Introduction
In temperate regions such as Ireland, the UK, New Zealand and parts of Australia pasture-based milk production systems predominate due to the abundance of pasture that can be grown (Roche et al., Reference Roche, Berry, Bryant, Burke, Butler, Dillon, Donaghy, Horan, Macdonald and Macmillan2017). With future feed costs projected to increase, using more grass in the diet of the lactating cow is a major objective for the Irish dairy industry. While grazed grass is the cheapest feed available (Hanrahan et al., Reference Hanrahan, McHugh, Hennessy, Moran, Kearney, Wallace and Shalloo2018), it has also been shown to increase milk production (Dillon et al., Reference Dillon, Crosse, O'Brien and Mayes2002) and milk protein concentration (Kennedy et al., Reference Kennedy, O'Donovan, Murphy, Delaby and O'Mara2005) in early lactation when compared to grass silage feeding. According to McEvoy et al. (Reference McEvoy, Kennedy, Murphy, Boland, Delaby and O'Donovan2008), targeting the early-lactation period for increasing grass input may eliminate the requirement to offer grass silage to animals, and depending on grass availability, concentrate supplementation level may also be reduced.
The variable and seasonal nature of pasture growth and feed quality, however, is a significant challenge in pasture-based farming in temperate regions (Roche et al., Reference Roche, Berry, Bryant, Burke, Butler, Dillon, Donaghy, Horan, Macdonald and Macmillan2017). In Ireland there is little net grass growth during the winter period from November to February (Brereton et al., Reference Brereton, Carton and O'Keeffe1985), and subsequently, grass supply in late autumn and early spring is generally not sufficient to meet herd demand (McEvoy et al., Reference McEvoy, Kennedy, Murphy, Boland, Delaby and O'Donovan2008). As a result of this grass deficit, grass silage and concentrates have previously constituted a large proportion of the early-lactation diet in spring and autumn-calving dairy cows (Kennedy et al., Reference Kennedy, O'Donovan, Murphy, Delaby and O'Mara2005, Reference Kennedy, Lewis, Murphy, Galvin and O'Donovan2015). Developments in autumn and spring pasture management have led to improved grass utilization and cow performance (O'Donovan et al., Reference O'Donovan, Geoghegan, Hennessy and O'Leary2015; Claffey et al., Reference Claffey, Delaby, Boland and Egan2020); however, grass supply is still limited during these periods, even in an optimal scenario (McEvoy et al., Reference McEvoy, Kennedy, Murphy, Boland, Delaby and O'Donovan2008).
A steady increase in the use of zero-grazing has been recently reported especially in the UK, Germany, Holland and the USA (Agrisearch, 2018; Cameron et al., Reference Cameron, Chagunda, Roberts and Lee2018). According to a recent survey (Holohan et al., Reference Holohan, Russell, Mulligan, Pierce and Lynch2021), an increasing number of dairy farmers in Ireland are choosing to use zero-grazing to overcome grass supply deficits. In this system, grass is cut using a purpose-built machine and transported to the farmyard where it is fed directly to the herd indoors (Agrisearch, 2018). This enables farmers to increase the quantity of grass available to the herd by utilising grass from external land parcels that are detached from the main grazing platform. On most of the farms surveyed, zero-grazing was carried out on a short-term basis, typically in spring or autumn, to reduce silage and concentrate feed requirements (Holohan et al., Reference Holohan, Russell, Mulligan, Pierce and Lynch2021).
