Introduction
The common mallard Anas platyrhynchos Linnaeus, 1758 is by far the most abundant waterfowl in central Europe. The mallard is native, widespread over Eurasia and well adapted to cultivated landscapes (Bauer, Reference Bauer2005). Therefore, it seems rather surprising that recent investigations on the parasite community hosted by this bird species are scarce. During a conference on the current state of helminth parasite databases in Europe in 2003, it was stated that ‘information on helminth faunas are scattered in a large number of papers, often published in hardly accessible journals and written in national languages’ (Sitko, Reference Simberloff, Moore, Clayton and Moore1997). Several important books, including checklists of digeneans (Sitko et al., Reference Sitko, Faltýnková and Scholz2006), nematodes (Sitko & Okulewicz, Reference Sitko and Okulewicz2010) and acanthocephala (Sitko, Reference Sitko2011) in birds in the Czech and Slovak Republics as well as additions to an existing checklist of tapeworms (Ryšavý & Sitko, Reference Reier, Sattmann, Schwaha, Harl, Konecny and Haring1992) were published. Those publications covered the major groups of helminth parasites occurring in some avian hosts. Although these checklists are highly valued contributions, there are no recent studies including not only systematic findings but also descriptions and analyses of the ecological aspects of the parasite communities of avian hosts. Such analyses have been described as useful tools in many aspects of ecology, including parasite infracommunity ecology (Simberloff & Moore, Reference Sheehan, David, Tonkyn, Yarrow and Johnson1997). Even on a small scale such analyses can be helpful, as shown, for example, by Sheehan et al. (Reference Shea, Kersten, Puccia, Stanton, Stiso, Helgeson and Back2016) who distinguished between migrating and stationary cormorant populations by analysing their helminth communities. The presence or the absence of a parasite in an ecosystem may indicate the presence or absence of its definitive host, even if it has not been observed. As importantly, perhaps, as they are often less-studied, the presence or absence of a parasite in an ecosystem indicates the presence of its intermediate host/s. On a larger scale, the assessment of environmental conditions and changes in ecosystems using parasites as bioindicators has been one of the emerging topics in the field of environmental parasitology (Lafferty, Reference Lafferty1997; Vidal-Martínez et al., Reference Vidal-Martínez, Pech, Sures, Purucker and Poulin2010; Nachev & Sures, Reference Nachev and Sures2016).
The acanthocephalan genera Filicollis Lühe, 1911 and Polymorphus Lühe, 1911 (Polymorphidae) are found in a wide range of aquatic or semi-aquatic birds (Petrochenko, Reference Pav and Zajicek1958). Species reported from European localities include Filicollis anatis (Schrank, 1788), and ten species of the genus Polymorphus, including six from the common mallard – namely, Polymorphus contortus (Bremser, 1821), Polymorphus corynoides (Skrjabin, 1913), Polymorphus diploinflatus Lundström, 1942, Polymorphus marilis Van Cleave 1939, Polymorphus meyeri Lundström, 1942 and Polymorphus minutus (Goeze, 1782) (see Petrochenko, Reference Pav and Zajicek1958; Golvan, Reference Golvan1994; Amin, Reference Amin2013). There has been, however, much confusion surrounding the identity of P. minutus (Bezubik, Reference Bezubik1957). Amin (Reference Amin1992) accepted the argument of Bezubik (Reference Bezubik1957) that Polymorphus magnus Skrjabin, 1913 is a junior synonym of P. minutus sensu Goeze, 1782 and that P. minutus sensu Petrochenko, 1958 is, in fact, P. contortus. Unfortunately, some authors are continuing to site the junior synonym P. magnus and P. minutus sensu Petrochenko, 1958 as valid species (e.g. Brgelz et al., Reference Brgelz, Ippen and Schröder1980; Birova et al., Reference Birova, Spakulova and Macko1990; Dimitrova et al., Reference Dimitrova, Murai and Genov1990; Dimitrova & Genov, Reference Dimitrova and Genov1992; Canaris & Kinsella, Reference Canaris and Kinsella2000; Goncharova et al., Reference Goncharova, Jjukshina, Movessian, Valova and Khbutiya2002; Bhattacharya, Reference Bhattacharya2003; Nikishin, 2004; Kavetska et al., Reference Kavetska, Rzad, Kornyushin, Korol, Sitko and Szałańska2008). Moreover P. minutus has been described previously as exhibiting a wide range of variability within and between different host species across a broad geographical range (Van Cleave & Starrett, Reference Van Cleave and Starrett1940). Three different genetic lineages, each with a different intermediate host, were recently discovered, which probably represent cryptic species (Zittel et al., Reference Zittel, Grabner, Wlecklik, Sures, Leese, Taraschewski and Weigand2018; Grabner et al., Reference Grabner, Doliwa, Bulantova, Horák and Sures2020).
Trematodes, in particular digenea, are by far the largest group of internal metazoan parasites, with 18,000 nominal species (Cribb et al., Reference Cribb, Bray, Littlewood, Pichelin, Herniou, Littlewood and Bray2001) and an important parasite group of birds. Although this huge variety has been documented, new species and lineages are discovered whenever an ecosystem is under investigation and the knowledge, particularly of this group, has been described as patchy at best (Sures et al., Reference Sures, Nachev, Pahl, Grabner and Selbach2017). Due to their complex life cycles, including a vertebrate (e.g. a bird) as definitive host and at least one intermediate host, their survival depends on manifold factors including the presence of the suitable hosts and the availability of appropriate environmental conditions for transmission of the life stages from one host to another. The diversity of trematodes found in an ecosystem is considered a suitable indicator for ecosystem health by, amongst others, Huspeni & Lafferty (Reference Huspeni and Lafferty2004) and Shea et al. (Reference Rzad, Sitko, Dzika, Zalewski, Smietana and Busse2012). High diversity, in this instance, refers mostly to the larval stages of digeneans found in snails and the diversity of larval stages in mallards is poorly known. Studies on A. platyrhynchos report varying numbers of trematode species: Chu et al. (Reference Chu, Cho, Chung, Won and Yoon1973) reported five species of intestinal trematodes from wild ducks in Korea; Fedynich & Pence (Reference Fedynich and Pence1994) reported six intestinal species from mallards in Texas; Kavetska et al. (Reference Kavetska, Rzad, Kornyushin, Korol, Sitko and Szałańska2008) identified 17 species in Polish hosts; and 43 species of trematodes from the intestine and other locations have been recorded in different studies of the mallard from the Czech and Slovak Republics by Sitko et al.(Reference Sitko, Faltýnková and Scholz2006). Echinostoma revolutum (Fröhlich, 1802) was the only species present in all studies, being described as the most widely distributed of the echinostome species. Echinostoma revolutum is pathogenic to humans when the intermediate hosts – raw snails and frogs – are consumed (Chai, Reference Chai, Toledo and Fried2009).
