Introduction
It is well established that fetal development can be influenced by various environmental factors particularly by maternal diet as the sole resource of the calorie and nutrients supply for the fetus. It is worth noting that fetal development can serve as a predictor of chronic diseases in later life. In recent decades, numerous studies have investigated the impact of the prenatal environment on both the fetus and the neonates, studying its effects on the prevalence of chronic diseases in the offspring. This phenomenon is well framed as the Developmental Origins of Health and Disease (DOHaD). Reference Armitage, Taylor and Poston1 During pregnancy and early childhood, developmental plasticity makes it possible for environmental factors (e.g., maternal and early childhood diet) to influence the development of tissues, organs, and regulatory systems (e.g., hypothalamus) via an adaptive mechanism. Reference Lucas, Baker, Desai and Hales2–Reference Gluckman and Hanson4 The predictive adaptive response (PAR) hypothesis has been developed to explain the interactive effects of the in utero and postnatal environment on phenotype of the offspring. According to the PAR, fetal adaptation to the maternal diet develops a prediction of the future environment accordingly. Therefore, similar maternal and weaning diet will result in a more positive outcome compared with an unmatched maternal and weaning diet Reference Lucas, Baker, Desai and Hales2 . For example, low-protein diets fed during 8 weeks prior to conception resulted in shorter but thicker guts of offspring, which may suggest that the intestines in the offspring are affected in the same manner as the intestines of the mice actually consuming the diets themselves supporting the PAR hypothesis for maternal preconception diets. This altered intestine (smaller and thicker dimensions) gives the offspring the ability to possess a higher amount of absorptive tissue per unit length of the gut, which could possibly lead to a higher absorption rate of fat, protein, carbohydrates, and calories leading to an obese phenotype. Reference Mortensen, Wang, Malte, Raubenheimer and Mayntz5
The impact of low-protein diet and high-protein diet (HPD) in maternal nutrition on the health outcomes of both mothers and their offspring has been studied considerably. Reference Zambrano, Bautista and Deas6–Reference Switkowski, Jacques, Must, Kleinman, Gillman and Oken15 Although both low- and high-protein maternal diets during pregnancy affects body weight (BW), blood pressure, metabolism, and food intake in the offspring, the focus of this study was solely on the effects of HPDs since the typical Western diet can be categorized as a HPD. The average protein consumption in 20–39 years old women in the United States is 73 grams/day Reference Hoy, Clemens and Moshfegh16 (NAHNES 2015-16) which is far beyond the recommended amount (46 g/day for women ages 19–70+ years). Reference Hoy, Clemens and Moshfegh16 Moreover, despite increased protein requirements during pregnancy (71 g/day), the average daily protein consumption in 20–40 years old pregnant women in the United States was higher compared with non-pregnant women (81.9 g and 71.4 g, respectively). Reference Bailey, Pac, Fulgoni, Reidy and Catalano17 A maternal HPD has been shown to elevate BW, Reference Zhang, Wang, Terroni, Cagampang, Hanson and Byrne9 blood pressure, and food utilization efficiency Reference Thone-Reineke, Kalk and Dorn10 while simultaneously reducing their energy expenditure Reference Daenzer, Ortmann, Klaus and Metges11 in the offspring of rats.
The role of a gestational HPD on fetal programming has been also studied by a few clinical studies. In humans, supplementation of a 40 g protein to the diet during pregnancy resulted in lower birth weight compared to those offspring born to mothers fed a low-protein supplement (6 g as 7.5% energy) beverage. Reference Rush, Kristal and Navarro18 In another study, maternal HPD during pregnancy and lactation resulted in higher BW at puberty, sustaining until the end of the study (week 22) only in female offspring of rats. Reference Thone-Reineke, Kalk and Dorn10 A high-protein gestational diet resulted in higher BW in the offspring which was associated with increased food efficiency Reference Zhang, Wang, Terroni, Cagampang, Hanson and Byrne9 and lower energy expenditure. Reference Daenzer, Ortmann, Klaus and Metges11 Nonetheless, these investigations predominantly focused on mothers with normal BW.
