Introduction
Agricultural land is being turned into commercial facilities and thus lost to urbanisation as the world’s population continues to climb. As a result, a cost-effective technology that is best adapted to local conditions is urgently needed to boost the greenhouse company. Greenhouse drying, a novel method of drying food and agricultural produce, has the potential to reduce post-harvest losses and improve the quality of a wide range of products. The availability of exotic plants increased dramatically in the nineteenth century, resulting in the significant development of glasshouse cultivation in England and around the world (Shamshiri et al. Reference Shamshiri, Kalantari, Ting, Thorp, Hameed, Weltzien, Ahmad and Shad2018). Hobbyists, collectors, and home gardeners utilise larger greenhouses for agriculture and horticulture, together with botanical science, while hobbyists, collectors, and home gardeners use smaller structures. Greenhouse technology (GT) has been utilised in many regions of the world for over two centuries, but it was only recently adopted in Indian agriculture. Due to a greater emphasis on horticulture and the resulting rise in financial assistance, India’s greenhouse area has expanded significantly over the last decade. GT also has a unique application in areas where farming is unfeasible due to agro-climatic conditions. Greenhouses allow the crop-growing season to be extended in northern India’s chilly climate. There has been research into the impact of various greenhouse design aspects on energy conservation for environmental management. A metal structure with a transparent plastic or glass roof that allows sunlight to pass through is known as an agricultural greenhouse. The greenhouse microclimate is created inside the enclosed building to create an isolated atmosphere. The greenhouse production system, which plays a critical role in improving the management, quality, and quantity of agricultural outputs, has a significant impact on modern agriculture. Continuous greenhouse production system optimisation is essential to meet the increasingly stringent criteria of local and worldwide markets (Ma et al. Reference Ma, Carpenter, Maki, Rehman, Tuinstra and Jin2019). Microclimate variables prediction and control tasks, which directly affect crop development and yield, are the most important optimisation level in the hierarchical structure of the greenhouse production system. The total solar radiation, temperature characteristics, and inside flow pattern appear to be influenced by the shape and orientation of the greenhouse, in addition to wind direction, the features of the covering material, and the employment of insect-proof screens. One of the most important factors in the development of healthy societies is agricultural productivity. Temperature, humidity, and water stress are expected to become more prevalent in agricultural systems in the near future. As a result, the need to improve the efficiency of land and water usage has never been more persistent. The control and construction of greenhouses allow for significant improvements in crop quality and year-round production. A well-designed self-contained greenhouse may significantly minimise the number of fertilisers and energy used in agricultural production.
Design of greenhouse
A modern greenhouse is a framed building with glass or plastic that is used to grow flowers, vegetables, fruits, and other plants that require specific temperatures. The span-type greenhouse, which has a double-sloped, or A-shaped, roof, and the lean-to greenhouse, which has only one roof slope and leans on a building, are the two primary structural types. To reduce the number of external walls and thus lower heating costs, two or more span-type greenhouses are typically linked side by side. Because of the large expanses of windows on the sides and top, the plants in a greenhouse are exposed to natural light for much of the day (Kim et al. Reference Kim, Ryou, Kim, Lee, Oh, Lee and Kim2019). Glass has long been the most common glazing material, though polyethene or polyvinyl films, as well as fibreglass, are increasingly commonly utilised. The framework of the construction is made of aluminium, galvanised steel, redwood, cedar, and cypress wood. A greenhouse is heated partly by sunlight and partly by artificial means such as steam, hot water, or hot air. As a result, a greenhouse might get too hot or too cold, necessitating the deployment of a ventilation system. Roof apertures that may be regulated manually or automatically, as well as end-wall openings that electric fans use to extract air and circulate it throughout the interior, are examples of this.
Figure 1 illustrates the different greenhouse shapes provided for a controlled environment. Numerous greenhouse designs, including even-span, uneven-span, plastic, Quonset, flat arch, lean to, gothic arch, dome, slant leg, and gable greenhouses, are available for agricultural products. The most common greenhouse types used around the world are even span and Quonset. For successful production, a well-designed greenhouse with optimal space use and accurate environmental controls is required. Today, the Venlo region is one of the world’s biggest greenhouse markets, with Venlo-style greenhouses being popular in many parts of the globe. Greenhouses are divided into two types: free-standing and gutter-connected.
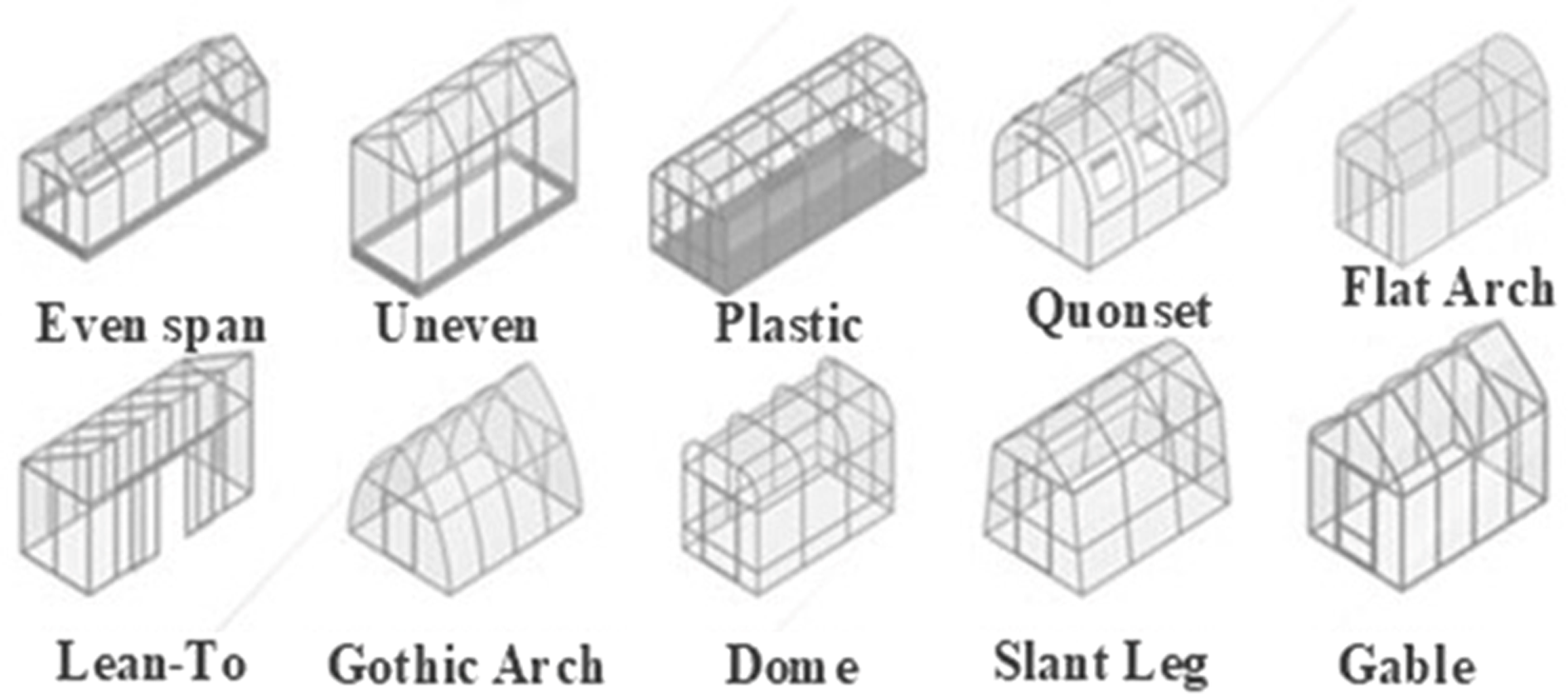
Figure 1. Different shapes of greenhouse.
