Introduction
Members of Osedax are well known for relying, with the aid of symbiotic bacteria, on sunken vertebrates for their nutrition (Rouse et al., Reference Rouse, Goffredi and Vrijenhoek2004; Goffredi et al., Reference Goffredi, Orphan, Rouse, Jahnke, Embaye, Turk, Lee and Vrijenhoek2005). Initially found living on whale bones (Rouse et al., Reference Rouse, Goffredi and Vrijenhoek2004; Glover et al., Reference Glover, Källström, Smith and Dahlgren2005; Fujikura et al., Reference Fujikura, Fujiwara and Kawato2006), it has subsequently been shown that Osedax can live off the exposed bones of a range of vertebrates, including teleost fish (Jones et al., Reference Jones, Johnson, Rouse and Vrijenhoek2008; Rouse et al., Reference Rouse, Goffredi, Johnson and Vrijenhoek2011, Reference Rouse, Goffredi, Johnson and Vrijenhoek2018; McClain et al., Reference McClain, Nunnally, Dixon, Rouse and Benfield2019). While one of the early descriptions, by Fujikura et al. (Reference Fujikura, Fujiwara and Kawato2006), reported that Osedax japonicus was found living ‘on the surfaces of tainted spermaceti’ no details were provided. There have been no subsequent reports of extant Osedax living on any other substrate apart from bone, though the unusual Osedax jabba is known to live off buried fragmented pieces of bone buried in the sediment (Rouse et al., Reference Rouse, Goffredi, Johnson and Vrijenhoek2018). With reference to fossil evidence for Osedax activity that is not bone-related, Kiel et al. (Reference Kiel, Kahl and Goedert2013) showed bore holes and cavities within the teeth of an Oligocene toothed mysticete whale. Amalfitano et al. (Reference Amalfitano, Giusberti, Fornaciari, Dalla Vecchia, Luciani, Kriwet and Carnevale2019) also suggested that circular boreholes in the fossil vertebrae of the shark Cretoxyrhina mantelli, dating to the Upper Cretaceous, could represent the action of Osedax. However, evidence to support this via internal microCT scans as performed on other fossils to support the presence of Osedax borings (Kiel et al., Reference Kiel, Goedert, Kahl and Rouse2010; Danise & Higgs, Reference Danise and Higgs2015), were lacking.
Osedax obtain nutrition via the symbiotic heterotrophic bacteria that live in their roots, which are in contact with the bone matrix. The inorganic component of the bone (calcium hydroxyapatite) is dissolved by acid secreted by the roots (Tresguerres et al., Reference Tresguerres, Katz and Rouse2013) allowing access to the organic components. The food source was initially hypothesized to be the major organic components of bone such as proteins (e.g. collagen) and hydrocarbons (e.g. cholesterol) (Goffredi et al., Reference Goffredi, Orphan, Rouse, Jahnke, Embaye, Turk, Lee and Vrijenhoek2005). Subsequent studies narrowed this down to the food source likely being collagen (Goffredi et al., Reference Goffredi, Johnson and Vrijenhoek2007, Reference Goffredi, Yi, Zhang, Klann, Struve, Vrijenhoek and Brown2014; Miyamoto et al., Reference Miyamoto, Yoshida, Koga and Fujiwara2017).