Furthermore, over two-thirds of women in the United States within the reproductive age range (20–39 years old) are either overweight or obese. Reference Hillemeier, Weisman, Chuang, Downs, McCall-Hosenfeld and Camacho19 This alarming trend has made gestational obesity a ubiquitous issue during pregnancy, emerging as a significant risk factor for the development of gestational diabetes mellitus (GDM). Reference Catalano and Shankar20–Reference Silverman, Rizzo and Green22 It is well established that gestational obesity negatively affects both mothers and their offspring. Reference Muhlhausler, Adam, Findlay, Duffield and Mcmillen3,Reference Catalano23 In mothers, it increases the risk of gestational hypertension, pre-eclampsia, GDM, preterm birth and prenatal death, and large-for-gestational-age babies. Reference Catalano and Shankar20 In offspring, gestational obesity predicts the development of obesity and cardiometabolic disease. Reference Kislal, Shook and Edlow24 Poor pregnancy outcomes in obese or mothers with GDM due to gestational obesity, can be attributed to the insulin resistance and inflammatory mediators from a hypoxic trophoblast in mothers which may alter fetal development. Reference Kislal, Shook and Edlow24 Maternal obesity or GDM increases the risk of obesity and/or glucose intolerance in offspring. Reference Catalano and Shankar20,Reference Silverman, Rizzo and Green22 There is a strong correlation between increased nutrient supply before birth and later obesity. Reference Glastras, Chen, Pollock and Saad26 Intrauterine exposure to maternal obesity is associated with an increased risk of metabolic syndrome and obesity in later life. Reference Glastras, Chen, Pollock and Saad26
Therefore, given the widespread adoption of HPDs in the contemporary Western lifestyle and the fact that over two-thirds of women aged 20–39 years in the United States are overweight or obese, this study sought to explore the effects of a HPD during pregnancy and lactation on food consumption, BW, composition, and glucose metabolism in obese Wistar rats and their offspring.
The primary objective of this study was to examine the DOHaD hypothesis and whether the HPD and normal-protein diet (NPD) fed during gestation and lactation affect mothers’ health and their offspring differently. Therefore, obese Wistar rat dams were fed either the AIN-93G HPD or NPD during pregnancy, and postpartum period. Additionally, according to the PAR hypothesis, there will be no negative impact of maternal diet on offspring weaned to the same maternal diet while offspring weaned to an unmatched diet with maternal diet may expect serious negative outcomes in later life. So, the secondary objective of this study was to examine the interactive effects of the protein content of the weaning diet and the dams’ diets on the offspring. To examine the PAR hypothesis, two groups of offspring from each maternal group were fed either a HPD or NPD.
Wistar rats have been used in numerous studies in this field because they share metabolic and physiological mechanisms that are parallel to those of humans and due to their relatively brief gestation and lactation periods, each lasting just three weeks, which makes them a perfect practical model for research in this field. In addition, a well-controlled environment in animal facilities will minimize the effect of outliers. In the current study, male offspring were utilized only to be comparable with previous studies conducted in our lab.
Materials and methods
Ethical statement
This study followed the National Institutes of Health guide for the care and use of laboratory animals (NIH Publications No. 8023, revised 1978). All experimental procedures involving Wistar rats were approved by the University of North Florida Institutional Animal Care and Use Committee (IACUC) (Protocol No: IACUC#16-001)
Experimental design
To calculate the sample size, a power analysis was performed based on data from a previous study Reference Muhlhausler, Adam, Findlay, Duffield and Mcmillen3 and based on statistical power (80%) and the two-sided significance level (0.05). Newly obese pregnant Wistar rats (on 3rd day of pregnancy) (n = 24) were randomly assigned to two groups (n = 12/ group) and received either a HPD or a NPD during pregnancy and lactation. At weaning, one male offspring from each dam within each maternal diet group was randomly allocated to either the HPD or NPD. This allocation was performed using a random number table ranging from 1 to 24 for each maternal group, totaling 48 for both maternal groups (1–12 for HPD and 13–24 for NPD). A research assistant selected two offspring from a pool of 10 pups from each mother’s cage, and based on the random number table, assigned the pups to the weaning diet groups randomly. The BW of both dams and their offspring was measured on weekly basis. In addition, BW of the pups was measured at birth. The litter size was reduced to ten pups per dam to ensure that all the mother’s offspring had equal access to her milk. Moreover, to examine the PAR hypothesis, one male offspring from each dam on each maternal diet group was randomly assigned to either the HPD or NPD (n = 12 per group) at weaning. BW was measured weekly for 15 weeks in the pos-weaning period. Fasting blood glucose (FBG) and blood glucose (BG) response to a glucose load (Oral Glucose Tolerance Test, OGTT) were measured at week 15 post-weaning (PW). The same parameters were measured in the dams at week 7 postpartum. Body composition was assessed by measuring fat pad mass at week 10 postpartum in the dams and at birth, at weaning, and week 17 PW for offspring (at the termination point). Similarly, plasma glucose and insulin were measured at birth, weaning, and at week 17 PW for the offspring and at week 10 postpartum in the dams. The variations in the total number of rats in each group, as described in the “Results” section, are due to various factors. These factors include the loss of animals resulting from unsuccessful attempts at procedures such as gavaging and intraperitoneal (IP) injections, which ultimately reduced the total number of rats.