Previous studies have suggested that there may be some additional benefits to zero-grazing, in terms of cow performance when compared to conventional grazing, with increases in dry matter intake (DMI) and milk production reported (van Vuuren and van den Pol-van Dasselaar, Reference van Vuuren, van den Pol-van Dasselaar, Elgersma, Dijkstra and Tamminga2006; Boudon et al., Reference Boudon, Peyraud, Faverdin, Delagarde, Delaby and Chaves2009; Dohme-Meier et al., Reference Dohme-Meier, Kaufmann, Görs, Junghans, Metges, van Dorland and Münger2014). The potential of zero-grazing to increase DMI is of particular interest in the context of the early-lactation period, when energy demand for production is higher than intake can support, often leading to cow health disorders and reduced fertility and performance (Mulligan and Doherty, Reference Mulligan and Doherty2008; Gilmore et al., Reference Gilmore, Young, Patterson, Wylie, Law, Kilpatrick and Mayne2011). There is however disagreement in the literature around the impact of zero-grazing on cow performance, with some studies noting lower DMI and milk yield in zero-grazed cows compared to grazing cows (Mohammed et al., Reference Mohammed, Stanton, Kennelly, Kramer, Glimm, O'Donovan and Murphy2009), and higher incidences of lameness (Haskell et al., Reference Haskell, Rennie, Bowell, Bell and Lawrence2006) and mastitis (Arnott et al., Reference Arnott, Ferris and O'Connell2015) with indoor housing systems.
The inconsistency in the current literature and overall lack of research on zero-grazing necessitates further investigation, particularly in an Irish context where interest in this feeding method has increased in recent years. The objective of this research therefore was to determine the effect of zero-grazing on the early-lactation performance of both autumn and spring-calving dairy cows in comparison to conventional pasture grazing. The experimental hypothesis was that zero-grazed cows would have higher DMI and milk yield.
Materials and methods
Location and management
Two experiments were carried out at University College Dublin's Lyons Farm, Kildare, Republic of Ireland (53°17′56″N, 6°32′18″W). In both experiments cows were assigned to one of two treatments: zero-grazing (ZG) and grazing (G). The ZG cows were housed full time in a free stall barn and fed zero-grazed grass, while the G cows grazed outdoors at pasture full time. During the experimental periods, grazing and zero-grazing was carried out simultaneously within the same paddock to ensure uniformity of grass quality offered to both groups. Cows in experiment 1 were fed a diet of concentrates, fresh grass and a buffer feed consisting of grass silage and maize meal while cows in experiment 2 were fed concentrates and fresh grass only. These are described in more detail later.
Cows in the G treatment were grazed together in a single group in a strip-grazing system, to enable a fresh allocation of pasture twice daily. Back fences on previously grazed areas were used to prevent cows grazing regrowth. Grass was allocated to achieve a target post grazing sward height of 4.5–5 cm. Herbage growth and supply was estimated twice weekly, using a rising plate meter (diameter 355 mm; 3.2 kg/m2; Jenquip, Feilding, New Zealand) by walking in a W shape across the experimental fields and measurements were entered into the PastureBase Ireland software system (Hanrahan et al., Reference Hanrahan, Geoghan, O'Donovan, Griffith, Ruelle and Shaloo2017), while daily pasture allocations were based on pre-grazing herbage mass as measured daily using the quadrat and shears method. Pasture allowance was adjusted daily to cater for the increasing demand of the cows based on stage of lactation with a continuing target post grazing sward height of 4.5–5 cm. Thus, the +0.5–1.5 cm additional post grazing residual above the recommended target for the first and last grazing rotation (O'Donovan and McEvoy, Reference O'Donovan and McEvoy2016) ensured that they were not restricted and was as similar as possible to the ad libitum allowance offered to the ZG treatment. In the ZG treatment, grass was harvested to 4 cm at 10:00 h daily using a specialized zero-grazing machine (Zero Grazer, Oldcastle, Ireland), and placed at the feed face where the cows were housed. The grass was manually moved closer to the feed face at regular intervals throughout the day to ensure cows had ad libitum access to grass at all times. A feed space allocation of 700 mm per cow was used to enable all cows to feed simultaneously at any one time. The floor surface of the barn was kept clean using an automatic scraper system (CleanSweep; Dairymaster, Kerry, Ireland) which removed dung and urine at regular intervals throughout the day, and free stalls were cleaned and bedded with a mixture of hydrated lime and sawdust twice daily.
