAA) during the premenstrual stage(Reference Sayegh, Schiff and Wurtman106, Reference Schmitt, Jorissen and Dye107). Furthermore, Markus et al. (Reference Markus, Panhuysen and Jonkman111) reported an enhanced short-term memory scanning in healthy high-stress-prone subjects following laboratory stress and after consumption of a high-carbohydrate low-protein diet (TRP:LNAA ratio increased by 42 %) or after consumption of a diet enriched with α-lactalbumin (Trp:LNAA ratio increased by 43 %)(Reference Markus, Olivier and de Haan112). Together these data suggest that also in vulnerable subjects ATS improves memory-related processes, at rather low increases in plasma TRP:LNAA ratios. As to whether the high Trp-induced sedative effects on cognition as observed in healthy subjects also take place in vulnerable subjects needs to be investigated.
Effects of acute and (sub-)chronic tryptophan supplementation in animal models
As in the case of Trp depletion, the added value of studying Trp supplementation in animals lies in the ability to provide direct 5-HT level read-outs from the whole brain or specific brain areas and assess (sub-)chronic effects of Trp supplementation. Feurté et al. (Reference Feurté, Gerozissis and Regnault113) showed that chronic supplementation of the diet of rats with α-lactalbumin leads to a stable increase of 40 % in plasma TRP:LNAA. Increasing plasma TRP:LNAA levels in rats were found to increase brain 5-HT levels, as dietary sub-chronic Trp supplementation (30 % increase in brain TRP:LNAA) in Sprague–Dawley rats resulted in an increase of 92 % in extracellular 5-HT levels in the dorsal hippocampus. Moreover, the effect of the 5-HT-releasing agent fenfluramine was enhanced as was performance in a differential reinforcement of low rate responding condition (mimicking SSRI treatment)(Reference Stelt, Broersen and Olivier114). Collins et al. (Reference Collins, Kloek and Elliott115) showed that supplementation of an egg white protein hydrolysate replenished brain serotonin stores, even after pharmacological depletion of serotonin from the brain by 3,4-methylenedioxymethamphetamine(Reference Collins, Kloek and Elliott115). In Wistar rats chronic treatment with Trp by oral administration (about 60 % increase in plasma Trp:LNAA) was shown to increase 5-HT levels in the hippocampus by 57 %, in the cortex by 46 % and by 72 % in the rest of the brain, as well as boosting performance in the radial arm maze test and water maze test, indicating improvement in both short- and long-term memory(Reference Haider, Khaliq and Ahmed116, Reference Haider, Khaliq and Haleem117). However, a 7 d Trp treatment in mice suppressed food intake significantly and, more worryingly, increased brain thiobarbituric acid-reactive substances (a measure for oxidative stress)(Reference Coskun, Ozer and Gonul118). Taken together, these data show that it might be worthwhile to investigate whether the positive effects of ATS in human subjects could be maintained when diet is supplemented with Trp over a longer period of time. Although some care should be taken, the increase in oxidative stress following chronic Trp supplementation in mice should not be taken lightly.
Summary of acute tryptophan supplementation
ATS seems to improve mood and cognition in both healthy and vulnerable subjects. Effects of ATS on cognition are more consistent and require lower increases in the plasma TRP:LNAA ratios than needed to improve mood. Yet, for both mood and cognition it has been shown that too high increases in Trp levels result in poorer performances. As serotonin synthesis can theoretically only be increased to a maximum of 200 % due to saturation of TpH2 (see the acute tryptophan supplementation section), it is not likely that the poor performance results from local negative responses to elevated serotonin levels like sensitisation. However, a possible explanation could be sedation, which was reported in one study(Reference Cunliffe, Obeid and Powell-Tuck105), caused by the subsequent synthesis of melatonin from serotonin by serotonin-N-acetyltransferase and hydroxyindole-O-methyltransferase(Reference Hickman, Klein and Dyda119). Taken together, these data suggest that there is an inverted U-shaped curve for plasma Trp levels and mood/cognition. Finally, research in animal models has provided results that seem to justify further investigation of chronic Trp supplementation in human subjects.