Animals and diets
Female Wistar rats were subjected to an 8-week of high-fat, high-calorie diet before their initial mating, conducted at the breeder’s facility to make the rats obese before mating (Charles River, NC, USA). However, the obesity reduces the likelihood of pregnancy. Given the challenge of attaining the required number of timed-pregnant rats within our own animal facility, the breeder conducted mating procedures on a larger scale to obtain a cohort of 48 timed-pregnant rats for this study. First-time obese pregnant Wistar rats arrived on day 3 of gestation and housed individually in ventilated plastic cages with bedding. Environmental conditions were maintained at a temperature of 22 ± 1°C, under a 12-hour light–dark cycle (lights off from 0900 to 2100 h).
The diets (in pelleted form) offered in glass jars and water was provided through automatic hydration system. Rats had ad libitum access to both water and the diet. Standard diets (AIN-93G) were provided by Dyets Inc. (Bethlehem, Pa, USA). The composition of the diets (g/per kilogram diet) are presented in Table 1.
Table 1. Composition of the normal-protein, high-protein, and high-fat diets
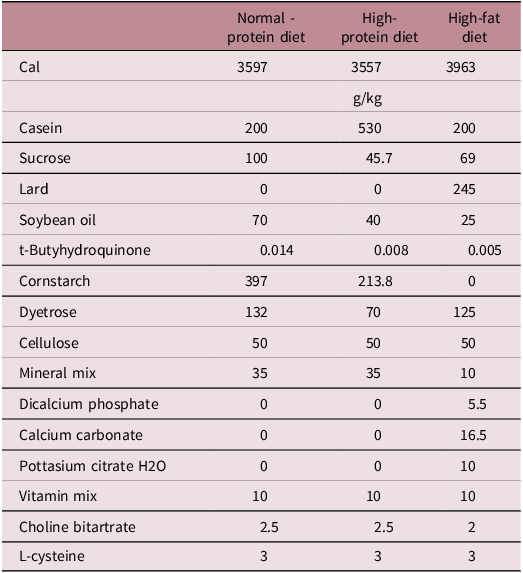
The well-being of animals was evaluated through physical assessments prior to, during, or after procedures. No significant adverse events were observed in any of the study groups throughout of the study.
Procedures
Food intake
Food intake was measured on weekly basis. To do so, the food containers at the beginning and at the end of each week was measured for 15 weeks. Spillage was also measured and deducted to calculate the real food intake.
Glucose tolerance test
After as overnight fasting for 12 h, blood samples were taken using the tail vein at fasting and at 15, 30, and 60 min after a glucose preload (0.375 g glucose per mL, 5 g glucose per kg BW). Reference Jahan-Mihan, Szeto, Luhovyy, Huot and Anderson27
Blood collection
At the end of the study, during the termination by guillotine, trunk blood (6 ml) was collected in chilled vacutainer tubes (BD, Franklin Lakes, NJ, USA) containing EDTA + Trasylol® (Bayer AG, Leverkusen, Germany) solution (10% blood volume, 5 × 108 IU L). Blood samples were centrifuged right after collection at 3,000 g and 4°C for 10 min. Plasma was separated from blood and directly stored at 70°C. Reference Jahan-Mihan, Szeto, Luhovyy, Huot and Anderson27
Blood glucose
To measure the BG concentration, blood sample was taken from the tail vein and was assayed by a hand-held commercial glucometer (Contour® Next Blood Glucose Meter, Bayer Healthcare LLC, Mishawaka, IN, USA) using test strips. Control solutions (levels 1 and 2) (Bayer, Bayer Healthcare LLC, Mishawaka, IN, USA) was also used to assess the accuracy and variance of the glucometer and test strips. Reference Jahan-Mihan, Szeto, Luhovyy, Huot and Anderson27
Hormone assays
An enzyme-linked immunosorbent assay (catalog no. 80- INSRT-E01, Alpco Diagnostics, Salem, NH, USA) was used to measure plasma insulin concentrations. The assay sensitivity was 0.124 ng mL1.