Experiment 1: the effect of zero-grazing on early-lactation performance in autumn-calving cows
This 7-week study was conducted between 1 October and 18 November 2018. Twenty-four autumn-calving Holstein Friesian dairy cows in early lactation were blocked on days in milk (28 ± 13.3), parity, predicted 305-day yield, body condition score (BCS) and body weight (BW), and assigned to one of two treatments in a randomized complete block design (n = 12). Prior to being assigned to their respective treatment groups, all cows were fed a diet of grazed grass and 8 kg concentrates, with concentrate feeding stepped up from 4 to 8 kg in the first 7 days post-partum. Cows were offered the experimental diets for a 14-day dietary acclimatization period. Following this, cows remained on their treatments for a further 35-day experimental period. Both treatment groups were offered the same daily diet which consisted of ad libitum quantities of perennial ryegrass (Lolium perenne L.), 7.2 kg dry matter (DM) concentrates fed in the parlour, 2.5 kg DM grass silage and 1.8 kg DM ground maize. Concentrate ingredients are highlighted in Table 1. The grass silage and ground maize were fed to both G and ZG groups directly before milking each day using individual pre-programmed feed boxes (RIC System; Insentec B.V., Marknesse, the Netherlands). In preparation for the study, paddocks that were previously rotationally grazed by dairy cows were mechanically cut to a height of 4 cm in the previous rotation and grass was harvested for baled silage. This prevented the risk of sward contamination from dung pads left by grazing livestock as per current guidelines (Agrisearch, 2018). Average pre-cutting/pre-grazing yield over the experimental period was 2024 kg DM/ha (± 557) (>4 cm). Post-cutting sward height for ZG was 4 cm, while post-grazing sward height for G was 5.3 cm. Mean total distance walked per day between paddock and milking parlour for G group was 1.6 km (±0.37) for the experimental period.
Table 1. Ingredient composition of concentrate fed in experiments 1 and 2
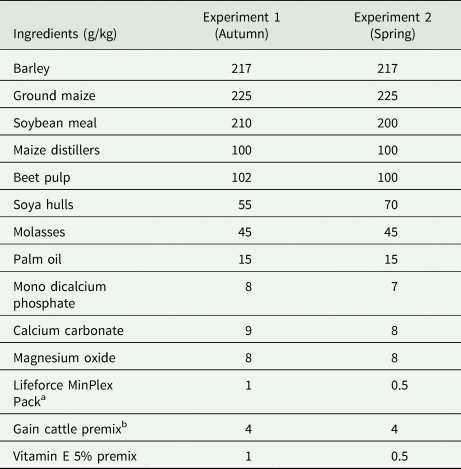
a Alltech, Nicholasville, KY, USA.
b Gain Feeds, Portlaoise, Ireland.
Experiment 2: the effect of zero-grazing on early-lactation performance in spring-calving cows
This 7-week study was conducted between 17 March and 6 May 2020. Thirty spring-calving Holstein-Friesian dairy cows in early lactation were blocked on days in milk (37 ± 6.1), parity, pre-experimental milk yield, predicted 305-day yield, BCS, locomotion score and BW, and assigned to one of two treatments in a randomized complete block design (n = 15). Cows were offered the experimental diets for a 10-day dietary acclimatization period. Following this, cows remained on their treatments for a further 42-day experimental period. Both treatment groups were offered the same diet which consisted of perennial ryegrass (L. perenne L.), and 7.2 kg DM concentrates fed in the parlour. Concentrate ingredients are highlighted in Table 1. Average pre-cutting/pre-grazing yield over the experimental period was 1680 kg DM/ha (±221) (>4 cm) for both ZG and G. Post-cutting sward height for ZG was 4.5 cm, while post-grazing sward height for G was 4.6 cm. Mean total distance walked per day between paddock and milking parlour for G group was 2.4 km (±0.63).
Animal measurements
All procedures described in this experiment were approved by the Animal Research Ethics Committee of University College Dublin and were conducted under experimental license from the Health Products Regulatory Authority under the European directive 2010/63/EU and S.I. No. 543 of 2012. The respective project licence numbers for experiments 1 and 2 were AE18982/P144 and AE18982/P175. Each person who carried out procedures held an individual authorization from the Health Products Regulatory Authority.