Geometrical parameter and orientation of greenhouse
The direction of the greenhouse has a considerable impact on the transmission of solar radiation within the greenhouse during the winter season when solar radiation is limited. The geometry and slope of the greenhouse roof, as well as the latitude and season of the year, all determine the direction. The finest all-year-round orientation is stated to be east-west since it receives more radiation in the winter (keeping the indoor air temperature higher) and less radiation in the summer, which is excellent for composite conditions. Above 40°N, single greenhouses should have an east-west ridge to allow low-angle light to enter from the sides instead of the ends. Because the sun’s angle is significantly higher than 40°N, single greenhouse ridges should be positioned from north to south. The gutter’s shadow can travel across the greenhouse at this angle. The position and direction of the greenhouse should avoid casting shadows on nearby greenhouses. To avoid shading from one greenhouse to the next, these should be positioned east to west. It is necessary to consider the wind direction and latitude. Brun and Ville-o-de investigated the effect of orientation on the greenhouse environment in Mediterranean settings. They discovered that a north-south orientation in the greenhouse helped to preserve microclimatic constancy, whereas an east-west orientation harmed early development. Plants grown in greenhouses with a north-south orientation provided higher yields and profits.
This orientation also allowed for improved soil usage and aided in the greenhouse’s support against the prevailing northwest winds. At latitudes of 49.25° N, Chandra discovered that a standalone gothic arch-shaped greenhouse oriented east-west used 20% less energy than a greenhouse positioned north-south. Ibrahim Al-Helal et al. (Al-Helal et al. Reference Al-Helal, Picuno, Alsadon, Ibrahim, Shady and Abdel-Ghany2022) investigated the influence of greenhouse orientation on the rate of deterioration of cover optical characteristics by building greenhouse prototypes four in the shapes of two and covering them with a 200-m thick low-density polyethene film. Two types were grown north-south, while the other two were grown east-west, and all were treated to a year of drought. As a result, the shape and orientation of the small-scale greenhouses had no obvious effect on the rate at which the cover’s optical properties deteriorated. When planning a greenhouse, consider glazing materials, energy saving, ventilation systems, and sun orientation.
Environmental factors influencing greenhouse cultivation
While substantial quantities of energy are necessary for greenhouse temperature management to produce acceptable yields and high quality, energy consumption is one of the key cost issues in commercial greenhouses. Oil and natural gas are commonly utilised as heating fuels, adding to the greenhouse industry’s environmental effect. Depending on the site, the grown plants, the greenhouse covering, and the level of climate control, the energy required to heat 1 m2 of greenhouse space ranges from 500 to 2700 MJm-2 yr-1. Many initiatives have been made to reduce greenhouse gas emissions for climate management, while interest in renewable energy sources, such as wind and solar energy, has recently sparked. Solar thermal collectors are a sustainable energy source that may be utilised to provide low enthalpy thermal energy for greenhouse heating systems.
Solar radiation collector in greenhouse
Solar thermal systems provide a greater energy exchange ratio and are more cost-effective due to the fact of their cheap installation costs. Solar collectors are often made out of a series of black-painted copper pipes that sit within a thermally insulated box with a glass sheet on the front. The heated fluid travels through the collector and into a storage tank; the fluid can be cycled through the tank numerous times to raise the temperature of the fluid as much as feasible. Solar greenhouses, as a typical energy-saving horticultural facility, make anti-seasonal food production easier. Most countries are now adopting solar greenhouses for crop production. Solar radiation, the temperature within the greenhouse, its location, the covering material, its shape, and its slope are all factors that determine how effectively it functions. One of the most critical factors affecting greenhouse performance is solar ray intensity. Because of the greenhouse structures and covering materials, solar energy is wasted. Absorption and scattering by the greenhouse cover material, as well as dust on the greenhouse cover material, diminish the intensity of solar radiation. Solar radiation transmittance is affected by both the greenhouse’s orientation and the sun’s elevation. The greenhouse roof slope is kept at the same angle as the latitude of the location. Flexible photovoltaic (PV) panels are a renewable energy source with a wide range of applications.
Flexible PV panels are a sustainable energy source that might be suitable for greenhouse agricultural cultivation, especially in warm, sunny climates. The objective of the RalAroca-Delgado et al. (Aroca-Delgado et al. Reference Aroca-Delgado, Pérez-Alonso, Callejón-Ferre and Díaz-Pérez2019) investigation was to see how flexible solar panels installed on the greenhouse roof affected tomato plant shape, the number of fruits per plant, and quality during spring and early summer. This research was carried out in a commercial greenhouse for tomato production throughout two growing seasons. On the greenhouse roof, two solar panel arrays were examined. The ideal design and orientation of seasonal solar radiation transmitted within the greenhouse were identified by AbderrahmanMellalou et al. (Mellalou et al. Reference Mellalou, Riad, Mouaky, Bacaoui and Outzourhit2021). The solar energy transmitted was computed using the greenhouse cover’s transmittance as a function of incidence angle (polycarbonate (PC)). Anhua Liu et al. (Liu et al. Reference Liu, Xu, Henke, Zhang, Li, Liu and Li2022), who examined the 3D CSG model and a detailed crop canopy model altered the light environment within CSG. The simulation was thoroughly validated using the Liao-Shen type Chinese Solar Greenhouse (CSG-LS) as the prototype. The sun radiation intercepted by the maintenance buildings and crops was used to calculate the assessment index. Mohammed Aissa et al. (Aissa & Boutelhig Reference Aissa and Boutelhig2021) assessed greenhouses in outside climatic conditions, taking into account wind velocity and solar trajectory. The findings showed that reorienting the tunnel greenhouse structure, or the design of the tunnel greenhouse structure used in the dome and chapel shapes, had a significant impact on the temperature difference between the interior and outside air temperature of the greenhouse. Mr Aditya Arvind Yadav and colleagues (Yadav et al. Reference Yadav, Oturkar and Deshmane2021) look at the design aspects of a solar-modified greenhouse prototype. Using a solar-adapted greenhouse prototype, experimental validation for drying of crops and fruits supported by phase change material (PCM) as thermal energy storage is possible.
Wind-driven natural ventilation in greenhouse
In new greenhouse construction and greenhouse retrofits, natural ventilation is becoming more common. The concept of cooling a greenhouse with thermal buoyancy and wind extends back to the dawn of the controlled environment. All greenhouses built had vents or louvres that could be opened to let hot air out and cool air in. A fan-cooled greenhouse’s summer ventilation capacity should be 0.58 to 0.65 metres of floor space. This will provide around one volume air change every minute, keeping the temperature differential between the louvre end and the fan end between -13.8 to -12.2°Celsius. There are horticultural and ornamental crops growing there, and natural ventilation is one of the most common aspects of greenhouses in this area. Natural ventilation, as opposed to forced ventilation, is a simple, low-cost method that requires little maintenance (McCartney et al. Reference McCartney, Orsat and Lefsrud2018). With the correct design and implementation, temperature and humidity excesses can be controlled, CO2 levels can be kept close to those of the outside environment, and pollination can be promoted within a greenhouse. Using a transient CFD-2D model, Edwin A. Villagran et al. (Villagran et al. Reference Villagran, Romero and Bojacá2019) evaluated natural ventilation in three types of greenhouses: a traditional Colombian wood greenhouse and two alternative forms of multi-span greenhouses with larger ventilation surfaces on the sides and roof.
Yicun Hou et al. (Hou et al. Reference Hou, Li, Li, Jin, Tian, Zhang, Wu, Zhang and Lei2021) used three greenhouses in northwest China as research subjects. Field research looked into microclimate elements such as internal air temperature, relative humidity, and sun radiation under natural ventilation. Ahmad Tusi et al. (Tusi et al. Reference Tusi, Shimazu, Ochiai and Suzuki2021) compared the Heat Balance and Water Vapour Balance methods with the tracer gas method as a reference to analyse the diurnal change in ventilation rate over the summer and early fall seasons. Offor Justus Nwafor et al. (Offor 2022) studied the impact of natural ventilation on the interior comfort of residents of Public Hospital wards in Nigeria, where the climate is classified as Hot-Humid. The purpose of this research is to determine how natural ventilation impacts people’s interior comfort in public hospital wards in the Hot-Humid zone.