Triple monoamine inhibitors
Unbeknownst to the individuals of ancient and medieval times, some of the medicinal herbs they used actually contained monoamine reuptake transporter inhibitors. During the previous decade a number of studies focused on the antidepressant-like effects of extracts from several subspecies of St John's wort (Hypericum perfoliatum), which was traditionally used to treat wounds, eczema and disorders of the central nervous system(Reference Bombardelli and Morazzoni120). Not only did these studies reveal replicable antidepressant effects in mice(Reference do Rego, Benkiki and Chosson121–Reference Viana, do Rego and von Poser124), analysis of monoamine uptake by rat synaptosomes showed that uptake of all three monoamines was inhibited by these extracts(Reference do Rego, Benkiki and Chosson121, Reference Viana, do Rego and von Poser124). In addition to St John's wort, the presence of monoamine reuptake inhibitors was shown in the extracts of Siphocampylus verticillatus (a Brazilian medicinal plant)(Reference Rodrigues, da Silva and Mateussi125), in Fructus Akebiae (a traditional Chinese cure for depressive disorders)(Reference Jin, Gao and Zhou126) and Sideritis scardica (used as a tea, flavouring agent and universal remedy around the Mediterranean)(Reference Knörle127).
Perhaps even more surprising is the presence of monoamine transporter inhibitors in regular foods. In 2003, a group of researchers reported on a group of isoflavans and isoflavenes, which are present in licorice, with serotonin transporter (SERT)-inhibiting properties(Reference Ofir, Tamir and Khatib128). After 7 d of oral injections with the extract of the roots of Glycyrrhiza glabra (from which licorice is made), mice showed enhanced learning and memory performance in the passive avoidance paradigm and reduced anxiety in the elevated plus maze(Reference Dhingra, Parle and Kulkarni129), as well as reduced immobility in the forced swim and tail suspension tests for depression(Reference Dhingra and Sharma130). These findings suggest that the extract from G. glabra possesses antidepressant potentials. Recently, Mechan et al. (Reference Mechan, Fowler and Seifert12) demonstrated that a supercritical CO2 oregano extract acting as a moderate noradrenaline, serotonin and dopamine reuptake inhibitor and MAO inhibitor exerted antidepressant-like and anxiolytic effects in mice. In the same study, the researchers used in vivo microdialysis in rats to confirm that extracellular 5-HT levels were indeed higher after a dose of the oregano extract, albeit more slightly compared with the antidepressant fluoxetine. Moreover, electroencephalogram measurements after acute oral supplementation in freely moving rats showed that this extract may exhibit antidepressant and neuroprotective activities(Reference Mohajeri, Goralczyk and Dimpfel131). Taken together, the results of these studies seem to justify further investigation into possible other dietary sources of monoamine reuptake inhibitors, as well as studies testing whether the beneficial behavioural effects of these plant extracts found in mice can be replicated in human subjects. An important fact to note is that the half maximal inhibitory concentration (IC50s) of these plant-derived monoamine reuptake inhibitors are about 1000-fold lower than those of pharmacological compounds, making the chances of detrimental side effects much lower(Reference Mechan, Fowler and Seifert12). In addition, a recent study investigating the attitudes of the general public and general practitioners towards enhancement of mood and cognition in healthy individuals by either pharmaceuticals or natural compounds showed that the public, especially, tends to be more favourable towards natural compounds(Reference Bergström and Lynöe132).