Given the apparent reliance on Osedax and their symbionts on collagen it seems reasonable to examine non-bone marine food sources that they might exploit. Collagen is a primary component of the cartilage of non-bony fishes such as elasmobranchs (Merly & Smith, Reference Merly and Smith2013). Parts of shark cartilaginous skeletons, such as the jaws, may be mineralized as tessellated cartilage (Balaban et al., Reference Balaban, Summers and Wilga2015), allowing resistance to rapid degradation by scavengers. It was hypothesized that this would provide time for colonization and growth of Osedax, which can occur in less than 2 months (Rouse et al., Reference Rouse, Worsaae, Johnson, Jones and Vrijenhoek2008). Also, the teeth of sharks contain various forms of dentin (or dentine), a bone-like matrix with an organic content that is largely collagen (Enax et al., Reference Enax, Prymak, Raabe and Epple2012). The dentin of the tooth is surrounded by an extremely hard enameloid ‘crown’ that does not extend over the tooth root (Salomon, Reference Salomon1969; Enax et al., Reference Enax, Prymak, Raabe and Epple2012; Jambura et al., Reference Jambura, Türtscher, Kindlimann, Metscher, Pfaff, Stumpf, Weber and Kriwet2020). Osteodentine is porous, cellular dentin, harder than bone and is found in the root (base) of all shark teeth (Whitenack et al., Reference Whitenack, Simkins, Motta, Hirai and Kumar2010). The teeth of most sharks and rays have hollow pulp cavities surrounded by another form of dentin, orthodentine, beneath the enameloid. However, in the clade Lamniformes (and some rays) the pulp cavity is missing, and space beneath the enameloid is filled with osteodentine. These latter teeth are referred to as osteodont or pseudoosteodont (Whitenack et al., Reference Whitenack, Simkins, Motta, Hirai and Kumar2010; Schnetz et al., Reference Schnetz, Pfaff and Kriwet2016; Jambura et al., Reference Jambura, Türtscher, Kindlimann, Metscher, Pfaff, Stumpf, Weber and Kriwet2020).
Rouse et al. (Reference Rouse, Goffredi, Johnson and Vrijenhoek2011) reported the deployment of the jaw and several vertebrae of a juvenile mako shark (Isurus oxyrinchus, Lamniformes) for 5 months at ~1000 m depth, but the vertebrae and jaws had disintegrated except for some calcareous elements. The teeth were intact and showed no signs of Osedax. Here we report on a deployment of jaws of the common thresher shark (Alopias vulpinus, Lamniformes) for 8 months, and document the occurrence of two known Osedax species using shark teeth as a food source.
Materials and methods
Heads of Alopias vulpinus had been donated to the NOAA Southwest Fisheries Science Center, La Jolla, California by anglers as part of the Oceanside Anglers' Club thresher shark sport fishing tournament in May 2018. Frozen heads were then provided for this project. Three jaws were dissected, two lower and one upper (Figure 1A) and placed in mesh bags and then into a half of a minnow trap that was weighted (Figure 1B), in order to prevent the teeth from being buried in the sediment, as in a previous deployment of mako shark jaws. The trap was deployed near a whale fall ‘Francisco’ at 1018 m depth in Monterey Submarine Canyon (36.77°N 122.08°W) by the ROV ‘Doc Ricketts’ from the R/V ‘Western Flyer’ on 13 December 2018, dive 1105, and recovered on 8 August 2019, dive 1172. Upon collection the remains of the jaws and teeth were removed from the mesh bags and examined for Osedax traces with a Leica S8APO stereomicroscope with a Pentax WG-III handheld camera. Some teeth were dissected open at this point and then fixed and preserved in 95% ethanol. All remains of the jaws and all other teeth including some with Osedax traces were also fixed and preserved in 95% ethanol. These samples were examined further with a Leica MZ9.5 stereomicroscope and photographed with a Canon Rebel T6s camera. A dwarf male found in the tube of one Osedax specimen was photographed with a Leica DMR compound microscope. Osedax specimens were subsampled for DNA analysis and the remaining tissue accessioned into the Benthic Invertebrate Collection at Scripps Oceanography. Mitochondrial cytochrome c subunit I (COI) sequences were obtained following methods outlined previously (Vrijenhoek et al. Reference Vrijenhoek, Johnson and Rouse2009).