Effect of greenhouse-covered materials
Seasonal fluctuations in energy and heat demand in summer (in the south) and winter (in the north) influence greenhouse material choices (in the north). As a consequence, they do not block out photosynthetically active light (PAR), and high transmittance coefficient materials are ideal for making greenhouse materials in the winter. In contrast, high PAR coefficient materials are inappropriate for use in the summer owing to the risk of overheating and the requirement for alternate cooling methods such as evaporative cooling. Thermal efficiency and optical qualities are important material features for greenhouse cladding because they determine radiation management, heat exchange, UV, soil, and IR absorption or transmittance. Glass, films, double or single glazing, and, plastic sheets are used to cover greenhouses. Fibreglass greenhouse cladding materials are the least transmissivity and offer complications in the recycling process. They are, nonetheless, appropriate for covering greenhouse structures in hot and arid climates to reduce energy loss and preserve interior cooling. Plastic sheets and films conversely have a greater NIR transmission. In comparison to glass, plastic materials give the least protection against night-time IR radiation. Correspondingly, glass structures are appropriate for plants that are sensitive to infrared light. Alternatively, while fibreglass has adequate mechanical qualities, it has the least desirable optical features, such as low light transmissivity.
The limited lifespan of plastic films used to cover greenhouses in dry places, which are heavily impacted by extreme environmental conditions, is a critical issue. The most often used plastic films have a lifespan ranging from one growing season to two to three years. Solar irradiance, extremely high temperature and humidity, wind, rainfall, and sand storms, as seen in the Arabian Peninsula desert, together with chemical compounds used in greenhouses, cause their degradation. Abdulaziz Alharbi et al. (Alharbi et al. Reference Alharbi, Campen, Sharaf, de Zwart, Voogt, Scheffers, Tsafaras, Abdelaziz, Babiker, Gruda and Qaryouti2021) investigated the effects of three different greenhouse-covering materials, PC, clear glass, and diffuse tempered glass, on cucumber production, water use efficiency, and energy use efficiency. The results suggest that compartments with diffused or transparent glass have greater water or light consumption efficiency than those with PC. Hyung-Kweon Kim et al. (Kim et al. Reference Kim, Lee, Kwon and Kim2022) studied and investigated the microclimate and thermal environment within single-span greenhouses coated with a single layer of plastic film, PC, and glass. The PC-covered greenhouse proved to be the best option for controlling the evening heating impact during the cold season, according to the findings of the trial. The glass-covered greenhouse was determined to be the best for controlling the cooling impact throughout the hot season.
In the visible and near-infrared, Eylul Simsek et al. (Simsek et al. Reference Simsek, Zhu, Kashanchi, Williams, Galy, Marszewski, Tolbert and Pilon2021) investigated the effect of suspended droplets formed on the back of semi-transparent glass panes on their normal-hemispheric transmittance and reflectance. Nefise Yasemin Tezcan et al. (Tezcan 2020) assessed heating requirements and fuel costs (fossil and geothermal energy) for certain regions in the Aegean and Mediterranean areas using long-term weather data. Choi, Kyung Yun, and colleagues (Choi et al. Reference Choi, Kim, Bae, Yoon, Yun and Kim2020) looked into the impact of a greenhouse-covering material on the interior atmosphere and the characteristics of cherry tomatoes growing in the greenhouse. The typical polyethene film on the greenhouse roof was replaced with a PC sheet, but the basic structural structure was left intact. Pahola Thathiana Benavides et al. (Benavides et al. Reference Benavides, Lee and Zare-Mehrjerdi2020) examined the life cycle analysis of bio-Polyethene and biodegradable polylactic acid plastics from feedstock production through product disposal to evaluate how they influence the environment.
Materials and methods
The typical method for climate control is to configure the static set point in actual greenhouse production, and the energy will be wasted because the static set point cannot be automatically adjusted according to the external environment. To solve this problem, the greenhouse climate control method based on greenhouse agrivoltaics was studied. Microclimate is the complex of environmental variables, including temperature, radiation, humidity, and wind, to which the plant is exposed. Thermal energy storage using UTES and PCMs has been identified as a potential solution to achieve considerable energy savings in greenhouse heating/cooling. This review investigates the latest technological advancements in greenhouse heating/cooling systems.
Solar greenhouse agrivoltaic system
To establish a sustainable future and reduce greenhouse gas emissions, a varied spectrum of sustainable energy sources will be critical. As the competition for land between food and energy production becomes more intense, interest in the co-use of land for both agricultural and energy production has grown. In that event, scientists have devised a novel modelling approach for evaluating the effectiveness of semi-transparent PV systems in greenhouses. Their unique method takes into account the irradiance’s broadband and spectrum content, solar cell technology and performance parameters, and the link between photosynthetic rate and effective photon flux falling on the crops. Agrovoltaics, which installs solar panels on farms to maximise the synergy between PV energy and agriculture, is establishing itself as one of the standards for making a sector that does not want to be left behind in the battle against climate change more sustainable. Efficiency is also a significant reason why agrivoltaic systems are favoured over traditional PVs. Agri-PVs improve the efficiency of solar panels while also benefiting the soil since it has been demonstrated that specific crops can be cultivated among traditional solar farms to aid with water flow and prevent the area from becoming barren which is depicted in Figure 2. The technological characteristics of APV systems are continually increasing, and they vary by country and location (Zainol Abidin et al. Reference Zainol Abidin, Mahyuddin and Mohd Zainuri2021).

Figure 2. APV system.
PVs presently generate about 50 GW of power in India. Solar plants with a capacity of 1059.64 MW in India can absorb 1,600,000 tonnes of CO2 per year and produce 10,000 tonnes of agricultural product per year, employing 2000 people. Bottle gourd, lady finger, watermelon, and spices like ginger, turmeric, and Chile are used in India’s agrivoltaic systems. PV modules in APV systems are installed at an angle that corresponds to the latitude of the area. The spacing between two PV modules is critical to avoid panels shadowing other panels. The panels are set at an appropriate height above the ground. In the space between two panels, various crops can be grown. Crop selection is important, though, because some crops require a lot of sunlight. Choose a crop that requires the least amount of sunlight to reduce production loss. Approximately 49% of the land might be used to raise food and generate renewable energy. It will increase farmer revenues by 1500%, according to many studies.
Greenhouse agrivoltaics in protected cultivation
Agrivoltaics is a concept that may be used in both open fields and protected agriculture (e.g. greenhouses, polytunnels). Greenhouses contribute considerably to increased global food and ornamental production by providing a proper microclimate for plants, allowing for greater plant development, a longer production period, environmental protection, and larger and higher-quality harvests. In the recent decade, many ways of combining crop growing with PV power production in the same greenhouse structure have been documented in the literature (e.g. Zhang et al. Reference Zhang, Yan, Wang, Jia, Wang and Klemeš2022; Magadley et al. Reference Magadley, Kabha, Dakka, Teitel, Friman-Peretz, Kacira, Waller and Yehia2022). Agrivoltaic systems can help meet the growing need for food and energy while also reducing water usage. PVs put atop greenhouses, for example, might effectively cover energy demands for power and heat. Various studies have been conducted in China, Spain, Italy, the United States, France, Germany, and India to reduce or partially replace energy use by placing PV modules on specific portions of agricultural greenhouse roofs or ground to preserve plants by reducing solar radiation, light intensity, and air temperature within the greenhouses.
On a global scale, Eduardo F. Fernandez et al. (Fernández et al. Reference Fernández, Villar-Fernández, Montes-Romero, Ruiz-Torres, Rodrigo, Manzaneda and Almonacid2022) investigated the energy production of APV systems in agriculture greenhouses. In four typical places with a large crop cultivation greenhouse installation, this is projected for 15 sample plant cultivars from five unique major socioeconomic crop families, namely Cucurbitaceae, Fabaceae, Solanaceae, Poaceae, and Rosaceae. The solar power output and development of Brassica rapa subsp. Chinensis L. at a 25-kW PV power plant was compared to the control by Manoch Kumpanalaisatit et al. (Kumpanalaisatit et al. Reference Kumpanalaisatit, Setthapun, Sintuya and Jansri2022) With a crop yield of 2.28 kW, the PV generated the most electricity. Ruth Anne Gonocruz et al. (Gonocruz et al. Reference Gonocruz, Nakamura, Yoshino, Homma, Doi, Yoshida and Tani2021) studied a variety of factors that affect rice crop yield, including fertiliser application, temperature, and solar radiation, all of which were directly observed and evaluated to see if changes were linked to PV systems erected above rice farms. According to studies, the highest shading rate for agrivoltaics installations is between 27 and 39%, preserving at least 80% of the rice output.