Body composition
To assess the body composition, fat mass and lean mass were measured right after termination at week 7 PW for dams and week 17 PW for pups. Fat mass was assessed through dissection of extracted abdominal, epididymal, and perirenal fat. Reference Jahan-Mihan, Szeto, Luhovyy, Huot and Anderson27
Statistical analyses
The primary and interactive effects of maternal and weaning diets on BW and glucose response were assessed through a two-way analysis of variance (ANOVA). Repeated measures were taken over time for BW, food intake, BG response, and FBG. The PROC MIXED procedure was utilized, with maternal diets, weaning diets, and time as the main parameters. If interactions showed statistical significance, a one-way ANOVA was followed by a post hoc Tukey’s test to assess the treatment effects. The effects of maternal diet on plasma measures were compared using an unpaired t-test. BG response was quantified as the total area under the curve (tAUC) of BG concentration over 1 hour following the glucose administration during the OGTT. The Homeostasis Model Assessment of Insulin Resistance (HOMA-IR) index was calculated as fasting glucose multiplied by fasting insulin divided by 22.5. Data are presented as means with standard errors. Statistical significance was defined as P < 0.05. All analyses were conducted using SAS (version 9.4; SAS Institute, Cary, NC, USA).
Results
Dams
BW of the dams was not affected by the protein content of the diet during pregnancy, and in postpartum period (Figure 1). Moreover, no effect of maternal diet on food intake during pregnancy and postpartum period was observed except for week 3 postpartum, when food intake was higher in mothers fed a HPD compared with those fed a NPD (P < 0.05) (Figure 2).
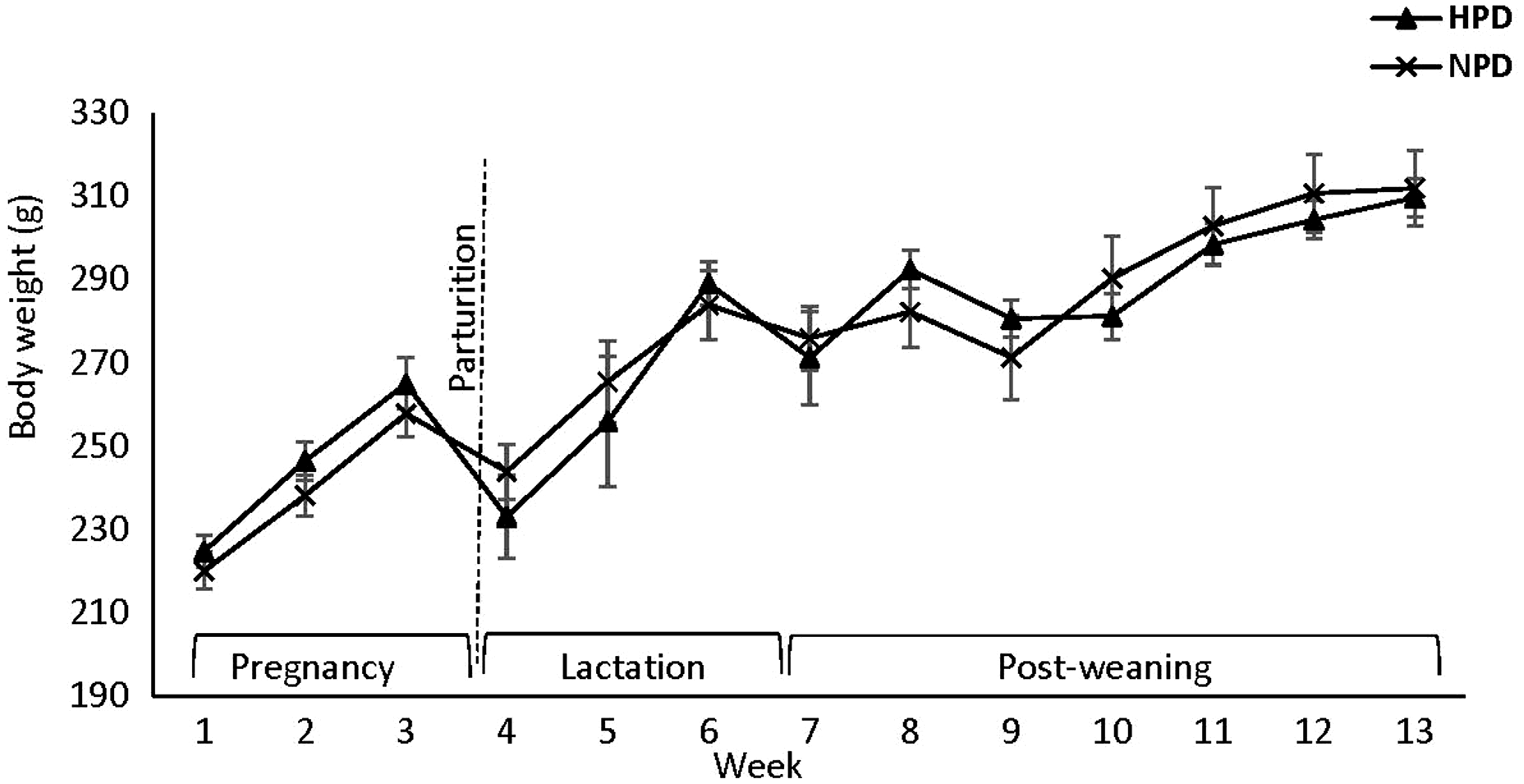
Figure 1. Effect of maternal HPD and NPD during gestation and postpartum period on dams’ body weight (BW). HPD, high-protein diet; NPD, normal-protein diet. Values are means, with their standard errors represented by vertical bars (n = 12). BW was analyzed by MIXED model followed by Tukey’s post hoc test with diet and time as main factors: diet (NS); time (P,0 · 0001).