As already stated by Janovy (Reference Janovy, Clayton and Moore1997), there is a lack of research on avian cestodes compared to other groups of parasites. This has been highlighted in more recent studies emphasizing that the diversity of cestode species is highly dependent on the number of host species examined. This has been best documented in game waterfowl. Marinova et al. (Reference Marinova, Georgiev and Vasileva2013) recorded 26 cestode species in A. platyrhynchos from Bulgaria. A similarly high diversity was described by Kavetska et al. (Reference Kavetska, Rzad, Kornyushin, Korol, Sitko and Szałańska2008), who identified 23 different species, 22 belonging to the family Hymenolepididae and one from the family Dilepididae, from mallards in Poland. Fewer species (13) were recorded by Fedynich & Pence (Reference Fedynich and Pence1994), although it is worth noting that these authors did not cite how many different species they subsumed as Diorchis spp. Thirteen species were described by authors from former Czechoslovakia (Pav & Zajicek, Reference Pav and Zajicek1963). It is noteworthy that, even in neighbouring countries such as Poland and former Czechoslovakia that display a high diversity of species, only four are described from both – namely, Aploparaxis furcigera (Rudolphi, 1819), Dicranotaenia coronula (Djuardin, 1845), Fimbriaria fasciolaris (Pallas, 1781) and Sobolevicanthus gracilis (Zeder, 1803). This emphasizes the need for molecular identification and clarification.
For this work we had the opportunity to get 60 freshly shot mallards directly from the hunters, from hunting grounds in northern and eastern Austria. The first aim of this project was to contribute to the knowledge of the intestinal parasite community of A. platyrhynchos by presenting the first report from Austria. The second was to confirm the identity of any specimens of the genus Polymorphus recovered in this survey. Analyses of prevalence and intensity of infection in this helminth community were carried out to give insight into species dominance, richness and evenness, thus allowing comparisons with helminth community structures from central European and other avian host–parasite systems. Further, some insights might be gained into the ecological status of the aquatic ecosystems of north-eastern Austria.
Materials and methods
Study site
Sampling for this study took place in the north-eastern part of Austria (fig. 1), which includes the capital Vienna (1.9 million inhabitants), with its industrial impact on the environment. The surrounding region is a typical man-made environment characterized by a population density of 84 inhabitants/km2 (Statistics Austria, 2014) dominated by agricultural activity and managed forests. The region lies within the catchment area of the Danube River and floodplains, smaller rivers and numerous lakes and ponds are present. Water quality is monitored in Austria regularly. The most recent available report from 2019, including data from 2016, states that 99% of the investigated water bodies were in ‘very good’ or ‘good’ physico-chemical conditions. Looking at the biological parameters, including macro-zoobenthos and phytobenthos, 44% of surface water bodies must be considered ‘mediocre’ or worse (BMNT, 2019). Hunting is executed within the whole region, except for congested areas. By far the most abundant waterfowl is the mallard A. platyrhynchos, which is native, widespread over Eurasia and well adapted to cultivated landscape. The numbers of wild fowl are estimated to be 10,000–20,000 breeding pairs in Austria (Bauer, Reference Bauer2005).

Fig. 1. Geographical locations of the sampling areas for Anas platyrhynchos from eastern Austria. Abbreviations: BE, Bernhardsthal; EB, Ebreichsdorf; GR, Groß-Schweinbarth; HA, Haag; LI, Litschau; TU, Tulln.
Animal sampling
In total, 60 specimens of A. platyrhynchos were received from six hunting grounds situated in the eastern parts of Austria (fig. 1). They were shot by hunters during autumn, using commercially available lead shot. Freshly killed animals were transported to the University of Veterinary Medicine in Vienna, where sex and weight were determined. Specimens were stored at 4°C until dissection, which was conducted as soon as possible, with a maximum time from delivery to the last dissected animal being three days. For parasite inspection, the digestive tract was removed, cut open and inspected under a dissecting microscope. Parasites were removed and stored in 4% formalin or 70% ethanol for identification and quantification. Nematodes and trematodes were identified with the help of the keys by McDonald (Reference McDonald1974) and McDonald (Reference McDonald1981), respectively. Cestodes were identified using the key by Khalil et al. (Reference Khalil, Jones and Bray1994). Acanthocephalans were identified with the help of the key by Smales (Reference Smales and Schmidt-Rhaesa2015) and relevant species descriptions. The parasitological terms used are those defined by Bush et al. (Reference Bush, Lafferty, Lotz and Shostak1997). Measures of the helminth community structure were the Shannon–Wiener index and evenness, Simpson's index and the Berger–Parker index as proposed by Magurran (Reference Magurran1988). Specimens of P. minutus were deposited in the South Australian Museum with registration numbers AHC 47,980 and 47,981. All other specimens were deposited in the Museum of Natural History, Vienna.