Thermal energy storage system for GT
Many commercial glass greenhouses or hothouses are state-of-the-art food or flower production facilities. To maximise plant development circumstances, the glass greenhouses are fitted with screening systems, heating, cooling, and lighting, all of which may be controlled by a computer. Temperature and humidity management are critical for increased crop yield in greenhouses, which consume a lot of power. The primary goal of the project is to employ PCM to lower the overall energy consumption of the greenhouse used to manage the interior climate for increased crop output. In addition, the article develops PV cells to create power. When used in conjunction with a solar air heater, thermal energy storage is one of the most efficient methods of storing solar energy for air heating. Thermal storage material is used in solar air heaters to heat air before and after sunset. Solar air heaters are used to dry agricultural products, store crops in warehouses, and for industrial dyeing, dairy, and chemical applications. The intermittent power supply is eliminated with thermal storage systems. Solar air heaters with thermal storage devices have lowered the costs and volume of a single product.
To address the intensive energy consumption of these production facilities, energy-saving technologies paired with alternative energy sources have been emphasised as a feasible option in agricultural greenhouses. A. Bazgaou et al. (Bazgaou et al. Reference Bazgaou, Fatnassi, Bouharroud, Elame, Ezzaeri, Gourdo, Wifaya, Demrati, Tiskatine, Bekkaoui and Aharoune2020) investigated and documented the efficacy of combining two technologies for heating a Canarian greenhouse: rock-bed thermal energy storage and water-filled passive solar. The results show that the combined heating system may boost the night-time interior air temperature by 3-5°Celsius on clear days and 2-3°Celsius on cloudy/rainy days with fewer fluctuations throughout the winter. Shiva Gorjian et al. (Gorjian et al. Reference Gorjian, Ebadi, Najafi, Chandel and Yildizhan2021) look into how renewable energy technologies, such as solar PV, solar thermal, biomass, and geothermal, can be integrated with greenhouse production systems like net-zero energy greenhouses. The following are the main thermal energy storage technologies used in various heating and cooling applications:
Underground Thermal Energy Storage (UTES)
A huge, undetectable, and isolated storage capacity can be provided by underground soil and/or rocks. UTES systems use this volume’s heat capacity to store thermal energy from any natural or artificial source for seasonal or diurnal uses. UTES is a potential based on greenhouses producing additional heat in the summer and requiring heating in the winter. Although UTES may offer both heating and cooling, some systems only deliver one of the two. The three main components of UTES systems are the thermal storage system, the thermal exchanging unit (not required indirect heating), and the thermal distribution system. J.G. Gluyas et al. (Gluyas et al. Reference Gluyas, Adams and Wilson2020) employ aquifers and flooded mines to evaluate the theoretical potential capacities for heat storage in the subsurface, with a focus on seasonal heat storage. Aquifer thermal energy storage (ATES) is the free cooling or heating of an aquifer’s natural groundwater basins, as seen in Figure 3. Due to its parametric nature, Ruben Stemmle et al. (Stemmle et al. Reference Stemmle, Blum, Schüppler, Fleuchaus, Limoges, Bayer and Menberg2021) provide a unique life cycle assessment (LCA) regression model that may be employed for a broad range of ATES setups. This model is a quick replacement for traditional, time-consuming LCAs. To aid global technology adoption, Paul Fleuchaus et al. (Fleuchaus et al. Reference Fleuchaus, Schüppler, Bloemendal, Guglielmetti, Opel and Blum2020) examined the dangers of HT-ATES. Although HT-ATES is less adjustable than competing systems such as pits or buffer tanks, the main difficulties are heat supply loss and increasing or decreasing heating needs.
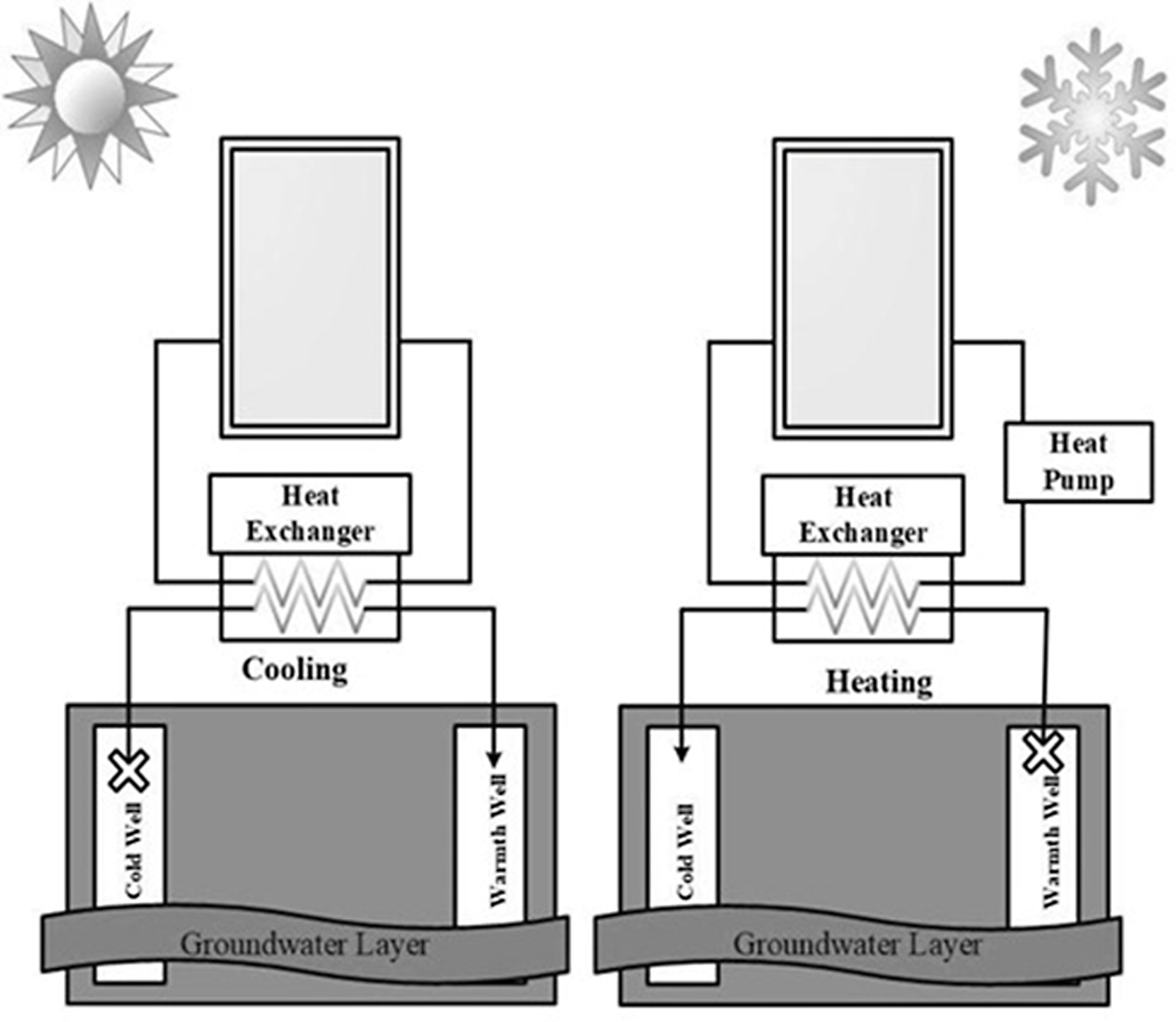
Figure 3. Aquifer thermal energy storage system.
If no appropriate aquifer exists, or if it is not possible to use an existing aquifer owing to laws, Borehole Thermal Energy Storage (BTES) can be a viable option for thermal energy storage. Thermal energy is transmitted to the subsurface via conductive flow from a series of closely spaced boreholes in a BTES system. By altering drilling depth and spacing while keeping the volume and number of boreholes constant, Seokhwa Hwang et al. (Hwang et al. Reference Hwang, Yun and Heo2020) study the impacts of internal energy on the system. Over three years of computations, the internal energy stored ranged from 1528.91 GJ to 1618.69 GJ. Diesel generators are often used to provide communal electricity in remote areas with little or no connection to the power grid. In this case, in an isolated Canadian town, Seyed Ali Ghoreishi-Madiseh et al. (Ghoreishi-Madiseh et al. Reference Ghoreishi-Madiseh, Fahrettin Kuyuk, Rodrigues de Brito, Baidya, Torabigoodarzi and Safari2019) suggest coupling a diesel generator’s heat recovery unit to a borehole seasonal thermal storage system to store rejected heat during the summer and giving improved heat when required during the winter season. In this way, BTES might be a solution to the seasonal misalignment of (renewable) energy consumption and production.