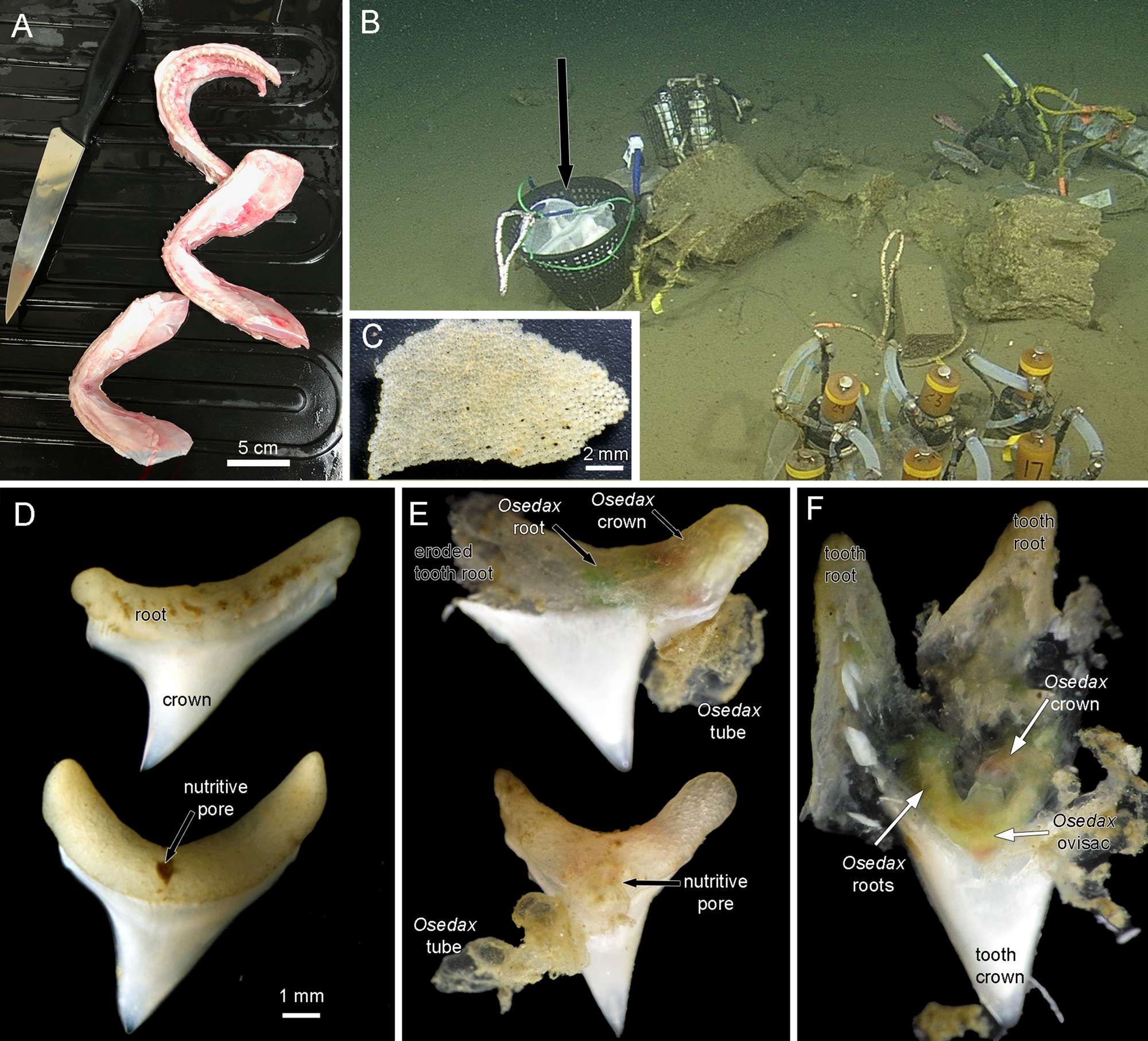
Figure 1. (A) Jaws of thresher shark Alopias vulpinus prepared for deployment. (B) Jaws in mesh bags in a weighted basket (black arrow) deployed near a whale fall at 1018 m depth in Monterey Submarine Canyon. (C) Tessellated cartilage fragment from mesh bags recovered after 8 months on the sea floor. The hyaline cartilage had disappeared. There were no obvious signs of Osedax activity on these fragments. (D) Most of the ~40 recovered teeth were intact. (E) Two teeth photographed on the initial recovery before fixation. Each has an obvious Osedax inside the tooth root and an emergent tube. (F) A tooth root dissected on the initial recovery showing a live Osdax, probably O. talkovici. Most of the dentin pulp has been digested, though the enameloid crown of the tooth was untouched.