Molecular genetic methods
Acanthocephalans were all placed in 70% ethanol prior to examination as temporary wet mounts cleared in beech wood creosote for identification. As the morphological identification of P. minutus was ambiguous, specimens of adult P. minutus from waterfowl from the UK, registered in the Natural History Museum, London (BMNH 1923.12.19.92–95, 1927.10.14.13–32, 1927.10.17.3–4, 1927.12.15.121–122, 1935.4.16.152–157, 1946.5.14.107–120, 1956.8.16.11–25,1969.347–355, 1973.156–160); from the Rhine, Germany, on loan from the Karlsruhe Institute of Technology, as well as cystacanths from Gammarus pulex (SAM AHC47567); from Germany from Gammarus roeselii (SAM AHC47568–71); from the Czech Republic (A54/1), from G. pulex from Ireland, and from Echinogammarus berilloni from France on loan from the Karlsruhe Institute of Technology, were examined for comparative purposes. For further support of the morphological identification, we conducted a molecular genetic characterization by DNA barcoding of species of genera belonging to the phylum Acanthocephala. Forty-two acanthocephalans were used for the molecular genetic analysis. DNA extraction was conducted in a clean room using the QIAmp DNeasy Blood and Tissue Kit (QIAGEN, Hilden, Germany) following the protocol of the manufacturer. A partial fragment of approximately 650 base pairs (bp) of the mitochondrial cytochrome c oxidase subunit 1 gene (COI) was used for DNA barcoding, amplified with the following primer pairs: H14AcanCOIFw1 (5-TTCTACAAATCATAARGATATYGG) as forward primer and H14AcanCOIRv2 (5-AAAATATAMACTTCAGGATGACCAAA) as reverse primer (Reier et al., Reference Reier, Sattmann, Schwaha, Harl, Konecny and Haring2019) for the species F. anatis and Poly-1+ (5-GTTWATATATGTWTTGGTTAGATTATG) as forward and Poly-2- (5-AATAAATGCTGATAYAAWARAGG) as reverse primers for the genus Polymorphus. Polymerase chain reactions (PCRs) were conducted in a final volume of 25 μl and contained 18.9 μl distilled water, 2.5 μl 10× PCR buffer, 1.5 mm MgCl2, 0.2 mm of each dNTP, 0.5 μM of each primer, 0.5 units TopTaq Polymerase and 1 μl template DNA. The amplification conditions started with an initial denaturation at 94°C for 3 min followed by 35 cycles of denaturation at 94°C for 30 s, primer annealing at 48°C for 60 s and extension at 72°C for 60 s. The temperature was held at 72°C for 7 min to complete elongation. The PCR products were sequenced (both directions) by Microsynth (Balgach, Switzerland) using the PCR primers. Sequences were manually aligned and checked using the program BioEdit (Hall, Reference Hall1999). Sequences determined in the present study were deposited in GenBank under the accession numbers MT184812–MT184814 (Polymorphus cf. minutus) and MT260853–MT260856 (F. anatis). A nucleotide BLAST® search was performed in National Center for Biotechnology Information (NCBI) GenBank to match the generated sequences to previously published sequences in GenBank. Then, five sequences of polymorphids available in NCBI GenBank were included into the final alignment: P. minutus (EF467865), Polymorphus obtusus (JX442195), Polymorphus sp. (DQ089721), Polymorphus brevis (KC549453 and KC549480), Profilicollis bullocki (JX442197), Profilicollis antarcticus (KX702253) and Pseudocorynosoma anatarium (EU267821). Also included in the alignment were selected BOLD sequences, with a minimum length of 600 bp, from the project PACDE-XXX-18 (individual numbers referred as XXX are given: 002–004, 008, 010–022, 032, 035–39, 041–043, 046–048, 051–053, 056–058, 061, 062, 066, 067, 069–071, 074–076, 078–082, 084–090, 097, 099, 106, 107, 117, 118, 134, 146–165). There was no reference COI sequence for F. anatis available in GenBank. A published sequence of Pomphorhynchus laevis (EF051062) rooted the tree as the outgroup. Due to the different lengths of the sequences, we trimmed the final alignment to 571 bp. A neighbour-joining (NJ) tree was calculated with uncorrected p-distances and branch support was tested by bootstrapping with 1000 replications using MEGA 10.0.5 (Kumar et al., Reference Kumar, Stecher, Li, Knyaz and Tamura2018). FigTree 1.4.3 (http://tree.bio.ed.ac.uk/software/figtree/) was used for graphical processing of the tree. Intraspecific genetic distances were calculated using MEGA 10.0.5 (Kumar et al., Reference Kumar, Stecher, Li, Knyaz and Tamura2018). We calculated a median-joining (MJ) haplotype network (Bandelt et al., Reference Bandelt, Forster and Rohl1999) with the software PopART 1.7 (Leigh & Bryant, Reference Leigh and Bryant2015) for P. cf. minutus using a 570 bp COI alignment of 22 sequences (three of this study, EF467865, and PACDE-XXX-18: 017, 018, 032, 035–038, 047, 048, 057, 058, 097, 099, 106, 107, 117, 118, 134). Calculations of haplotype diversity (Hd) and nucleotide diversity (π) of the COI dataset were conducted in DnaSP v5 (Librado & Rozas, Reference Librado and Rozas2009). InkScape 0.92 was used for the final layout of the tree and the network (www.inkscape.org).
Results
Helminth community
The 60 hosts harboured a total of 504 intestinal worms. The majority (39 hosts) carried zero to five worms, only six were heavily parasitized with 21–116 worms; the dispersion pattern is given in fig. 2. All species identified during this survey are listed in table 1, together with the statistical evaluation of the parasite community. A total of nine identified species, two acanthocephalans, four trematodes, two cestodes and a nematode were recovered, as well as a nematode that could not be identified further. Overall, the dominant parasite species was the cestode Diorchis sp. Clerc, 1903 (P = 31.7%, mean intensity (MI) = 8.7) followed by the acanthocephalan P. minutus (P = 30.0%, MI = 6.3) and the trematode Notocotylus attenuatus (Rudolphi, 1809) Kossack, 1911 (P = 23.3, MI = 6.9). Mixed infections occurred as well with two or more species from one or more of the systematic taxa. The diversity characteristics of the helminth community are given in table 2.