Animals were milked twice daily at 07.00 and 16.00 h. Milk yield and milk sampling were facilitated by using a milk metering and sampling system (Weighall; Dairymaster). Samples of milk were taken weekly during consecutive a.m. and p.m. milkings and pooled according to individual daily milk yield to determine weekly milk composition and quality. Animal BW was measured twice daily after milking using an automatic weighing system (Dairymaster) and averaged on a daily basis. Locomotion scoring was performed on a bi-weekly basis using a five-level ordinal scale (1 = healthy; 5 = severely lame) as described by Sprecher et al. (Reference Sprecher, Hostetler and Kaneene1997). BCS was measured bi-weekly and determined using a five-point scale (1 = emaciated; 5 = obese) with quarter-point increments (Edmonson et al., Reference Edmonson, Lean, Weaver, Farver and Webster1989) and evaluated by the same trained researcher.
Pasture DMI and N excretion was determined over a 6-day period for both treatments during week 4 of experiment 1 (48 ± 13.3 DIM; mean ± sd) and during week 3 in experiment 2 (58 ± 6.1 DIM; mean ± sd). Pasture DMI was determined using the n-alkane technique of Dove and Mayes (Reference Dove and Mayes2006). Briefly, cows were orally dosed with a paper bolus impregnated with 500 mg of the n-alkane n-dotriacontane (C32) for a period of 12 days following a.m. and p.m. milking. From day 6 to 12, samples of the concentrates, pasture, milk and faeces were collected. Pasture samples were immediately dried at 55°C for 48 h. Faecal samples were collected, whenever possible, when cows naturally defecated, and, if not, samples were collected per rectum and placed in a forced-air oven at 55°C for 72 h or until dry. Samples of milk were collected during a.m. and p.m. milking and pooled according to milk yield.
In experiment 1, blood samples were harvested by jugular venepuncture once weekly for determination of glucose, NEFA and BHB. Blood samples for glucose were harvested into a 4 ml Vacutainer tube containing NaF (6 mg) and Na2EDTA (12 mg; Ref. No. 368521; BD, Plymouth, UK) and centrifuged at 2100 × g for 20 min at 4°C for extraction of plasma. These samples were stored at −20°C pending analysis. Blood samples for other analytes were harvested into a 10 ml Vacutainer (Ref. No. 8303209; BD) and allowed to clot for 16 h at 4°C before centrifuging at 2100 × g for 20 min at 4°C for extraction of serum. These samples were also stored at −20°C pending analysis. In experiment 1 rumen fluid samples were collected weekly via Flora Rumen scoop oral oesophageal sampler (Prof-Products, Guelph, ON, Canada) and samples were analysed immediately for pH (EC-25 pH/Conductivity Portable Meter, Phoenix Instrument, Garbsen, Germany). Samples were strained through four layers of cheesecloth. An 8 ml sample was drawn off, mixed with 2 ml of 50% (wt/vol) TCA and stored at −20°C pending determination of NH3N concentrations.
Pasture and feed measurements
Samples of herbage were collected daily and pooled weekly, while concentrate samples were collected weekly during the trial period. In the case of experiment 1, grass silage and ground maize samples were also collected weekly. Pasture and feed samples were dried at 55°C for 72 h in a forced-air oven to determine DM. For chemical composition analysis daily snip samples were taken using a hand-held shears (Gardena Accu 90, Gardena GmbH, Ulm, Germany) to a height of 4 cm at random locations in the paddock. A 100 g subsample was then taken for determination of DM, ash, crude protein (CP), neutral detergent fibre (NDF), acid detergent fibre (ADF), organic matter digestibility (OMD) and water-soluble carbohydrate (WSC) content (Table 2).