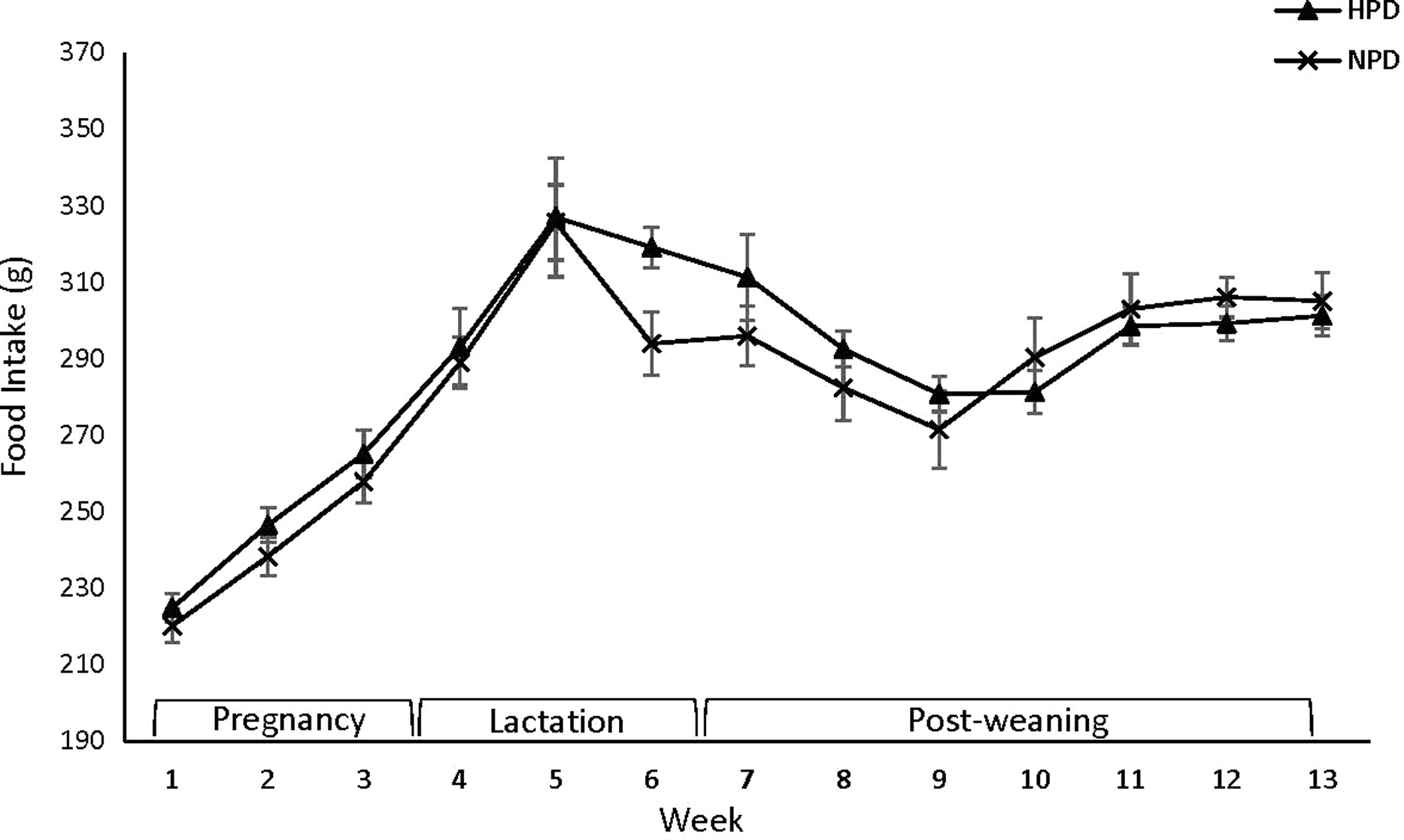
Figure 2. Effect of maternal HPD and NPD on dams’ food intake (FI). HPD, high-protein diet; NPD, normal-protein diet. Values are means, with their standard errors represented by vertical bars (n 12). Food intake was analyzed by MIXED model followed by Tukey’s post hoc test with diet and time as main factors: diet (NS); time (P,0 · 0001).