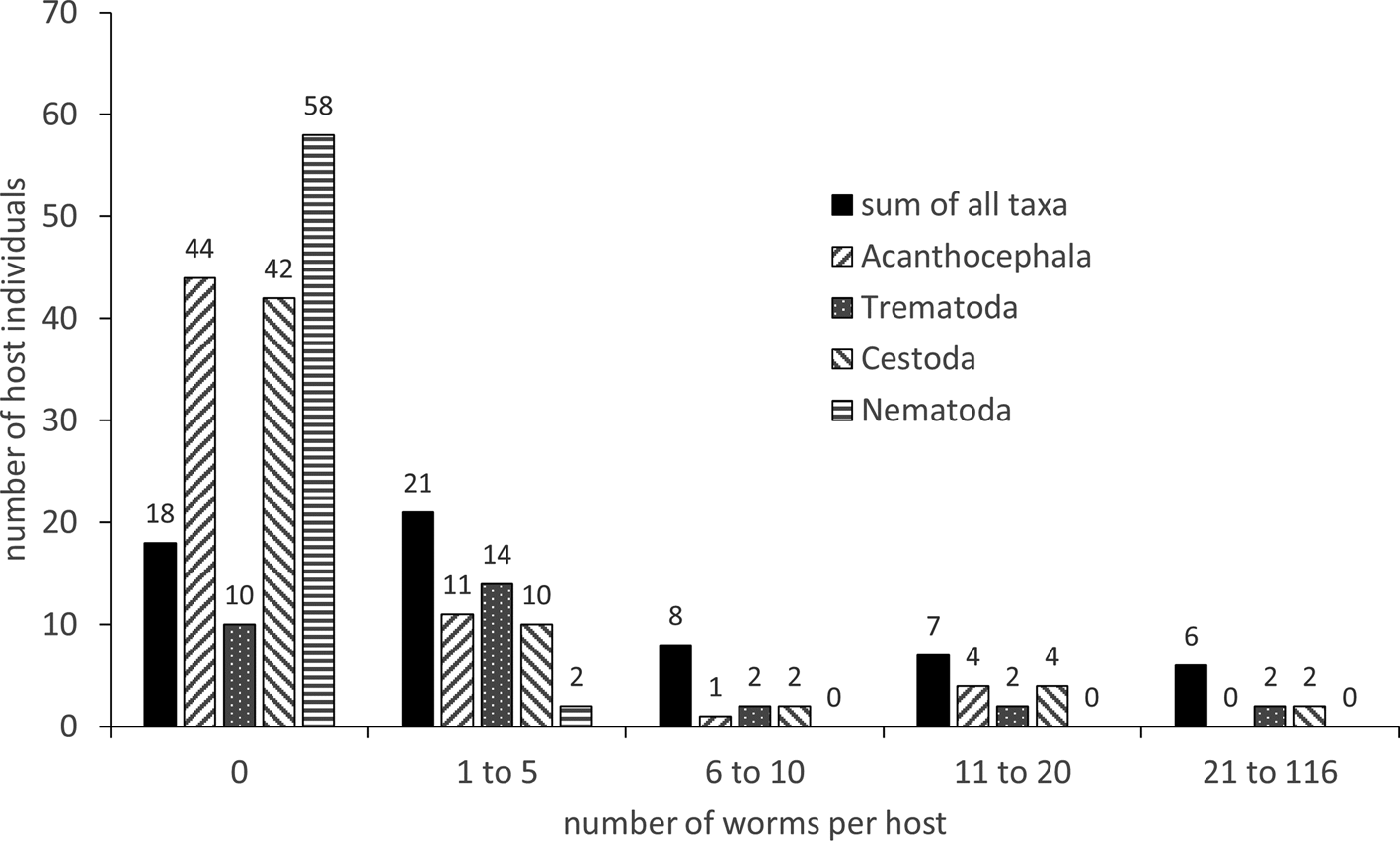
Fig. 2. Infection parameters of helminths in Anas platyrhynchos.
Table 1. Species list of helminth parasites recovered from Austrian mallards Anas platyrhynchos (n = 60).

Table 2. Diversity characteristics of the helminth community of the mallard from eastern Austria.
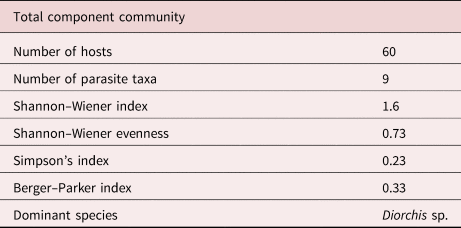
Genetic analysis of Acanthocephala
Only seven out of 42 specimens included in the genetic analysis gave positive PCR products. This could have been due to suboptimal fixation methods for molecular genetic analysis (e.g. use of formalin), which could have inhibited PCR (e.g. Kruckenhauser & Haring, Reference Kruckenhauser and Haring2008; Zimmermann et al., Reference Zimmermann, Hajibabaei, Blackburn, Hanken, Cantin, Posfai and Evans2008; Jaksch et al., Reference Jaksch, Eschner, Rintelen and Haring2016).
The calculated NJ tree revealed high bootstrap support for most of the nodes (fig. 3a). The specimens of this study occurred within two distinct clades: The basal split in the tree separated sequences of the species F. anatis from the remaining clades belonging to the family Polymorphidae (fig. 3a). The sequences of F. anatis showed a mean sequence divergence of 0.1%.