Summary and conclusion
While the serotonergic system is an important target for many pharmacological agents applied in psychiatry, this system is also amenable to influences of food. Indeed, food containing a low TRP:LNAA ratio tends to reduce mood and cognition, and food that increases this ratio may increase mood and cognition in humans. However, as we reviewed, the relationship between Trp intake and behavioural performance is not unequivocal. In part this is because methods that have been used to lower or increase the plasma TRP:LNAA ratio differ considerably across studies. Nonetheless, there may be an inverted U-shaped relationship between central 5-HT levels and optimal mood/cognition; low and too high Trp levels impair mood/cognition, and moderate to high Trp levels improve mood/cognition. Regarding cognition this relationship is found for both healthy and vulnerable subjects (Fig. 1(a)). However, regarding mood, effects of ATD and ATS seem to depend on the functioning of the serotonergic system of vulnerable or subclinical subjects, with a much more profound decrease in mood of vulnerable subjects compared with healthy subjects in the lower brain Trp range (Fig. 1(b)). However, if brain Trp levels are in the optimum range, mood in vulnerable subjects is comparable with mood in healthy subjects. In healthy individuals an increase in plasma TRP:LNAA of 67 % does not modulate mood, while in vulnerable subjects an increase of 20–50 % is sufficient to normalise their brain Trp levels and thus produce positive effects on mood. Such positive effects on mood are only seen in healthy subjects following a 191–255 % increase in plasma TRP:LNAA levels. When plasma Trp levels are elevated beyond that range, mood in both healthy and vulnerable subjects is negatively affected. Given that Western and new world societies are increasingly demanding and food is generally abundantly available in these societies, insight in beneficial TRP:LNAA ratios in food for specific target groups may help the nutrition industry to adapt food to support our mood and cognition.
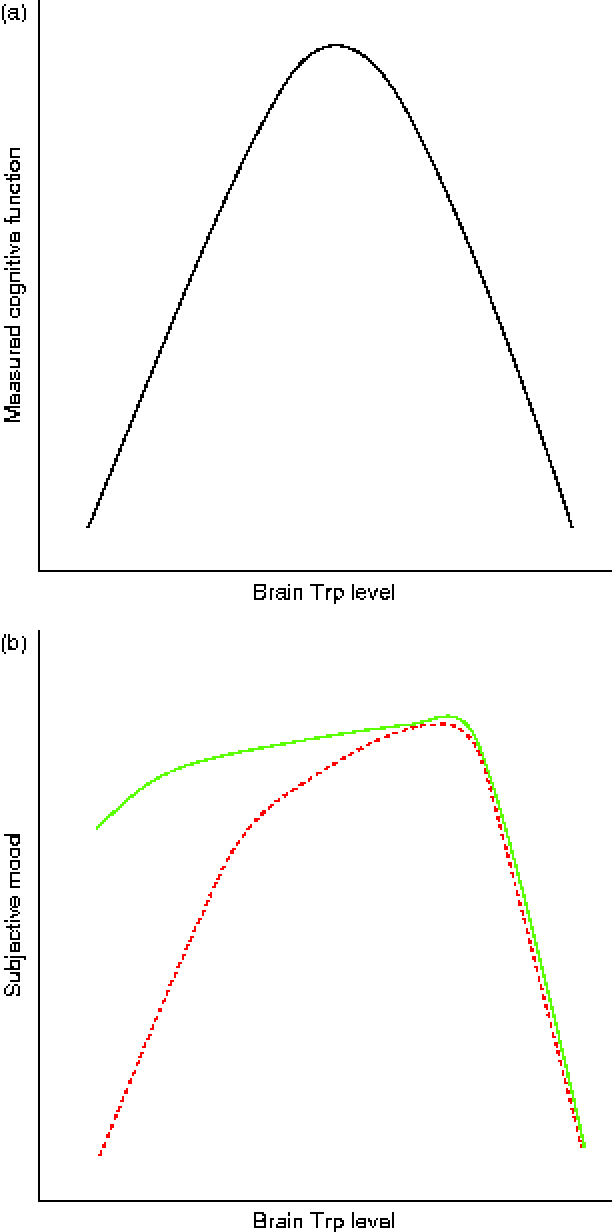
Fig. 1 Relationship between brain tryptophan (Trp) levels and cognition/mood. In both healthy and vulnerable subjects too low and too high brain Trp levels result in impaired cognitive ability. This indicates that in the case of cognition, brain Trp levels should lie within an optimum range (a). The effects of small increases or decreases in brain Trp levels on the mood of healthy subjects is negligible (b, ). Only large increases in brain Trp levels are able to improve mood significantly in these subjects. Conversely, in vulnerable subjects (b,
) relatively small increases in brain Trp result in an improved mood. Unphysiologically high increases in brain Trp lead to negative effects on mood in both healthy and vulnerable subjects. (A colour version of this figure can be found online at http://www.journals.cambridge.org/nrr)