Table 2. Chemical composition of pasture, supplemental concentrates, grass silage and ground maize offered during the experiments 1 and 2
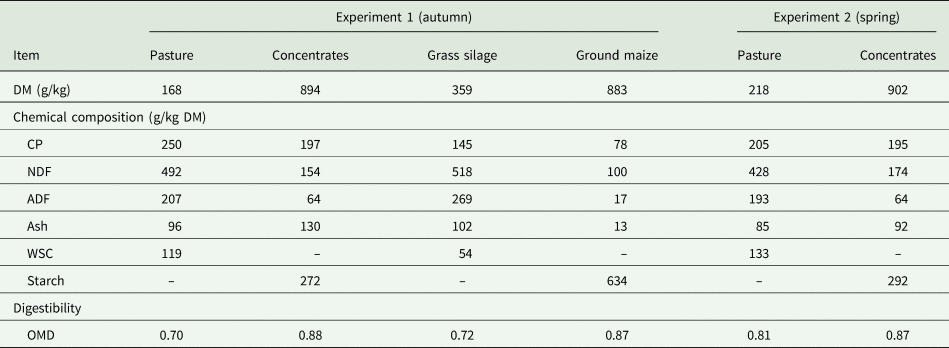
CP, crude protein; WSC, water-soluble carbohydrates; OMD, digestibility of OM.
Meteorological data
Meteorological data on rainfall, air temperature and soil moisture deficit were recorded for the duration of the experiments at Casement Aerodrome (53°30′N, −6°44′W), approximately 5.8 km east of Lyons Farm, by the Irish Meteorological Service.
Experiment 1
As outlined in Table 3, total rainfall for the period from 1 October to 18 November was 108 mm in 2018, which was 27% lower than the 10-year average for the same period. The number of ‘wet days’ (>1 mm rainfall) for the period was 16, which was 20% lower than the 10-year average. Daily mean air temperature was 9.2°C, marginally lower than the 10-year average of 9.5°C, while soil moisture deficit was 15.41 mm, which was 2.4 times higher than the 10-year average.
Table 3. Total rainfall, average daily air temperature and daily soil moisture deficit (mm) at the Casement Aerodrome weather station for experiments 1 and 2, and the previous 10-year average for each experimental period

a Met Éireann (2022).
Experiment 2
Total rainfall for the period from 17 March to 6 May was 25 mm in 2020, which was 72% lower than the 10-year average for the same period (Table 3). The number of ‘wet days’ (>1 mm rainfall) for the period was 7, which was 56% lower than the 10-year average. Daily mean air temperature was 8.5°C, which was marginally higher than the 10-year average of 7.9°C, while soil moisture deficit was 28.5 mm, which was 2.5 times higher than the 10-year average.
Sample analysis
Feed and faecal sample analysis
Dried pasture, concentrate, grass silage, ground maize and faecal samples were ground in a hammer mill fitted with a 1 mm screen (Lab Mill; Christy Turner, Suffolk, UK). The DM content of samples was determined after drying overnight at 105°C (16 h minimum) (AOAC International, 2005, method 930.15). The ash content was determined following combustion in a muffle furnace (Nabertherm GmbH, Lilienthal, Germany) at 550°C for 5.5 h (AOAC International, 2005, method 942.05). The N content of samples was determined by combustion on Leco and CP content calculated (N × 6.25; FP 528 Analyzer, Leco Corp., St. Joseph, MI, USA; AOAC International, 2005, method 990.03). Neutral detergent fibre and ADF were determined using the method Van Soest et al. (Reference Van Soest, Robertson and Lewis1991) adapted for use in the Ankom 220 Fiber Analyzer (Ankom Technology, Macedon, NY, USA). Samples were not subjected to combustion after fibre extraction; therefore, the values reported include ash. No Na2SO4 was used in the procedure. However, a thermostable α-amylase (FAA, 17 400 Liquefon Units/ml; Ankom Technology) was included when concentrate NDF was determined. Starch was determined using the Megazyme Total Starch Assay Procedure (Product No. K-TSTA; Megazyme International Ireland Ltd., Wicklow, Ireland). The concentration of WSC was determined as described by DuBois et al. (Reference DuBois, Gilles, Hamilton, Rebers and Smith1956). In vitro digestibility of organic matter of pasture and concentrates offered was determined using a modification of the method of Tilley and Terry (Reference Tilley and Terry1963) for use in the Ankom Daisy Incubator (Ankom Technology). In experiment 2, NDF, ADF, WSC and starch were determined using near infrared spectrometry (Burns et al., Reference Burns, Gilliland, Grogan and O'Kiely2011).