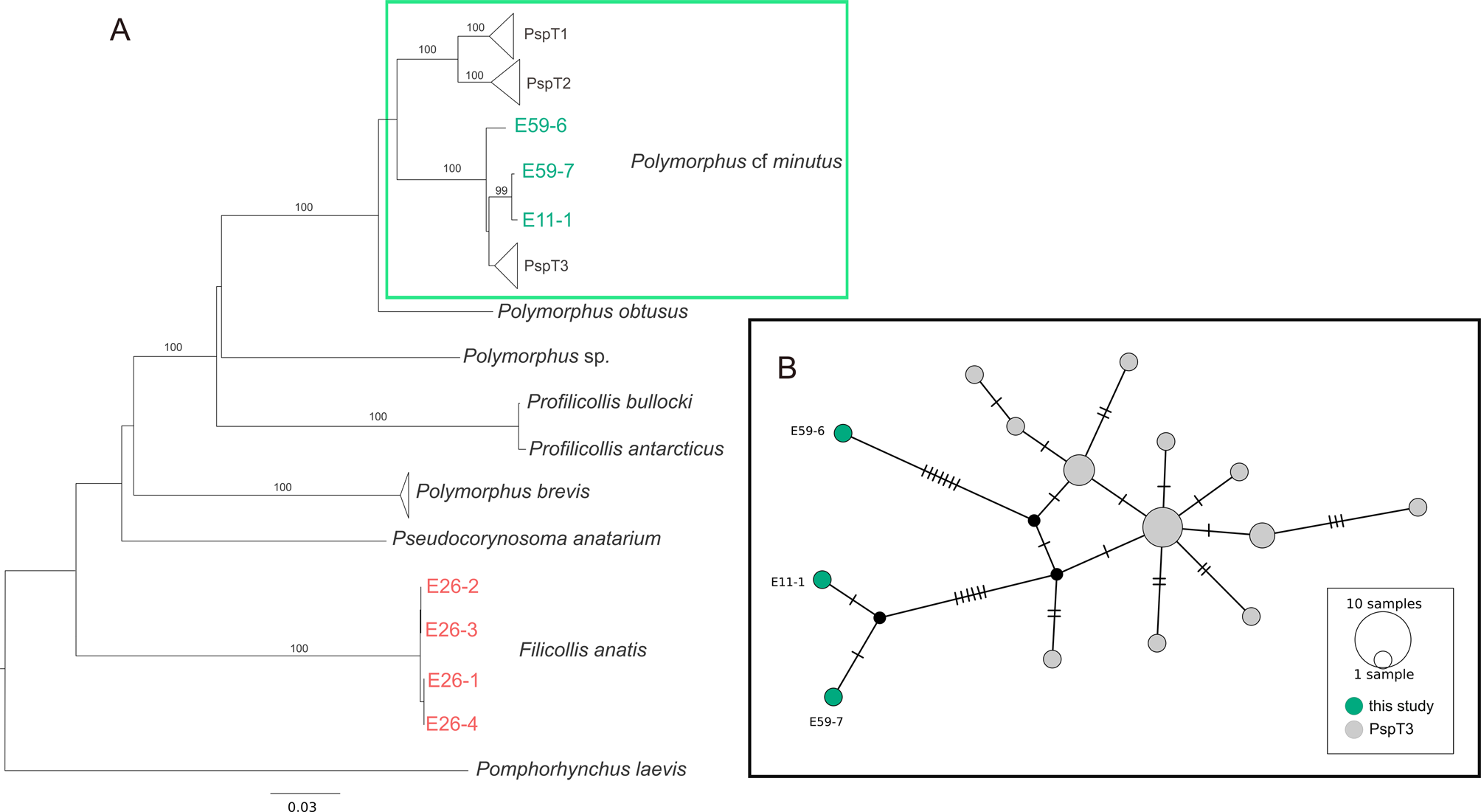
Fig. 3. Phylogenetic relationships between species of the family Polymorphidae based on a 571 bp COI dataset. (A) NJ tree showing uncorrected p-distances between the genera Polymorphus, Profilicollis, Pseudocorynosoma and Filicollis. Bootstrap values (1000 replicates, in %, only values with high support (>80%) are shown) are shown next to the nodes. The dataset includes sequences generated in this study and sequences obtained from NCBI GenBank and BOLD. Clades comprising sequences processed in this study are coloured. (B) MJ haplotype network of Polymorphus cf. minutus subclade PspT3. Mutation steps are indicated with vertical lines. Black dots represent haplotypes missing in the study sampling.
The sequences of P. minutus investigated in the present study were found in a main clade comprising three subclades (PspT1, PspT2 and PspT3), showing the same topology as in Zittel et al. (Reference Zittel, Grabner, Wlecklik, Sures, Leese, Taraschewski and Weigand2018). The sequences of this study clustered in subclade PspT3, together with sequences processed by Zittel et al. (Reference Zittel, Grabner, Wlecklik, Sures, Leese, Taraschewski and Weigand2018) and one sequence obtained from GenBank.
We calculated a mean genetic distance of 1.6% (range 0.4–1.9%) within the subclade PspT3, and one of 9.7% between PspT3 and the other two subclades PspT1 and PspT2. The mean genetic distance between the subclade PspT3 and the closest related single sequence of P. obtusus was 11.5%, while the distances to all other clades and single sequences were between 21 and 32%. The MJ network of the subclade PspT3 showed a high intraspecific variation with a high Hd of 0.94 and a considerably low π of 0.008. The network consisted of 15 haplotypes, whereof three haplotypes were found among the specimens of this study (fig. 3b). One specimen (E59-6) was separated by seven mutation steps from the main haplo-group, including sequences processed by Zittel et al. (Reference Zittel, Grabner, Wlecklik, Sures, Leese, Taraschewski and Weigand2018), mostly from Germany. Two samples (E59-7 and E11-1) were closely related to each other and separated by six mutation steps from this main haplo-group (fig. 3b).