No significant difference in fat and fat/weight percentage at week 10 postpartum in dams was observed (Table 2).
Table 2. Body weight, fat, and fat/weight ratio of dams at week 10 and pups at birth, at weaning and at week 17 PW

HPD, high-protein diet; NPD, normal-protein diet; PW, post-weaning.
The total fat content is determined by pooling the dissected abdominal, perirenal, and epididymal fat.
Maternal diet did not influence mothers’ FBG, HOMA-IR index, or insulin/glucose ratio at week 10 postpartum. However, glucose response to glucose preload (OGTT) was influenced by maternal diet as demonstrated by higher tAUC observed in dams fed a NPD compared with those fed a HPD at week 7 postpartum (P < 0.03) (Table 3).
Table 3. Effect of high- and normal-protein diets of dams and offspring on fasting plasma measures in the offspring* (Mean values with their standard errors, n 8–12)
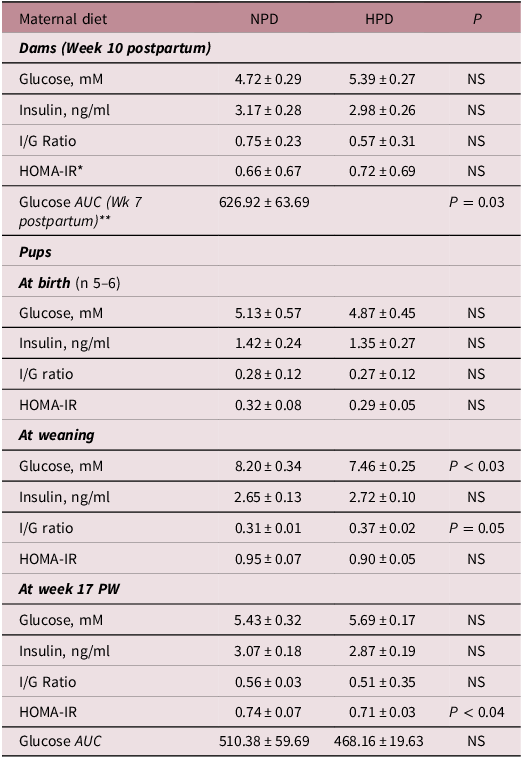
HPD, high-protein diet; NPD, normal-protein diet; HOMA-IR, homeostasis model assessment of insulin resistance; I/G ratio: insulin/glucose ratio; PW, post-weaning.
* HOMA-IR index was calculated as fasting glucose (mM) multiplied by fasting insulin (ng/ml) divided by 22 · 5.
** Glucose area under the curve (AUC): (g/mM*60 min).
Pups
Birth weight was not influenced by maternal diet (Table 2). Moreover, maternal diet did not influence indicators of glucose metabolism at birth (data are not shown).
At weaning, neither BW nor body fat was influenced by maternal diet. However, fat/weight ratio was relatively higher in offspring born to dams fed a NPD (P = 0.06). Neither maternal nor weaning diet’s effect on the BW of pups after weaning was observed (Figure 3). Similarly, neither maternal nor weaning diet influenced food intake at ay time in PW period (Figure 4).

Figure 3. Effect of maternal HPD and NPD on post-weaning body weight (BW) of male offspring. HPD, high-protein diet; NPD, normal-protein diet. Effect of protein source during gestation on post-weaning body weight (BW) of male offspring. HH, maternal and weaning high-protein diet diet; HN, maternal high and weaning normal-protein diet; NH, maternal normal and weaning high-protein diet; NN, maternal and weaning normal-protein diet. M, maternal diet; W, weaning diet; NS, not significant. Values are means, with their standard errors represented by vertical bars (n 12). BW was analyzed by MIXED model followed by Tukey’s post hoc test with gestational diet, weaning diet, and time as main factors: gestational diet (NS); weaning diet (NS); time (P,0 · 0001); gestational diet × time (P,0 · 05).

Figure 4. Effect of maternal HPD and NPD on post-weaning food intake of male offspring. HPD, high-protein diet; NPD, normal-protein diet. Effect of protein source during gestation on post-weaning body weight (BW) of male offspring. HH, maternal and weaning high-protein diet; HN, maternal high and weaning normal-protein diet; NH, maternal normal and weaning high-protein diet; NN, maternal and weaning normal-protein diet; M, maternal diet; W, weaning diet; NS, not significant. Values are means, with their standard errors represented by vertical bars (n 12). Food intake was analyzed by MIXED model followed by Tukey’s post hoc test with gestational diet, weaning diet, and time as main factors: gestational diet (NS); time (P,0 · 0001).