Milk, blood and rumen sample analysis
Concentrations of milk fat, protein, lactose and SCC were determined in a commercial milk laboratory (Progressive Genetics, Dublin, Ireland) using infrared analysis (CombiFoss 5000, Foss Analytical A/S, Hillerød, Denmark; Soyeurt et al., Reference Soyeurt, Dardenne, Dehareng, Lognay, Veselko, Marlier, Bertozzi, Mayeres and Gengler2006). Blood samples were analysed for NEFA (Kit No. FA115) and BHB (Kit No. RB1007) using enzymatic tests. Glucose (Kit No. GL3816) was analysed using the hexokinase test. All kits were sourced from Randox Laboratories Ltd (Crumlin, County Antrim, UK). All blood analysis was carried out using a clinical blood analyser (RX imola; Randox Laboratories Ltd). Rumen fluid was allowed to thaw for 16 h to 4°C, and then centrifuged at 2100 × g for 10 min at 4°C. One millilitre of supernatant was drawn off and diluted 1:5 with distilled H2O, and then centrifuged at 1600 × g for 15 min at 4°C, and 200 μl of supernatant was drawn off and used to determine NH3N concentrations according to the phenol-hypochlorite method of Weatherburn (Reference Weatherburn1967).
Pasture dry matter intake
Pasture DMI was determined by extracting n-alkanes from pasture, concentrate and faeces samples according to the method of Dove and Mayes (Reference Dove and Mayes2006). Following extraction, samples were analysed for concentrations of n-alkanes by GC using a Varian 3800 GLC (Varian Inc., California) fitted with a 30 m capillary column with an internal diameter of 0.53 mm, coated with 0.5 μm of dimethyl polysiloxane (SGE Analytical Science Pty Ltd., Ringwood, Australia).
Nitrogen partitioning
After DMI was calculated, the data were used to calculate N partitioning as follows: N intake (g) = [(kg of pasture DMI × g of N/kg of DM pasture) + (kg of concentrate DMI × g of N/kg of DM concentrate) + (kg of grass silage DMI × g of N/kg of DM grass silage) + (kg of ground maize DMI × g of N/kg of DM ground maize)]; faecal N (g) = kg of faecal DM excretion × g of N/kg of DM faeces; milk N (g) = kg of milk yield × g of N/kg of milk; and urine N (g) = N intake (g) − faecal N (g) − milk N (g). Faecal excretion was calculated from the indigestibility of the diet fed while urine N was calculated by subtracting N in milk and faeces from N intake (Mulligan et al., Reference Mulligan, Dillon, Callan, Rath and O'Mara2004).
Statistical analysis
Data were analysed as a complete randomized block design using the mixed model procedure (PROC MIXED) in SAS (SAS, version 9.4, Inst. Inc., Cary, NC, USA). The natural logarithm transformation of milk SCC was used to normalize the distribution. The transformed data were used to calculate P values. Data distributions were analysed to fit the assumptions of normality using the UNIVARIATE procedure of SAS. Individual cow was the experimental unit. Analysis included tests for the fixed effects of treatment and week, and their interactions. The following model was used:

where Yijklm is the variable of interest, μ is the overall mean, Ti is the fixed effect of treatment i, Wj is the fixed effect of week j, TWij is the fixed interaction of treatment i and week j, and ɛijklm is the residual error.
Statistically significant differences between least squares means were tested using the PDIFF command incorporating the Tukey test for pairwise comparison of treatment means. Statistical significance was assumed at a value of P < 0.05 and a tendency towards significance assumed at a value of P > 0.05 but <0.10.