Discussion
Helminth community
The observed aggregated distribution is in accordance with most publications on helminth parasites of field populations of birds (e.g. Goater & Holmes, Reference Goater, Holmes, Clayton and Moore1997 and references therein). Comparing the diversity characteristics with the literature is not easy, as publications vary in the evaluation of community structures and infection rates to a high degree. Although data for comparison are scarce, we can say that diversity indices (Shannon–Wiener, Simpson) do not point to a high diversity, but the distribution of parasite species (evenness) has a value of 0.73, indicating a rather evenly distributed species diversity across the sampled specimens (the value of ‘1’ would mean a completely even distribution). Also, the Berger Parker index is rather low, indicating a not too high proportional importance of the most abundant species. Very recently, Rzad et al. (Reference Ryšavý and Sitko2020) compared the community structure of trematodes in mallards from Poland and the Czech Republic and stated that a higher diversity was observed in Poland due to a smaller anthropogenic influence compared to that in the Czech Republic. That the Austrian region under investigation might be considered even more influenced and altered by humans, may be the reason for the depauperate helminth community observed in this study as compared to others. Of course, these alterations may not be influencing the occurrence of ducks directly, as they are well adapted to human presence (Bauer, Reference Bauer2005). As stated by the Austrian Government, 44% of surface waters are not in good conditions regarding their macro-zoobenthic community (BMNT, 2019). The massive restructuring and diverse use of all waterbodies in the region might have major influences on the occurrence and abundance of suitable intermediate hosts for avian parasites and influencing their community structure in this indirect way. Amongst those uses is, for example, the damming of many streams to produce electricity with over 1300 so-called ‘small hydro-electric power stations’ in the region (kleinwasserkraft, 2021) or the use of ponds for fish farming, where anti-parasitic drugs might be used to protect crowded fish populations. These fish farms currently consist of a network of 121 farmers using 13 km2 of ponds in the region (Niederösterreichischer Teichwirteverband, 2021). An additional factor that reduces the biodiversity of intestinal helminths is chronic lead intoxication (Pruter et al., Reference Pruter, Franz and Auls2018). Lead levels in liver described by these authors are congruent with the levels in liver of mallards from Austria given by Plessl et al. (Reference Plessl, Jandrisits, Krachler, Keppler and Jirsa2017), who reported at least 3.9% of the mallards showing acute lead pollution.
Nematoda
Most striking is the occurrence of only two individual nematodes within the sample. This stands in contrast to most literature on the nematodes of mallards. Pav & Zajicek (Reference Pav and Zajicek1963), Birova & Macko (Reference Birova and Macko1984), Fedynich & Pence (Reference Fedynich and Pence1994), Kavetska et al. (Reference Kavetska, Rzad, Kornyushin, Korol, Sitko and Szałańska2008), Yoshino et al. (Reference Yoshino, Uemura, Endoh, Kaneko, Osa and Asakawa2009) and Syrota et al. (Reference Syrota, Greben, Poluda, Maleha, Lisitsyna and Kornyushin2018) identified between four and nine species. Only De Jong (Reference De Jong1976) reported negative results from mallards from the Netherlands. We identified Porrocaecum crassum (Deslongchamps, 1824), which has been recorded as occurring in low prevalences and low intensities in other studies (2% and 1–2 in Canada; 5% and 1–4 in eastern Slovakia, respectively) (Birova & Macko, Reference Birova and Macko1984; Fedynich & Pence, Reference Fedynich and Pence1994). Birova et al. (Reference Birova, Spakulova and Macko1992) also described a general seasonal fluctuation in nematode numbers with a rapid reduction by October and lowest numbers in November and December. In particular, for P. crassum they observed low intensities (1–4) over the whole year, with fluctuations in prevalence between the lowest, 6.1% in October and the highest 39.5% in February (Birova et al., Reference Birova, Spakulova and Macko1990). Our samples were obtained in October and November, so the time of sampling might contribute to the paucity of nematode findings. A pronounced seasonality has also been described for Tetrameres fissispina (Diesing, 1861), which has been reported as the most prevalent nematode in mallards in Eastern Slovakia (Birova et al., Reference Birova, Spakulova and Macko1992) but was absent in our study. Interestingly enough, this species has been considered to be an incidental finding by other authors (Fenton et al., Reference Fenton, MacManamon, Howerth, Terio, McAloose and Leger2018), which might point to a non-regular appearance in general, most possible depending on the occurrence of suitable intermediate hosts, which are crustaceans. Amidostomum acutum (Lundahl, 1848), another well-known nematode of mallards (Birova & Macko, Reference Birova and Macko1984; Fedynich & Pence, Reference Fedynich and Pence1994), was absent as well. The parasite has a direct life cycle with eggs developing in the environment (Anderson, Reference Anderson1992), its presence does not depend on intermediate hosts and its absence in this study must remain unexplained and calls for further investigation.
Cestoda
The cestode Diorchis sp. was the dominant parasite species in our study, occurring in 31.7% of the mallards with intensities between 1 and 26 worms per host. We only recorded one other species of cestode: Fimbriarioides intermedia (Fuhrmann, 1913) was found in a single bird host. Our findings stand in complete contrast to most studies published, in which cestodes showed much higher diversities and very often higher infection intensities. Even in the aforementioned study from the Netherlands, in which no nematodes were discovered, a total of nine cestode species were identified (De Jong, Reference De Jong1976). Because cestodes have a life cycle which involves a crustacean (copepod, ostracod or amphipod) as intermediate host (Hiepe et al., Reference Hiepe, Buchwalder and Nickel1985), it could be argued that since most crustaceans are much more sensitive to pollution than, for example, molluscs, the occurrence of cestodes might be reduced in Austrian waterbodies. Consequently, fewer cestode species might be found infecting Austrian mallards. But this would need further investigation.