Man-made buildings are the alternative option for UTES. When the current aquifer and subsoil conditions are unfavourable, these are utilised. They’re known as cavern thermal energy storage, and they span a wide range of subsurface ‘cavities.’ Pascal Lalanne et al. (Lalanne & Byrne 2019) presented a hybrid approach with a low CapEx cost and a medium-high RTE (60–70%) by utilising the dependability and efficiency of pumped-hydro equipment. To assess their UGS potential for CAES applications, David Evans et al. (Evans et al. Reference Evans, Parkes, Dooner, Williamson, Williams, Busby, He, Wang and Garvey2021) employed an approach developed during the EPSRC-funded IMAGES project, whose equations were confirmed using operational data from the Huntorf CAES station. Filtering the estimates based on cavern dimensions and storage cycles, as well as UGS projects and operational modes, yields more realistic storage estimates of tens of TWh from theoretical storage with over 300 TWh.
Greenhouse with PCM
Energy storage in acceptable forms that can be traditionally transformed into the needed form is a current technological problem. The procedure of PCMs is one of the potential ways of storing thermal energy. Notan Kumar et al. (Kumar et al. Reference Kumar, Gupta and Sharma2021) discuss the use of PCM for thermal energy storage and how to design new PCM with higher performance and safety. The wide variety of applications of PCM, such as electronic, biomedical, textile, construction, and automotive sectors, has been reviewed, and this study can aid future research in the domain of PCM in thermal energy storage. Zaineb Azaizia et al. (Azaizia et al. Reference Azaizia, Koolz, Hamdi, Elkhal and Guizani2020) published experimental results on a unique mixed-mode solar greenhouse drying system with and without a thermal energy storage unit in PCM. During the night, the air temperature inside the solar greenhouse with PCM is around 7.5°Celsius higher than conventional driers, according to the testing results.
In the applications of cold thermal energy storage, PCM technology offers a lot of potential for energy storage and cost savings. Marcot and Ramkunwer explored the use of PCM inclusion in a concentrated solar power system numerically and found differences in PCM behaviour due to thermophysical parameters. Yusufoglu conducted the same tests on four different types of PCMs in a refrigeration system. They stated that 0.95 kg of PCM saved 9.4% of energy and increased compressor running time in a variety of refrigerator systems. However, there are no options for energy delivery when grid power is unavailable. Solar electricity is the greatest method to offset the absence or shortage of grid energy in India, based on climatic conditions. It follows that P. Sarafoji et al. (Sarafoji et al. Reference Sarafoji, Mariappan, Anish, Karthikeyan and Reddy2021) demonstrated the performance and assessment of PCM in the PSCS prototype system. According to the results of the experiment, 16 kg of PCM could be charged in 75-90 minutes and kept the temperature within the specified range for an average of 150 minutes.
Auxiliary heating system in greenhouse
Most of the time, modern solar greenhouses do not need to be heated, but they require supplemental heating when the weather is severely cold or gloomy. A thermal energy system is also installed in the greenhouse, which employs an underground water tank to store and transfer heat absorbed by solar radiation or produced by an auxiliary heat pump via subsurface Utube heat exchangers. The greenhouse is equipped with an auxiliary heat pump system that utilises energy to compensate for solar input inadequacy at night or during periods of low solar radiation. Burning caves have been progressively employed in greenhouses as an extra heating option. On the contrary, failure to manage the burning rate can result in dangerously high temperatures, uneven temperature distribution, and other heating problems. In this manner, controlling the burning rate is critical to preventing these issues.
There are various articles on using a heat pump to heat a greenhouse, but the experiments below, which use solar heat as an auxiliary heat source, are typical of heat pumps and mixed central heating. Hassanien et al. (Hassanien et al. Reference Hassanien, Li and Tang2018) investigated a heat pump system for greenhouse heating that used a vacuum tube-type solar collector as an auxiliary heat source. In October, March, and April, the interior temperature for heating was set at 14°Celsius, and it was feasible to cover 62%, 40%, and 78% of the needed heating load, respectively. The most efficient technique to manage the burning pace in a burning cave should be to adjust the different forms of smouldering. Henceforth, Z. Xu et al. (Xiu et al. Reference Xiu, Nie, Chen, Yan, Cai, Liu and Wei2019) investigated the airflow distribution in a blazing cave. The realisable k-turbulence model and the non-equilibrium wall function were used to model the turbulent airflow. Additional auxiliary heating sources can be used in solar dryers to adjust the external factors that impact the drying process (inlet air temperature, airflow rate, relative humidity, and heat input). Wang et al. tested a mango drying system that included solar air collectors and auxiliary heat pumps. Later, Samuel N. Ndirangu et al. (Ndirangu et al. Reference Ndirangu, Kanali, Ronoh, Langat, Roskilly, Royapoor and Laidler2022) tested the performance of Power Roll Limited’s (UK) low-cost, flexible, and lightweight solar PV film for food drying applications.
Efficient operation of greenhouses in a controlled environment
One-third of global greenhouse gas emissions come from the food production sector. Accordingly, food production centres are frequently located far from customers, 30% of the world’s food perishes while en route. Greenhouse crop production, as one kind of controlled environment agriculture (CEA), is a critical component of meeting the world’s rising food needs. A greenhouse’s regulated atmosphere enables crop production in locations and seasons when growth would otherwise be impossible, extending the growing season of seasonal crops. This permits crops to be produced close to where they will be consumed. CEA boosts output, shortens transportation routes, saves water and land, and eliminates the need for pesticides. These benefits are substantial, but sustaining the environmental conditions required for plant development necessitates temperature, humidity, light availability, and water use management are depicted in Figure 4. In that case, greenhouses utilise a lot of energy in comparison to other agricultural industries.
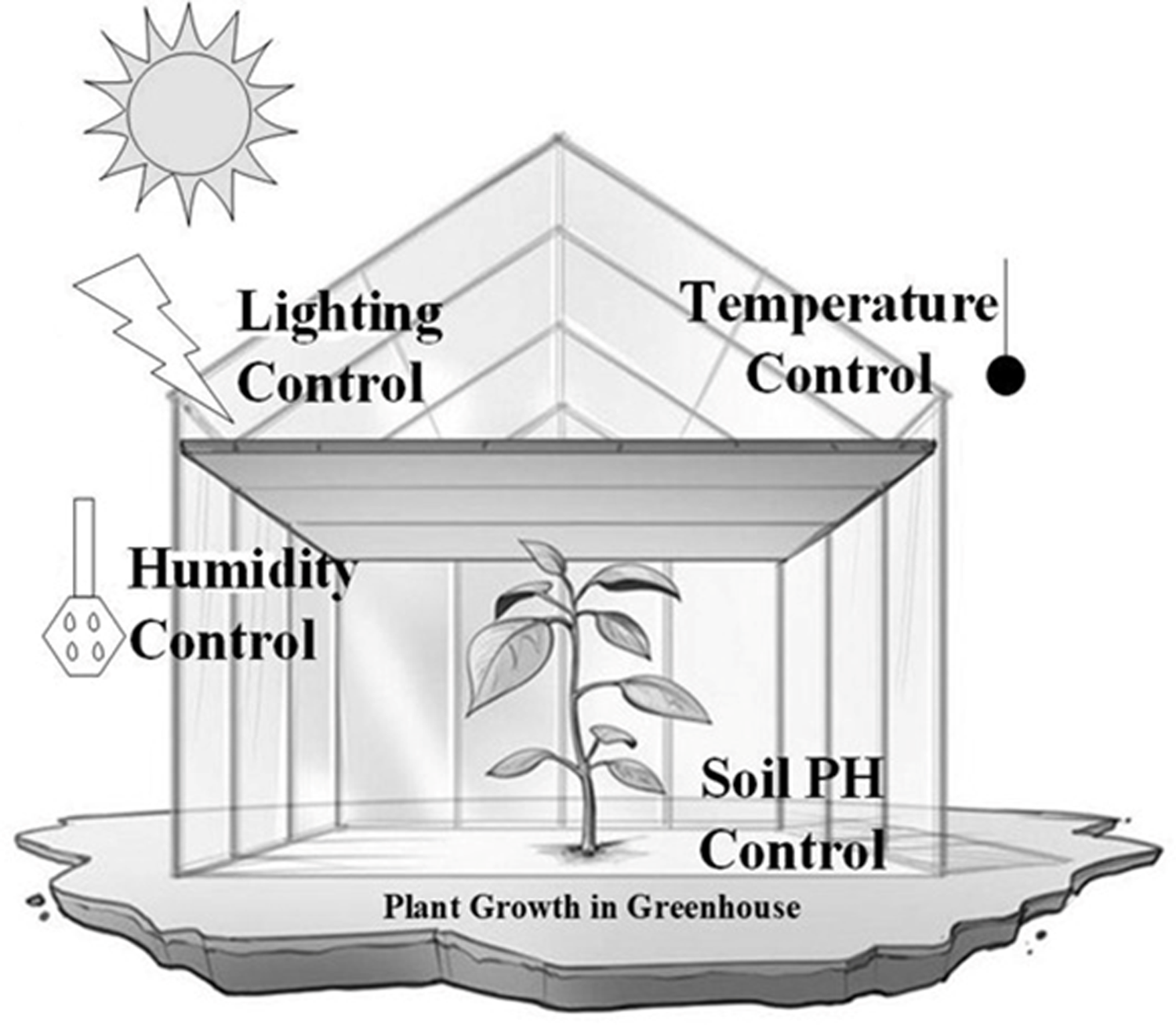
Figure 4. Climatic factors affecting greenhouse plant growth.