Results
Experiment 1: the effect of zero-grazing on early-lactation performance in autumn-calving cows
Pasture and feed quality
Chemical composition of pasture, supplemental concentrates, grass silage and ground maize offered during the experimental period is outlined in Table 2. Average grass DM content was 168 g/kg, with OMD of 0.70, CP of 250 g/kg DM and NDF of 482 g/kg DM. Average grass silage DM was 349 g/kg, with OMD of 0.72, CP of 145 g/kg DM and NDF of 518 g/kg DM.
DMI, milk production, milk composition
The effects of grazing method on DMI, milk production, milk composition, animal BW, BCS and locomotion score are shown in Table 4. Zero-grazing did not have a significant effect on DMI in this study. Similarly, it had no effect on milk yield or milk composition.
Table 4. Effects of grazing method on DM intake, milk production, milk composition, animal BW, body condition and locomotion in comparison to in pasture grazing
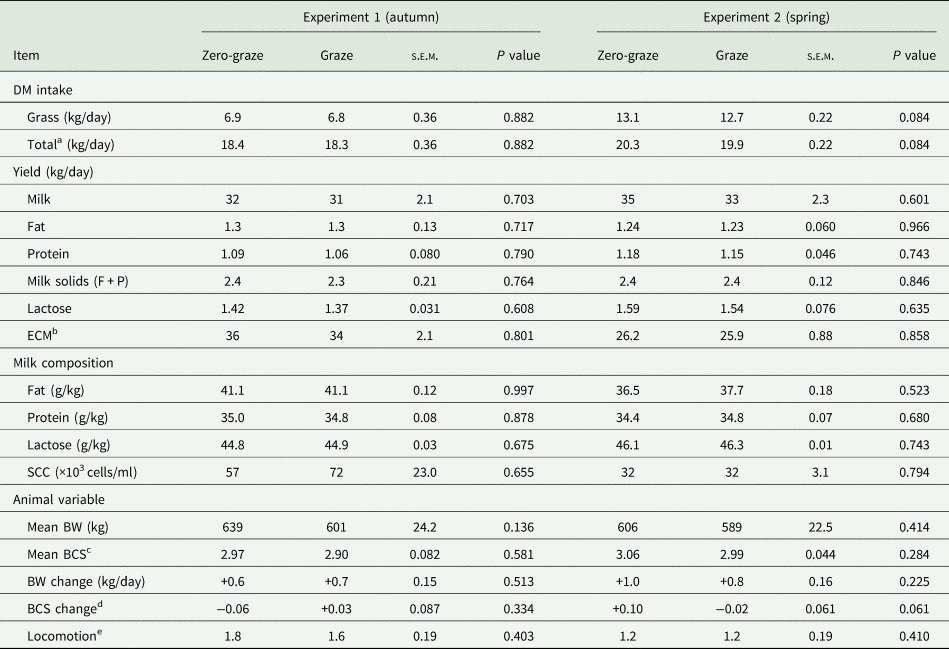
a Total DM intake = grass + 7.2 kg concentrates + 2.5 kg silage + 1.8 maize meal for experiment 1; grass + 7.2 kg concentrates for experiment 2.
b Energy corrected milk calculated as per Tyrrell and Reid (Reference Tyrrell and Reid1965).
c BCS was on a scale of 1–5 in 0.25 increments (Edmonson et al., Reference Edmonson, Lean, Weaver, Farver and Webster1989).
d BCS change from beginning to end of experimental period.
e Locomotion was on a scale of 1–5 (Sprecher et al., Reference Sprecher, Hostetler and Kaneene1997).
Cow BW, BCS and locomotion
As outlined in Table 4, there was no significant difference in BW and BCS between the ZG and G treatments. In addition, treatment had no effect on locomotion score.
Nitrogen partitioning
The effects of grazing method on nitrogen partitioning are shown in Table 5. Dietary N intake did not differ significantly between treatments. Zero-grazed cows partitioned a greater proportion of N to faeces compared to G cows (P = 0.009); however, N percentage in urine and milk was similar for both treatments. In addition, zero-grazing had no effect on total N excretion or NUE.