Results
There was no trace of any hyaline cartilage after the 8 months on the seafloor. There were some fragments of the outer layer of tessellated cartilage that showed no traces of Osedax (Figure 1C). Of the ~40 teeth that were recovered most showed no obvious traces of Osedax (Figure 1D). These intact teeth, which had a width of up to 8 mm across the root section, showed a distinct nutritive pore on one side (Figure 1D). Ten teeth were found to have obvious Osedax occupying the root area of the tooth and we obtained COI sequences for six of these (GenBank OQ814198-OQ814203), identified as either Osedax packardorum or O. talkovici. Several teeth that were observed and photographed on the initial recovery (Figure 1E) showed the typical gelatinous tubes of Osedax attached to the root portion. The Osedax specimens had all retracted, with colours visible inside the tooth root suggestive of Osedax roots (green) and crown (red). The outer surfaces of the tooth roots were relatively intact (Figure 1E) but on dissection of one specimen there was no little to no dentin visible inside the root, or the crown of the tooth (Figure 1F).
In one case there was a cluster of four teeth bound together (Figure 2A) and all four showed evidence of Osedax (Figure 2B) in their respective roots. One of these specimens was sequenced for COI (GenBank OQ814199) and was found to be Osedax packardorum Rouse et al., Reference Rouse, Goffredi, Johnson and Vrijenhoek2018, with a 98.6% similarity to the sequence of the holotype (EU223341). Another tooth dissected in the laboratory showed a tube emerging from the side of the tooth root (Figure 3A). On dissection, the tooth was largely empty, except for a female Osedax with a distinctive yellow patch (Figure 3B), like that seen in Osedax talkovici Rouse et al., Reference Rouse, Goffredi, Johnson and Vrijenhoek2018. The COI sequence for this specimen had a 99.4% similarity to the sequence of the holotype of Osedax talkovici (MG262313). Four other Osedax talkovici were also found in other teeth (GenBank OQ814200-OQ814203). The tube of the female specimen in Figure 3A, B was examined, and a single dwarf male was present (Figure 3C, D). The enameloid crowns of the teeth were unaffected with no trace of Osedax borings.

Figure 2. Preserved thresher shark teeth with Osedax. (A) A cluster of four teeth that were stuck together. One obvious Osedax tube is present. (B) The four teeth separated, and each was occupied by an Osedax. The specimen labelled Osedax packardorum, missing its crown of palps, was sequenced (GenBank OQ814199).

Figure 3. Preserved thresher shark tooth with Osedax. (A) Both sides of a tooth with the root filled with an Osedax specimen and an emergent tube on one side. (B) The Osedax female dissected from the tube with the yellow patch found on one side of the trunk that is distinctive is Osedax talkovici. The specimen was sequenced (GenBank OQ814198). (C) The tube had a single dwarf male present. (D) The dwarf male viewed with a compound microscope showing sperm, spermatids and posterior hooks.