Temperature is the most important initial variable in the greenhouse environment that can and must be controlled, according to the research. The majority of greenhouse plants are warm-season species that thrive at temperatures ranging from 17 to 27ºC, with lower and upper-temperature limits of 10 and 35ºC, respectively. Humidity, which is commonly expressed in terms of relative humidity, is the second important variable in the greenhouse environment. If the relative humidity exceeds 95% for an extended period, especially at night, serious problems may arise because fungal infections are easily transmitted. The third variable is Ph, which regulates the mobility of these nutrients depending on the substrate (Engler & Krarti Reference Engler and Krarti2021). Micronutrients become more mobile and are absorbed in greater quantities than the plant requires when the pH is too low, resulting in toxicities. Micronutrients are less mobile when the pH is too high, and the plant cannot absorb enough, resulting in shortages. This indicates that the plant is under pH stress. A greenhouse farmer’s objective is to keep the pH of the crop consistent throughout its life cycle. The predictive controller forecasts the future values of process variables using a model of the process. The controller then adjusts the climatic variables in the greenhouse based on these forecasts.
Temperature control in greenhouse
Greenhouse horticulture has been widely utilised across the world to lengthen agricultural growing seasons and protect crops from adverse weather conditions. The greenhouse climate has a tremendous impact on the quality and production of crops and vegetables, with greenhouse temperature being one of the most critical aspects of plant growth and the major source of energy consumption. To enhance the control performance of contemporary greenhouses utilising model-based predictive control systems, an environment model that can accurately anticipate greenhouse microclimate is required. R. Liu et al. (Liu et al. Reference Liu, Li, Guzmán and Rodriguez2021) proposed a transient greenhouse model that estimates temperature and humidity in typical Chinese solar greenhouses using a mechanistic technique. Instead of utilising boundary temperature data, the environment model used an innovative and easy-to-use wall temperature estimating approach based on the energy balance. To predict the inside temperature of a greenhouse, Wentao Cai et al. (Cai et al. Reference Cai, Wei, Xu and Ding2021) proposed the Gradient Boost Decision Tree (GBDT) model, which is based on the recently established Light Gradient Boosting Machine approach (LightGBM or LGBM). In the end, air vent management should take into account local weather conditions in addition to the appropriate temperatures inside the structure. Wentao Cai et al. (Briga-Sá et al. Reference Briga-Sá, Paiva, Lanzinha, Boaventura-Cunha and Fernandes2021) proposed the GBDT model, which is based on the recently established LightGBM approach or LGBM.
Evaporative cooling is also achieved by the use of wet walls and fans, with cooled air pulled through the wet wall. Evaporative cooling combined with ventilation is a well-known way of lowering high air temperatures. The spatial fluctuations of microclimatic conditions in enclosed North-South (N-S) oriented single-arched greenhouse PC structures with a wet wall providing evaporative cooling at the S end were explored by Michael J Savage et al. (Savage Reference Savage2014). Andrea Costantino and colleagues proposed a modelling technique for predicting the energy consumption of mechanically ventilated greenhouses for climate management. The strength of the suggested energy model is its integrated approach to simulating greenhouse dynamics, which takes into account the building’s dynamic thermal and hydric behaviour along with the dynamic reaction of planted crops to variations in solar radiation.
Optimal temperature set points for greenhouse
Greenhouse production procedures show that the greenhouse climatic setpoint has a substantial influence on greenhouse energy costs and crop yield. As a consequence, dynamically tweaking greenhouse temperature setpoints can boost crop output while simultaneously lowering the greenhouse’s total energy use. With the help of adjusting the greenhouse, climate setpoint is a cost-effective strategy to conserve energy. The temperature integration hypothesis states that crop development is mostly influenced by the cumulative temperature over time rather than the transitory temperature. Consequently, if it can maintain a particular accumulated temperature over some time, crop development will not be severely impacted. This means that if the weather is cold, a low-temperature setpoint is preferable, and the loss of accumulated temperature can be compensated on warm days. In this case, it simply optimises the cumulative temperature or means temperature over a specific period to generate the temperature setpoint. Many setpoint optimisation approaches have been presented in recent years based on this concept. Jones, for example, described a method for determining greenhouse daily temperature using the TOMGRO tomato growth model with the POLY-2 greenhouse climate model.
Good, dynamically calculated greenhouse temperature setpoints boost crop production while simultaneously lowering the greenhouse’s total energy usage. Long-term weather is the main source of uncertainty for establishing setpoints. Yuanping Su et al. (Su and Xu Reference Su and Xu2021) proposed a multi-layer hierarchical optimisation approach for greenhouse climate setpoints to address this uncertainty. The entire production cycle is separated into multiple crop development phases, each of which lasts several days, and this framework contains two optimisation layers with differing timelines. Because the recommended technique optimises the stage mean temperature and daily mean temperature rather than the greenhouse environment setpoint. Later, Yuanping Su et al. (Su et al. Reference Su, Xu and Goodman2021) introduced a technique that employs a surrogate-aided multi-objective optimisation algorithm to get the ideal mean temperatures of the crop growth phases while minimising energy consumption and maximising crop output. Henceforth, the suggested technique does not optimise the setpoint directly, a serialisation method is provided to convert the mean daily temperature into the interior temperature setpoint.
Greenhouse temperature control with ventilation
Controlling the greenhouse environment necessitates a variety of systems to have a specific impact on internal climatic factors. Ventilation and heating are the most common systems; however, sophisticated greenhouses can also employ contemporary technologies like carbon dioxide injection or artificial lighting. Even still, applying automation control to greenhouses is a difficult task. Meanwhile, they continually create undesired changes in the interior climatic variables, and external weather conditions should be included as disturbances in the formulation of the control issue. Using a natural ventilation system, Ana Paola Montoya-Rios et al. (Montoya-Ríos et al. Reference Montoya-Ríos, García-Mañas, Guzmán and Rodríguez2020) created a control approach to manage the indoor air temperature of a greenhouse during the day. The control strategy employs a Proportional-Integral controller in conjunction with feedforward compensators to improve performance against measurable external disturbances such as outside air temperature, solar radiation, and wind velocity. A. Hoyo et al. (Hoyo et al. Reference Hoyo, Moreno, Guzman and Rodríguez2019) suggested a control approach based on the feedback linearisation technique and quantitative feedback theory to regulate the interior diurnal temperature in a greenhouse in the south of Spain. Vita Ayu Kusuma Dewi et al. (Dewi et al. Reference Dewi, Setiawan, Waspodo and Liyantono2020) study the relationship between climatic conditions within and outside the greenhouse, the influence of microclimate on evapotranspiration, and the ability to forecast evapotranspiration inside the greenhouse. The evapotranspiration value follows the climate condition due to the varied environment within and outside the greenhouse. The study was carried out in a natural-ventilated organic produce greenhouse. According to the findings, the daily air temperature within the greenhouse was greater than the outside temperature.