Trematoda
The diversity in trematode species found in other studies is highly variable. We identified four species of trematodes, which is comparable to five intestinal species found by Pav & Zajicek (Reference Nikishin1960) in a relatively small area in the former Czechoslovakia. Fedynich & Pence (Reference Fedynich and Pence1994) reported five species from Texas and Chu et al. (Reference Chu, Cho, Chung, Won and Yoon1973) reported six species in wild ducks from Korea. A much higher species diversity of 12 from a larger study area including 13 regions in Bohemia and Moravia was reported by Pav & Zajicek (Reference Pav and Zajicek1963); this number was exceeded by Kavetska et al. (Reference Kavetska, Rzad, Kornyushin, Korol, Sitko and Szałańska2008), who identified 16 trematode species from the north-western part of Poland. Most remarkably, only one species, E. revolutum (Froelich, 1802) Looss, 1899 seems to be globally distributed and occurs in all the aforementioned reports as well as from Bangladesh (Yousuf et al., Reference Yousuf, Das, Anisuzzaman and Banowary2009) and Mexico (Farias & Canaris, Reference Farias and Canaris1986). Echinostoma revolutum uses lymnaeid snails as the first intermediate host and metacercariae develop in the second intermediate host, another snail, bivalve or amphibian. Definitive hosts include mammals and birds, which become infected by ingesting infected snails or amphibians (Hiepe et al., Reference Hiepe, Buchwalder and Nickel1985). Displaying so little host specificity indicates that the parasite may not have highly specific requirements to complete its life cycle and can, therefore, become prevalent in aquatic systems worldwide. On the one hand, however, species differentiation within the family Lymnaeidae is still under investigation (Vinarski, Reference Vinarski2013), and on the other hand, the systematic status of all worms identified as E. revolutum is also under discussion. Echinostoma revolutum could well represent a complex of closely related taxa, which are morphologically indistinguishable (Sitko et al., Reference Sitko, Faltýnková and Scholz2006). In more recent publications authors have, therefore, used the term ‘E. revolutum group’ for these worms (Syrota et al., Reference Syrota, Greben, Poluda, Maleha, Lisitsyna and Kornyushin2018). Further molecular investigations might identify a higher number of echinostome species, presently subsumed under E. revolutum. The prevalence of E. revolutum in our study was 6.7% and quite low compared to the 15% found by Fedynich & Pence (Reference Fedynich and Pence1994) or the 53% by Pav & Zajicek (Reference Nikishin1960). Intensity of infection of 1–19 was comparable with the two studies mentioned. The most prevalent trematode in our study was N. attenuatus, which is also distributed worldwide and was found in all the aforementioned studies except in the one from Texas (Fedynich & Pence, Reference Fedynich and Pence1994). Notocotylus attenuatus has an abbreviated life cycle, with no second intermediate host involved, metacercariae develop from cercariae that emerge when their aquatic lymnaeid intermediate hosts attach to solid surfaces, such as mollusc shells or water plants (Harper, Reference Harper1929). Host specificity, neither for the intermediate nor the definitive host seems to be well developed and, therefore, the widespread occurrence of the parasites is not surprising. Omnivorous bird species, such as the mallard, can become infected easily by ingesting infested food items such as molluscs, crustaceans or water plants. Prevalence of infection (23.3%) in our studies is much lower compared to that of other studies (40% by Pav & Zajicek (Reference Nikishin1960) and 60% by Birova & Macko (Reference Birova and Macko1984)). Apatemon gracilis uses fish as its second intermediate host and, therefore, our finding of A. gracilis in mallards is worth mentioning. Although the infection was of low prevalence (3.3%) and exhibited low intensities (3–8), this finding proves that mallards are piscivorous. Compared to other studies, the numbers in our study are low. Infection occurred with high prevalence (42%) and MI of 17.5 in the study from Texas (Fedynich & Pence, Reference Fedynich and Pence1994), and P = 43% and MI = 10.3 in the study by Pav & Zajicek (Reference Nikishin1960). Birova & Macko (Reference Birova and Macko1984) observed that the parasite vanished after the restoration of the wetlands resulted in a richer fauna of snails and crustaceans, which may have led to a shift in diet of mallards towards prey easier to catch than fish.
Acanthocephala
The acanthocephalan species recovered in this study accord well with other studies from this host. Specimens of the genus Polymorphus – namely, P. minutus and its junior synonym P. magnus (the status of P. magnus is discussed in the introduction) – and F. anatis are the two taxa recorded from Europe (Pav & Zajicek, Reference Pav and Zajicek1963; Macko, Reference Macko1979; Birova & Macko, Reference Birova and Macko1984; Kavetska et al., Reference Kavetska, Rzad, Kornyushin, Korol, Sitko and Szałańska2008; Crompton & Harrison, Reference Crompton and Harrison2009; Syrota et al., Reference Syrota, Greben, Poluda, Maleha, Lisitsyna and Kornyushin2018). One other acanthocephalan genus, Corynosoma, was recorded in mallards from North America, the species being Corynosoma constrictum Van Cleave, 1918 (Van Cleave & Starrett, Reference Van Cleave and Starrett1940; Fedynich & Pence, Reference Fedynich and Pence1994). Prevalence in our study for P. minutus (30%) was relatively high compared with earlier studies from central Europe. Macko (Reference Macko1979) and Birova et al. (Reference Birova, Spakulova and Macko1990) found only 1.5% and 2.2% prevalence, respectively, of the host population infected in Slovakia. By contrast, Crompton & Harrison (Reference Crompton and Harrison2009) found 50% of the mallards infected in Kent, UK. The prevalence of F. anatis from our work (5%) compares well to other publications. Infection intensities for acanthocephalans are reported to be highly variable and also subject to seasonal fluctuations, similar to those found in nematodes. Highest intensities occur in summer, most probably due to the period of maximal reproduction of their gammarid intermediate hosts (Birova et al., Reference Birova, Spakulova and Macko1990; Crompton & Harrison, Reference Crompton and Harrison2009). Ecosystem health seems to be an important factor for the occurrence of acanthocephalans: Birova & Macko (Reference Birova and Macko1984) observed a significant rise in F. anatis infections (prevalence as well as intensity) in mallards from the East Slovakian lowlands after water system regulations had been implemented. They argued that these measures changed many seasonal water bodies into permanent ones, therefore allowing continuous development of intermediate hosts and making the mallards more exposed to possible infections.