Discussion
This study provides the first direct evidence for Osedax to exploit a substrate other than bone for nutrition. The worms appear to have used the inner parts of the shark teeth, composed of osteodentine and orthodentine (Goto, Reference Goto1991; Whitenack et al., Reference Whitenack, Simkins, Motta, Hirai and Kumar2010). The root surfaces were largely intact with erosion only near the Osedax tubes. This appears to be a general strategy of Osedax, where surface regions of bone are left alone while the roots ramify inside (Rouse & Goffredi, pers. obs.). It was notable that one tooth at least was penetrated by Osedax via the nutritive pore (Figure 1E), though based on the position of the tubes, other Osedax accessed the interior via other parts of the tooth root, such as the lateral region (Figures 1E, 2B & 3A). Dentin has a similar hardness to bone and its organic matrix is also largely composed of collagen (MacDougall & Javed, Reference MacDougall, Javed, Bronner, Farach-Carson and Roach2010). The dentin of shark teeth is about 20% organic matrix (Enax et al., Reference Enax, Prymak, Raabe and Epple2012), comparable to that seen in sperm whales (Brault et al., Reference Brault, Koch, Gier, Ruiz-Cooley, Zupcic, Gilbert and McCarthy2014) and humans (LeGeros, Reference LeGeros1981). This makes dentin an obvious food source for Osedax if accessible. The teeth of Alopias vulpinus studied here had roots that obviously could be penetrated by Osedax. In a previous experiment using the jaws of a juvenile mako shark (Isurus oxyrinchus), deployed at a similar depth, no Osedax occurrences were found after 5 months of deployment (Rouse et al., Reference Rouse, Goffredi, Johnson and Vrijenhoek2011). Both these sharks belong in Lamniformes and so have similar osteodont teeth with the crown full of dentin and no pulp cavity (Jambura et al., Reference Jambura, Türtscher, Kindlimann, Metscher, Pfaff, Stumpf, Weber and Kriwet2020). It may be that Osedax larvae simply did not recruit to the mako shark teeth during the deployment period, since they fell to the bottom of the deployment trap and may have been buried, while the teleost vertebrae in the experiment were secured above the sediment (see Figure 1A in Rouse et al., Reference Rouse, Goffredi, Johnson and Vrijenhoek2011). For the present experiment the thresher shark jaws were placed in mesh bags (Figure 1A), which stopped the teeth being buried when the jaws disintegrated.
There was no evidence of Osedax boring through the outer tooth crown, which is made up of enameloid, a much harder substrate than dentin or bone, and with a much lower proportion of organic matrix (Enax et al., Reference Enax, Prymak, Raabe and Epple2012). Also, shark enameloid incorporates significant amounts (2.5% or more) of fluoride (LeGeros, Reference LeGeros1981; Miake et al., Reference Miake, Aoba, Moreno, Shimoda, Prostak and Suga1991) and this may resist the acid deployed by Osedax (Tresguerres et al., Reference Tresguerres, Katz and Rouse2013). These factors could preclude Osedax larvae from surviving long enough to bore through the enameloid crown to access the organic-rich dentin beneath. Kiel et al. (Reference Kiel, Kahl and Goedert2013) showed evidence of boreholes in the teeth of Oligocene whales, and these also appeared to be restricted to the root with little evidence of boring into enamel crown. Tessellated cartilage, with its collagen matrix (Maisey et al., Reference Maisey, Denton, Burrow and Pradel2021), is a possible food source for Osedax, however, there was no evidence of Osedax utilizing this calcified sheath that surrounded the hyaline cartilage of the jaw. Further study on the possible utility of the various forms of cartilage found in skeletons of sharks and rays is warranted.