Humidity control for greenhouse crops
The use of particular engineering facilities and technology to specific plant growth and development requirements to improve or produce environmental meteorological elements is referred to as facility agriculture. This creates a favourable environment for plant development, minimising dependency on the natural environment to a degree. The primary goal of greenhouse horticulture is to protect crops from adverse weather and illnesses. It’s a complicated system that, in recent years, has evolved into a technique for achieving regulated agricultural production by maintaining a precise temperature. Climate, water resources, and energy resource exploitation all have a significant impact on agricultural productivity. As a result, the expansion of the agricultural sector needs the introduction of new technologies that emphasise economic efficiency and the most efficient use of limited resources while having the least possible environmental effect. Keeping the greenhouse dry is the most cost-effective method, especially when the temperature drops at night. The water on the greenhouse floor evaporates, as does the water on the leaves and growth media surfaces, bringing moisture to the greenhouse. Evaporation dampens the air and consumes energy that could be utilised to keep the house warm. Sebastian-Camilo Vanegas-Ayala et al. (Vanegas-Ayala et al. Reference Vanegas-Ayala, Barón-Velandia and Leal-Lara2022) studied the many connections in fuzzy inference systems now used for the modelling, prediction, and control of humidity in greenhouses, as well as how they have changed over time, to develop more robust and intelligible models. Barkat Rabbi et al. (Rabbi et al. Reference Rabbi, Chen and Sethuvenkatraman2019) find climate control strategies for protected cropping in places where cooling technologies combined with humidification or dehumidification are often used. Based on clumped and oxygen isotope analyses of pedogenic siderites, Joep van Dijk et al. (Van Dijk et al. Reference Van Dijk, Fernandez, Bernasconi, Caves Rugenstein, Passey and White2020) reconstructed continental temperatures and precipitation oxygen isotope compositions (reflective of specific humidity). Tae Cheol Seo et al. (Seo et al. Reference Seo, Kim, Kim, Cho, Choi, Ryu, Shin and Lee2022) investigated the effect of high-temperature ventilation on powdery mildew, silver leaf whitefly, and two-spotted spider mite management. On bright days, the temperature within the greenhouse was raised to the specified temperature and maintained for roughly 9 hours at 45° Celsius with high relative humidity.
Lighting control in greenhouse environment
Supplemental illumination has the potential to improve plant development in CEA, particularly in greenhouses. However, the power required for supplementary lighting might account for up to 30% of a greenhouse’s total running expenditures. Subsequently, several CEA researchers have lately focused on increasing the cost-effectiveness of lighting through better management systems. The dimming feature of light-emitting diodes (LEDs), which allows the controller to change the output light to practically any continuous level, was explored in several lighting systems. The DynaLight system, an on/off control technique for high-intensity discharge (HID) lights, was invented by Qu et al. (Afzali et al. Reference Afzali, Mosharafian, van Iersel and Mohammadpour Velni2021a). To lower power costs while achieving a minimal daily total of photosynthesis, this system takes into account a leaf photosynthesis model, variable electricity price, and weather prediction. The supplementary lighting management problem in greenhouses equipped with HID lights is formulated as a discrete restricted optimisation problem by Shirin Afzali et al. (Afzali et al. Reference Afzali, Mosharafian, van Iersel and Velni2021b). This model attempts to reduce supplementary lighting power costs by taking into account solar forecasts, plant light requirements, and variable electricity prices.
The adaptive lighting technique is another rule-based control solution that saves money by altering the duty cycle of LEDs to meet a pre-determined threshold based on photosynthetic photon flux density (PPFD) levels. Although the constraint of the optimisation issue is to ensure sufficient light for healthy crop development, it is important to execute the strategy in the greenhouse and evaluate plant growth through experimental investigations. Simultaneously, Shirin (Afzali Reference Afzali2021) proposed the best supplementary lighting strategy and used IoT technology to apply it in a research greenhouse. The growing need for food has piqued academics’ interest in using Internet of Things (IoT) technologies in agriculture. The IoT is a network of physical objects that connect through the Internet. In CEA, the IoT has reduced human labour, time, and cost while increasing yield. The IoT includes wireless sensor networks, radio-frequency identification, cloud and edge computing, and human-computer interaction.
PH control in greenhouse environment
Soil is an important part of life support systems because it provides a variety of products and services that have positive or negative effects on human well-being, such as water control, carbon storage, food production, and soil fertility, including pH. Soil pH is one of the most important factors in determining whether or not nutrients are available in the soil. The availability of secondary macronutrients is reduced at lower pH levels, whereas the availability of micronutrients in the soil is limited at higher pH levels. Furthermore, the efficacy of insecticides, plus the usage of organic and inorganic fertiliser sources in the soil, needs a correct pH for optimal plant uptake. Be a result, soil pH is referred to as a ‘primary soil indicator’ that influences biogeochemical cycles additionally the soil microbial population. Mineral techniques for changing soil pH have shown to have downsides, such as being too difficult to modify. Accordingly, adjusting the rhizospheric pH might be a viable option. In doing so, Aqarab Husnain Gondal et al. (Gondal et al. Reference Gondal, Hussain, Ijaz, Zafar, Ch, Zafar, Sohail, Niazi, Touseef, Khan and Tariq2021) indicated that a microbial-breeding technology such as microbe genome replication may be a good way to change rhizospheric pH. It’s conceivable that the genetic product of the bacterium releases too many acidic or basic substances, which raise or lower the pH of the rhizosphere.
Experiments were conducted on two types of soil textures to investigate the addition of varying amounts of sulphuric acid by irrigation to reduce soil pH and enhance the availability of the elements on cucumbers, according to Hemn Othman Salih et al. The results show that rising sulphuric acid reduced the pH of the soil regularly. However, there is just limited research on the pH of irrigation water used in hop cultivation. According to this, Joao de Jesus Guimaraes et al. (de Jesus Guimarães et al. Reference De Jesus Guimarães, de Sousa, Román, Dal Pai, Rodrigues and Sarnighausen2021) investigate the influence of irrigation with various pH ranges on the agronomic growth of hops cultivated in a greenhouse in Botucatu, Sao Paulo, Brazil. Because of climate change, increased salt in water and soil has harmed plant functioning and lowered plant yield, posing a danger to future food security. Consequently, Mohammad Javad Ahmadi-Lahijani et al. (Nabati et al. Reference Nabati, Ahmadi-Lahijani, Goldani, Nezami, Oskoueian, Hosinaiyan and Mohammadi2021) hypothesised that varied pH values (unadjusted as control (8.5-9), 5.5, and 4.5) would reduce saline impacts on tomato plants (cv. Mobil). The plants with the highest fruit fresh weight and plant dry weight were those cultivated at pH 5.5.
Greenhouse climate control models /software
Modelling the greenhouse with and without the heating and cooling systems was done using a variety of simulation tools. When engineers and researchers need to do numeric technical computing, they commonly use Matlab. Matlab was used to create a script that calculated the total solar energy gained by the greenhouse for each month using a mathematical model. Matlab/Simulink is also used to predict the temperature of the storage water, the temperature within the greenhouse, and the quantity of auxiliary fuel. The region’s location, geometry, and meteorological data were all taken into account. Moreover, a web-based greenhouse environment simulator was created to demonstrate the physics of greenhouse systems by allowing the user to model changes inside the greenhouse environment based on input factors such as climate, structure, and environmental control options with the help of several studies, TRaNsient SYstem simulation programme (TRNSYS) used for greenhouse simulations. It is frequently used for the simulation and evaluation of solar energy system performance because of the ease with which a user can interlink the library component models. One of the modules is linked to a meteorological data file to model greenhouse thermal behaviour and forecast greenhouse heating and cooling loads (Ahamed et al. Reference Ahamed, Guo and Tanino2020). This module allows one to select the greenhouse’s geometrical properties, and also the greenhouse-covering material, orientation, and window and door placement TRNSYS has been used in various studies involving energy analysis and greenhouse thermal simulation.