One group of individuals that is vulnerable to manipulations of the serotonergic system is the one that carries the s allele of the 5-HTTLPR polymorphism. Studies employing 5-HTT knockout mice and rats as a model have revealed that reduced 5-HTT expression (as hypothesised for 5-HTTLPR s-allele carriers) is associated with increased extracellular 5-HT levels, reduced intra-neuronal 5-HT levels and increased 5-HT synthesis. Also, various 5-HT receptors are down-regulated(Reference Kalueff, Olivier and Nonkes32). As to whether Trp levels or its transport over the blood–brain barrier is affected by the 5-HTTLPR genotype is unknown to date. Nonetheless, if 5-HT synthesis is enhanced to compensate lower 5-HT tissue levels, it may not be surprising that 5-HTTLPR s-allele carriers are more sensitive to Trp depletion(Reference Neumeister, Hu and Luckenbaugh74), an effect that has also been observed in 5-HTT knockout rats(Reference Olivier, Jans and Korte-Bouws87). In contrast to ATD, ATS has a positive effect on mood, vigor and cortisol response after exposure to laboratory stress in ss subjects(Reference Markus and Firk108, Reference Cerit, Jans and Van der Does109). 5-HTTLPR s-allele carriers are more sensitive to stress(Reference Owens, Goodyer and Wilkinson133) and are more prone to develop depression after adverse life events(Reference Caspi, Sugden and Moffitt31). Given that ATS has been reported to dampen the negative effects on mood invoked by stress(Reference Markus and Firk108, Reference Cerit, Jans and Van der Does109), it is of interest to test whether (chronic) ATS improves stress coping and decreases risk for depression in s-allele carriers. Finally, to date, no studies on the effects of chronic Trp supplementation in human subjects have been conducted. With the recent discovery of powerful tools for enhancing available Trp through diet, such as hydrolysed protein or egg protein hydrolysate(Reference Mitchell, Slettenaar and Quadt8, Reference Markus, Firk and Gerhardt9), and promising data from ATS studies, chronic Trp supplementation studies in human subjects are warranted. Ultimately, enhancing available Trp in food such as cereals, maize and milk formulas may represent an efficient and cost-effective way of increasing mood and cognition, and thus our general well-being in the current increasingly demanding society. Specifically, insight into beneficial Trp:LNAA ratios in food for specific target groups – as presented here – may help the nutrition industry to increase the functionality of our food. Specifically, contrary to popular belief, most common foods high in Trp do not increase the plasma TRP:LNAA ratio enough to exert any positive effects on mood/cognition, because they also contain large amounts of other LNAA. Hence, functional foods have to be designed containing much higher levels of Trp compared with other LNAA. With about 10 % of the population over 12 years of age taking antidepressants in the USA alone(Reference Pratt, Brody and Gu134) and the numerous side effects associated with their use, consumption of these functional foods to prevent depression in the first place is alluring for both clinicians and the general public.
Acknowledgements
J. R. H. is financially supported by The Netherlands Organisation for Scientific Research (NWO), grant no. 86410003. NWO had no further role in the design of the study, in the collection, analysis and interpretation of data, in the writing of the report, and in the decision to submit the paper for publication.
S. H., A. M., M. H. M. and J. R. H. wrote the manuscript, S. H. organised the references and made the figures, J. R. H. designed the manuscript, and J. R. H. and M. H. M. together wrote the conclusions.
There are no conflicts of interest.