Osedax was discovered living on a whale skeleton and two species were initially described (Rouse et al., Reference Rouse, Goffredi and Vrijenhoek2004). Subsequently Osedax species were shown to be able to utilize other mammal bones, initially with the deployment of cow bones (Jones et al., Reference Jones, Johnson, Rouse and Vrijenhoek2008). This was dismissed as not being ecologically relevant and that Osedax was most likely a whale fall specialist (Glover et al., Reference Glover, Kemp, Smith and Dahlgren2008). Further deployment experiments revealed that Osedax could colonize the bones of marine teleosts (Rouse et al., Reference Rouse, Goffredi, Johnson and Vrijenhoek2011), seals (Rouse et al., Reference Rouse, Wilson, Worsaae and Vrijenhoek2015), marine turtles birds (Rouse et al., Reference Rouse, Goffredi, Johnson and Vrijenhoek2018) and alligators (McClain et al., Reference McClain, Nunnally, Dixon, Rouse and Benfield2019), rendering the whale fall specialist argument untenable. Also Osedax appears to have evolved well before the appearance of whales (Vrijenhoek et al., Reference Vrijenhoek, Johnson and Rouse2009; Danise & Higgs, Reference Danise and Higgs2015) and so its original nutritional choice remains unknown. The two species of Osedax found to exploit dentin in this study, O. packardorum and O. talkovici, are phylogenetically quite separated in the context of the overall diversity of the clade, belonging to subclades IV and I, respectively (Rouse et al., Reference Rouse, Goffredi, Johnson and Vrijenhoek2018). The ability of disparate Osedax lineages to exploit bones as well as dentin suggests calcified collagenous tissues may be an ancestral preference, rather than bone as previously proposed. Rouse et al. (Reference Rouse, Goffredi, Johnson and Vrijenhoek2018) summarized the various bone substrates that had been specifically colonized by different Osedax up to that time. Osedax talkovici was notable for having been found on the widest range of bones deployed at 600–1000 m depth (cow, whale, elephant seal, teleost, turkey, turtle) and to this can now be added shark teeth. Osedax packardorum lives across a similar depth range as O. talkovici (600–1000 m depth) but had only been found on whale and cow bones until now.
The discovery that Osedax can exploit the dentin of teeth raises the obvious question of the evolutionary origin of the group and its original nutrition source. Modern sharks (crown group Elasmobranchii) have a fossil record extending back into the Devonian (Frey et al., Reference Frey, Coates, Ginter, Hairapetian, Rücklin, Jerjen and Klug2019), with bony fishes (Osteichthyes) even older. Thus, bones and teeth have been available as food sources in the sea for over 400 million years. Sharks notably show substantial turnover of teeth in their lifetimes (Botella et al., Reference Botella, Valenzuela-Ríos and Martínez-Pérez2009) with shed teeth falling to the seafloor and potentially being available for Osedax. Osedax is nested within Siboglinidae, which contains taxa such as Frenulata, Sclerolinum and Vestimentifera, taxa that also utilize symbiotic bacteria for their nutrition, though their energy source is hydrogen sulphide or methane from seeps and/or vents (Rouse et al., Reference Rouse, Pleijel and Tilic2022). Estimates of the origin of Siboglinidae based on molecular clocks have yet to be fully explored, though such estimates for the origin of crown group Osedax and Vestimentifera both fall in the late Cretaceous (Vrijenhoek et al., Reference Vrijenhoek, Johnson and Rouse2009; Vrijenhoek, Reference Vrijenhoek2013; Taboada et al., Reference Taboada, Riesgo, Bas, Arnedo, Cristobo, Rouse and Avila2015). The presence of fossils attributed to Osedax and Vestimentifera date to the mid-Cretaceous and Jurassic, respectively (Danise & Higgs, Reference Danise and Higgs2015; Georgieva et al., Reference Georgieva, Little, Watson, Sephton, Ball and Glover2019), suggest an earlier origin of Siboglinidae as a whole. Close examination for Osedax traces in fossilized marine bones and teeth from earlier in the Mesozoic and possibly back into the Paleozoic may well be warranted.
Data
All relevant data are within the manuscript or on GenBank.
Acknowledgements
Thanks to the captains and crew of the R/V ‘Western Flyer’ and the pilots of ROV ‘Doc Ricketts’ for technical support and skill. Thanks to Tor Mowatt-Larssen for assistance with obtaining the thresher shark heads and to Alex Clifford for onboard processing. Avery Hiley is thanked for obtaining most of the COI sequences. We thank the two anonymous reviewers for their useful comments on the initial submission.
Financial support
Cruise support funding via the Monterey Bay Aquarium Research Institute (The David and Lucile Packard Foundation).
Conflict of interest
None.
Ethical standards
Not applicable.