Results and discussions
Numerous studies were performed to develop greenhouse thermal models to describe cooling processes in a greenhouse microclimate. The indoor environment was controlled by attaching the greenhouse with either active/passive heating or cooling system like mass storage, buried pipes, natural ventilation, shading devices, evaporative cooling, geothermal energy, etc. Accordingly, the review analyses the influencing factors of greenhouse construction. The greenhouse construction shields the crops from rain and wind while allowing sun radiation to flow through the walls, allowing for heat exchange with the outside world. This section presents the comparison results of various control techniques with advantages and disadvantages in the greenhouse model to describe the inside climate. In addition, the article presents the control factors of temperature, humidity, and lighting-based techniques findings with simulation tools under various technologies.
Agriculture confronts the enormous task of producing enough food and other essentials for a growing global population, which is expected to reach nine billion people by 2050. There is limited room for arable land growth, and the increasing danger of climate change to agriculture in the form of unpredictable weather, floods, and other catastrophic occurrences makes the challenge of feeding the world’s population much more difficult. Agriculture is defined as the cultivation of plants for food, provender, oils, and industrial use. Plants from several families are used in this activity, including grasses, vegetables, edible and attractive flowers, legumes, oilseeds, industrial crops, medicinal plants, and others. All of these plant groupings are produced in soil and open fields, with some of them being protected by protective coverings, hence the term ‘Protected Agriculture’. The creation of a structure that allows for the proper management of environmental conditions, which is the core notion of generating this study, is one of the most significant challenges encountered by greenhouse designers. A greenhouse is a metal-framed agricultural structure used for plant culture and/or protection that is covered in a translucent plastic film to keep the rain out. The greenhouse construction shields the crops from rain and wind while allowing sun radiation to flow through the walls, allowing for heat exchange with the outside world. Hydroponics is often used in greenhouse agriculture, which is also known as CEA. Hydroponics is a cultivation technique for managing the nutrients of the crop. Hydroponics is now the most widely used crop production method in the agricultural business. Table 1 illustrates the comparison of different control strategies in the greenhouse environment. The optimal functioning of the photosynthetic and transpiration processes of the plants may be achieved by managing the microclimate, resulting in increased agricultural yields.
Table 1. Control strategies used for microclimate factors affecting greenhouse

Nonlinear, strong coupling, multi-input and multi-output, and dynamically changing factors define the greenhouse microclimate, making it challenging to govern. Predictive control, fuzzy control, nonlinear feedback control, robust control, optimum control, and supervisory control are just a few of the greenhouse microclimate environmental controlling approaches that have been developed. Temperature, relative humidity, light intensity, carbon dioxide (CO2), and electrical conductivity/pH of the nutrient solution in the irrigation system are the most significant parameters or variables to consider and regulate in a hydroponic automated greenhouse at different phases of growth. The approaches for managing the temperature in the greenhouse environment are shown in Table 2.
Table 2. Techniques used for temperature control in greenhouse

Table 3 defines the humidity control major techniques in the greenhouse. Later of their superior productivity and resource efficiency, closed horticulture production systems have recently gotten a lot of attention. However, as previously stated, these systems need microclimate control and lighting, both of which are energy-demanding. Furthermore, these buildings are airtight and lack natural ventilation, evapotranspiration from crops tends to raise humidity levels, necessitating continual dehumidification.
Table 3. Techniques used for humidity control in greenhouse
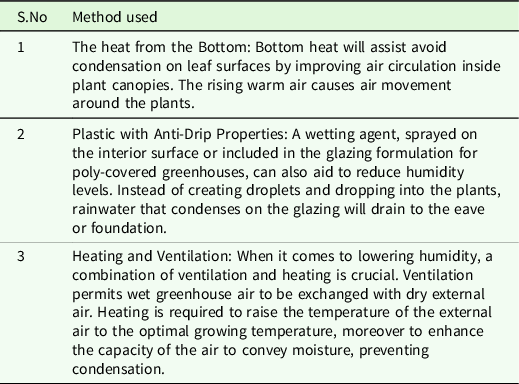
Table 4 illustrated the major lighting factor in greenhouse design. Previous research on supplementary lighting management with LEDs took one of two approaches: employing real-time sunlight intensity measurements to deliver pre-set PPFD levels or assuming prior knowledge about sunlight intensity throughout the day. HID lamps (especially high-pressure sodium lamps) and LED lamps are the two main types of horticulture lighting.
Table 4. Major lightings used for greenhouse environment

Climate control technologies should be chosen under the farmed crop and local climatic circumstances. When forced/mechanical ventilation systems are used to boost and sustain ventilation rates throughout the greenhouse, it may not be enough to drop the internal temperature to achieve the needed levels during peak summer conditions. As a result, it should be used in conjunction with other cooling solutions. In hot and dry climates, evaporative cooling methods such as fan pads and fog cooling are excellent ways to manage the climate. During the summer, electricity generated by a hybrid system comprising grid-connected solar PV panels and a heat pump may meet 33.2-67.2% of the greenhouse requirement. The air temperature in greenhouses may be reduced by up to 12°Celsius using a ground cooling system. The following Table 5 gives a general overview of the simulation tools (ANSYS Fluent, COMSOL, MATLAB, TRNSYS) utilised in greenhouse investigations.
Table 5. Simulation tools used for modelling greenhouse

To summarise, the majority of the above models are validated and created under certain conditions, such as covering qualities and infiltration. For the sake of simplicity, the evapotranspiration of the crops and the CO2 content of the greenhouse air were ignored. However, there is no universal model that can accurately capture greenhouse behaviour. All effective parameters should be considered in a credible thermal model. The mathematical model should be able to precisely forecast the greenhouse’s thermal behaviour by taking into account all of the relevant characteristics, such as geometrical specifications, orientation and form, and climatic conditions. According to a review of numerical greenhouse modelling in hot areas, CFD is a useful method for estimating airflow, temperature, and humidity distributions inside the greenhouse. Protected agriculture has underperformed in recent years by the reason of a scarcity of competent workers and delayed technology advancements. The use of intelligent greenhouses to increase crop quality and quantity allows for accurate manipulation of the greenhouse’s environmental parameters in connection to the crop’s life cycle within the control regulations.
Conclusions
Improvements in greenhouse construction, cladding materials, and insulating techniques, as well as innovative climate control equipment and the integration of physical and physiological knowledge into operational climate control systems, have all aided the development of energy-efficient greenhouse systems. Several researchers employed diverse methodologies such as evolutionary algorithms, genetic algorithms, dynamic modelling, and numerical modelling to analyse and optimise greenhouse climatic conditions in hot regions. The review paper presents an up-to-date survey of the literature on greenhouse systems. The following are the results of the survey. This review provided an in-depth analysis of conventional greenhouse designs added to the accompanying technical development of control systems for usage in climates with varying climates.
Additionally, utility-driven demand response and peak load control are taken into account using optimisation-based building energy modelling and simulation tools. This would be beneficial in the development and maintenance of a resilient and long-term built environment. For greenhouse climate control, there are a variety of systems, methods, strategies, and models. Climate control should be centred on crop activities in the future and towards greenhouse sustainability. Considering the effectiveness and quick response time, numerous simulation tools have been effectively utilised to control the greenhouse inside temperature. To offer a favourable microclimate for different types of crops, greenhouse systems can be fitted with numerous solar or energy storage devices. CFD models are effective simulation tools for investigating the velocity and temperature fields inside the greenhouse. The agrovoltaics system model will be used to generate the most profit/power by utilising farming land for developing flowering plants coupled to generate electricity. Using APV we can increase the land productivity. The development of new novel systems (PV system technology) and components (PV device technology) can improve the energy performance of some APV greenhouse design options. The manufacturing quality will be triggered by greenhouse technologies with regulated settings. The intricate interplay between the interior and outside factors is the key issue that should be considered for greenhouse control. This tendency has necessitated the employment of various elements, tools, materials, and structures for crop protection to achieve higher-quality goods. According to the findings, more advanced user-friendly tools that incorporate both design and control-oriented improvements of building energy systems for crop production are needed.
Acknowledgement
Not Applicable.
Financial support
This research received no specific grant from any funding agency, commercial or not-for-profit sectors.
Competing interests
The author(s) declare none.