Genetic results
Even though no reference sequences of F. anatis were available, the identity of F. anatis was unequivocal, due to its distinct morphological traits. The identity of P. minutus was more difficult to establish because of the taxonomic confusion discussed above. The specimens of this study clustered in a subclade (PspT3), already defined by Zittel et al. (Reference Zittel, Grabner, Wlecklik, Sures, Leese, Taraschewski and Weigand2018). These authors suggested that cystacanths of P. cf. minutus comprising different lineages or cryptic species used different intermediate hosts. In this study, we also detected considerable variation in some characters, particularly proboscis armature and neck length, in individuals from several of the Austrian mallards. Grabner et al. (Reference Grabner, Doliwa, Bulantova, Horák and Sures2020) found similar variations in the proboscis armature of the three genetically distinguished subclades (PspT1, PspT2 and PspT3). We also calculated a lower number of hook rows (12–14; table 3) in the genetically investigated specimens of Austria, while previous descriptions of P. minutus referred to 14–18, usually 16, hook rows (e.g. Lühe, Reference Lühe1911; Amin, Reference Amin1992). Due to the morphological variations measured in this study, it can be expected that more lineages of P. cf. minutus may occur in Austria. Unfortunately, it was not possible to elucidate whether these morphologically examined specimens belonged to one of the other two subclades (PspT1, PspT2), or represented a further genetic lineage, since these specimens did not yield PCR products in the COI amplification, perhaps due to bad preservation.
Table 3. Comparative measurements of males and cystacanths of Polymorphus cf. minutus from Europe, the UK and North America.
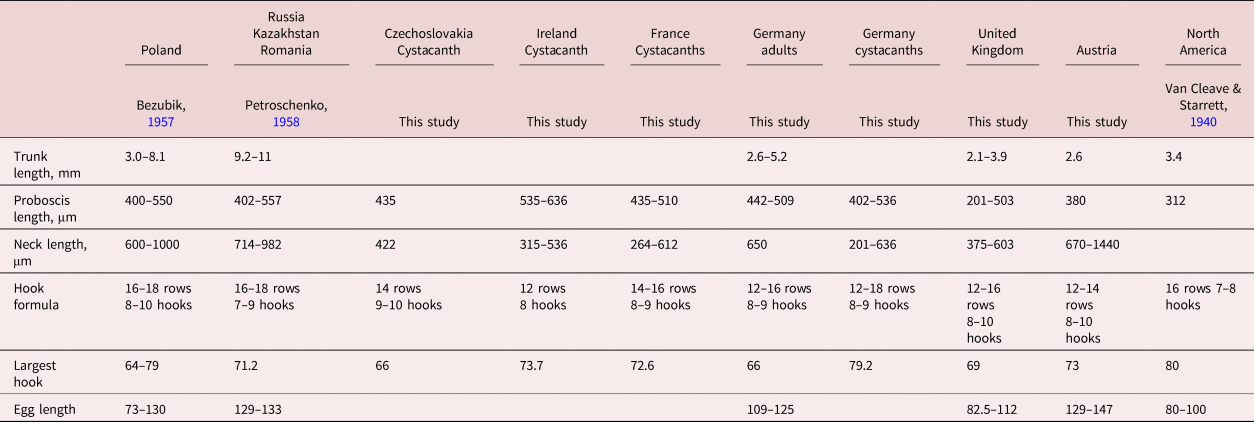
Polymorphus minutus has, however, been previously described as exhibiting a wide range of variability within and between different host species across a broad geographical range (Van Cleave & Starrett, Reference Van Cleave and Starrett1940; Zittel et al., Reference Zittel, Grabner, Wlecklik, Sures, Leese, Taraschewski and Weigand2018; Grabner et al., Reference Grabner, Doliwa, Bulantova, Horák and Sures2020) as can be seen in table 3. However, the genetic results further implicated the specimens from Austria as belonging to the subclade PspT3, which uses G. pulex and G. roeselii as intermediate hosts (Zittel et al., Reference Zittel, Grabner, Wlecklik, Sures, Leese, Taraschewski and Weigand2018; Grabner et al., Reference Grabner, Doliwa, Bulantova, Horák and Sures2020). According to Grabner et al. (Reference Grabner, Doliwa, Bulantova, Horák and Sures2020), it can be expected that the subclade was introduced by the invasive gammarid G. roeselii. Those authors suggested the invasion from south-east Europe to Germany, which would include a passage through Austria, where G. roeselii is a prevalent species in rivers (Poeckl et al., Reference Poeckl, Webb and Sutcliff2003). Further investigations regarding the species status of P. minutus are necessary, since there is a high probability that the three lineages known to date represent separate species.
Acknowledgements
We cordially thank Anja Joachim from the University of Veterinary Medicine, Vienna, for providing lab space for dissection and space in deep-freeze chambers. Michael J. Mühlegger is thanked for the dissection of the birds. Michaela Hejl and Anton Legin are thanked for the translation of Slovakian and Russian literature, respectively. We thank Elisabeth Haring for valuable discussion of genetic data. In addition, the cooperation with the confederations of hunters from Lower Austria and Upper Austria, mediated by Alois Gansterer, is highly acknowledged.
Financial support
Open access funding was provided by the University of Vienna.
Conflicts of interest
None.
Ethical standards
The authors assert that all procedures contributing to this work comply with the ethical standards of the relevant national and institutional guides on the care and use of laboratory animals.