Glucose metabolism was not altered at birth (Table 3). However, at weaning, fasting plasma glucose was higher in offspring born to NPD dams (P < 0.03). Moreover, insulin/glucose ratio was influenced by maternal diet reaching to the significant point (P = 0.05). It was relatively higher in pups born to HPD dams (P = 0.05) (Table 3). No effect of either maternal or weaning diet on fasting plasma glucose, insulin, insulin/glucose ratio, and glucose response to glucose preload at week 17 PW was observed (Table 4). However, HOMA-IR index was higher in offspring born to NPD dams compared with those born to HPD dams at week 17 PW (P < 0.04) (Table 4). It was also affected by the weaning diet and was higher in offspring fed a NPD compared with those fed a HPD at week 17 PW (P < 0.05) (Table 4).
Table 4. Effect of high- and normal-protein maternal and weaning diets on fasting blood glucose, insulin, insulin/glucose ratio, and HOMA-IR index at week 17 (Mean values with their standard errors, n 8–12)

HPD, high-protein diet; NPD, normal-protein diet; HOMA-IR, homeostasis model assessment of insulin resistance; I/G ratio: insulin/glucose ratio; PW, post-weaning.
* HOMA-IR index was calculated as fasting glucose (mM) multiplied by fasting insulin (ng/ml) divided by 22 · 5.
Discussion
This study builds upon our previous investigations into the effect of the protein source in the maternal diet fed during gestation and lactation on the health of offspring in pregnant Wistar rats. Our earlier findings indicated that maternal casein and soya protein diet fed during gestation and lactation affected mothers and their offspring differently. Reference Jahan-Mihan28 Notably, the soya protein maternal diet negatively impacted the offspring’s BW, composition, blood pressure, and glucose metabolism compared to a casein-based maternal diet. Reference Jahan-Mihan28 In subsequent study on obese dams with a similar study design, gestational obesity overshadowed the impact of protein source in maternal diet on various parameters in both casein- and soy protein-fed dams and in their offspring Reference Jahan-Mihan28 .
Similarly, in the present study, we observed no significant effect of a maternal HPD on birth weight, BW, and composition of the offspring born to obese mothers. However, both maternal and weaning diets influenced parameters related to glucose metabolism. While numerous studies support the impact of maternal HPDs on health outcomes for both mothers and offspring, Reference Zhang, Wang, Terroni, Cagampang, Hanson and Byrne9–Reference Daenzer, Ortmann, Klaus and Metges11,Reference Rush, Kristal and Navarro18 these studies predominantly focus on normal-weight mothers. In normal weight dams, a HPD resulted in higher BW, Reference Zhang, Wang, Terroni, Cagampang, Hanson and Byrne9–Reference Thone-Reineke, Kalk and Dorn10 blood pressure, and food utilization efficiency, Reference Thone-Reineke, Kalk and Dorn10 and reduced energy expenditure Reference Daenzer, Ortmann, Klaus and Metges11 in the offspring. In a study on Wistar rats, female offspring born to mothers fed a HPD fed during pregnancy higher BW at puberty that sustaining until the end of the study (week 22) was observed Reference Thone-Reineke, Kalk and Dorn10 Moreover, in humans, protein supplementation (40g/day) during pregnancy resulted in lower birth weight compared to those offspring born to mothers fed a low-protein supplement (6g/day) beverage. Reference Rush, Kristal and Navarro18
Notably, limited research has examined the effects of maternal HPD and NPD in obese dams. In a clinical study, obese mothers on a high-protein, low-glycemic index diet exhibited lower gestational weight gain compared to those on a NPD. Contrary to expectations, our study found no discernible impact of a high-protein maternal diet on food intake, BW, or composition in mothers and their offspring, aligning with results reported in human studies. Reference Geiker, Magkos and Zingenberg29
Contrary to expectations, the findings of this study revealed no significant impact of a high-protein maternal diet on food intake, BW, or body composition in mothers and their offspring. However, the fat/BW ratio was reaching significance at weaning when the ratio was higher in rats born to NPD dams compared with those born to HPD dams (p = 0.06). This favorable impact of the HPD on body composition is consistent with previous studies when paternal HPD improved body composition in offspring compared with those born to fathers fed a high-fat/sucrose diet in rats Reference Chleilat, Schick and Deleemans30 However, this effect was disappeared at week 17 PW.
Additionally, there was no effect on birthweight aligning with the results reported by Geiker et al. in human studies Reference Geiker, Magkos and Zingenberg29 Gestational obesity’s universal impact on pregnancy outcomes, irrespective of protein source or quantity in the maternal diet, is a potential explanation for these findings. Gestational obesity increases the likelihood of gestational hypertension and GDM in mothers Reference Catalano and Shankar20 while increases the risk of obesity and cardiometabolic disease in offspring in later life Reference Kislal, Shook and Edlow24 These consequences are thought to stem from insulin resistance and inflammatory mediators in obese mothers that may potentially affect fetal development. Reference Poblete and Olmos25
While maternal obesity or GDM can elevate the likelihood of offspring developing obesity and/or glucose intolerance, Reference Leddy, Power and Schulkin31 the absence of a control group consisting of dams with a normal weight in this study prevents us from definitively interpreting these results as indicative of the impact of gestational obesity on the phenotype of the offspring. Nevertheless, these findings imply that the impact of maternal and weaning HPDs is disguised by gestational obesity, as observed across various indicators. This stands in contrast to earlier studies focused on dams with normal weight.
The result of this study supports the favorable effects of high-protein maternal and weaning diets on glucose metabolism in both obese mothers and in their offspring compared with NPD. These results align with existing research indicating the positive effects of a HPD on glucose metabolism in both clinical and animal studies. Reference Zhang, Wang, Terroni, Cagampang, Hanson and Byrne9–Reference Daenzer, Ortmann, Klaus and Metges11,Reference Tettamanzi, Bagnardi and Louca32–Reference Samkani, Skytte and Kandel34 In a clinical study, HPD improved insulin resistance and HOMA-IR in obese women received HPD compared with those received a Mediterranean diet. Reference Tettamanzi, Bagnardi and Louca32 In rats, paternal HPD improved body composition and insulin sensitivity in offspring compared with those born to fathers fed a high-fat/sucrose diet. Reference Chleilat, Schick and Deleemans30 Notably, weaning on a HPD improved glucose metabolism, as evidenced by reduced HOMA-IR at week 17 PW, consistent with established findings on the beneficial impact of HPDs on glucose metabolism.
The outcomes of this study do not align with the PAR hypothesis. Based on PAR, offspring exposed to a weaning diet corresponding to the maternal diet would have more suitable adaptations to the postnatal environment than those exposed to an uneven diet. Reference Gluckman and Hanson4 Evidently, pups born to mothers on a HPD or NPD weaned on the same diet, did not show any superior adaptation compared to those subjected to unmatched maternal and weaning diets.
While it is quite difficult logistically, a study encompassing both normal-weight and obese mothers (forming four groups of mothers and eight groups of pups) could help to compare the outcomes between obese and normal-weight pregnant mothers, as well as their pregnancy outcomes. This study’s limitations include the necessity to compare its results with previous studies on normal-weight mothers conducted at different times and settings. Furthermore, extending the study’s duration may facilitate understanding the long-term consequences associated with gestational obesity in both mothers and offspring. The observed significant effect of time on various measured parameters supports this conclusion.
Furthermore, it’ commonly understood that to formulate a HPD, adjustments in the levels of other macronutrients are necessary. In the specific HPD examined in this study, fat intake was reduced (40g compared to 70g of soybean oil in HPD and NPD, respectively), and carbohydrate intake was also lower (213.8g compared to 397g of corn starch, and 45.7 g compared to 100g of sugar in HPD and NPD, respectively). Hence, the outcomes of this study can also be attributed to the decreased fat and carbohydrate contents in the HPD.
Although the direct applicability of these findings to humans is not possible, they may serve as a foundational basis for future clinical trials, given the shared metabolic and physiologic mechanisms between rodents and humans.
Conclusion
In conclusion, despite gestational obesity diminishing or concealing the impact of maternal HPDs on measured variables in contrast to prior studies on normal-weight dams, the result of this study suggests that the protein content of the diet remains a significant factor influencing pregnancy outcomes. This is valid even in the face of the substantial effects of gestational obesity on both mothers and their offspring.
Supplementary material
The supplementary material for this article can be found at https://doi.org/10.1017/S2040174424000254.
Acknowledgments
The author would like to extend his sincere appreciation to the following students who served as Research Assistants in this study: Jennifer Wihlborg, Leila Ninya, Kea Schwarz, and Tatyana Kimble.
Author contributions
Alireza Jahan-mihan conceptualized and designed the study and conducted the study with research students, analyzed the data, interpreted the findings, and wrote the article.
Financial support
This study is supported by the Brooks College of Health, University of North Florida.
Competing interests
None.