Table 5. Effects of grazing method on nitrogen (N) partitioning in comparison to in pasture grazing
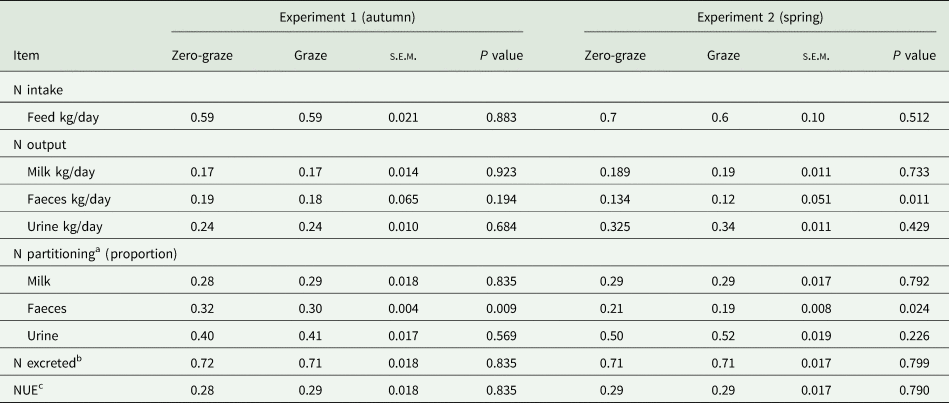
a N partitioning = N out {[faeces, urine, milk (kg/day)]/N intake (kg/day)}.
b N excreted = N out {[faeces + urine output (kg/day)]/N intake (kg/day)}.
c Nitrogen utilization efficiency = N out {[milk output (kg/day)]/N intake (kg/day)}.
Blood metabolites and rumen fermentation
The effects of grazing method on blood metabolites and rumen fermentation are shown in Table 6. Treatment had no effect on blood glucose, NEFA or BHB concentrations. Similarly, there were no significant differences between treatments for rumen pH or ammonia levels.
Table 6. Effect of grazing method on blood metabolites and rumen fermentation in comparison to in pasture grazing in experiment 1
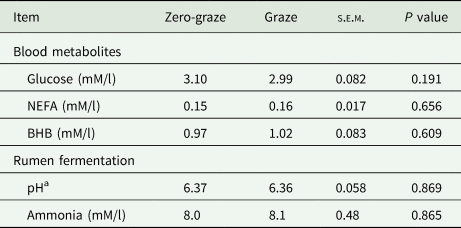
a Mean pH taken once weekly post afternoon milking.
Experiment 2: the effect of zero-grazing on early-lactation performance in spring-calving cows
Pasture and feed quality
Chemical composition of pasture and supplemental concentrates offered during the experimental period is outlined in Table 2. Average grass DM content was 218 g/kg, with OMD of 0.81, CP of 205 g/kg DM and NDF of 428 g/kg DM.
DM intake, milk production and milk composition
The effects of grazing method on DMI, milk production and milk composition are shown in Table 4. Grass and total DMI tended to be higher in the ZG than G cows (13.07 v. 12.66 kg/day and 20.27 v. 19.86 kg/day; P = 0.081 respectively). Average daily milk production did not significantly differ. In terms of milk composition (milk fat, protein and lactose percentage and yield) there was no significant difference between treatments. Energy corrected milk (ECM) yield was also similar between treatments. Somatic cell count was similar in both ZG and G (31 600 v. 32 400 cells per ml).
Cow BW, BCS and locomotion
Average BW was not significantly different between treatments, while daily BW change between the start and end of the experiment was similar. Mean BCS was similar; however, BCS change between the start and end of the experimental period tended to be higher in ZG than G cows (+0.10 v. −0.02; P = 0.061). Average locomotion score was the same for both treatments.
Nitrogen partitioning
The effects of grazing method on nitrogen partitioning are shown in Table 5. Dietary N intake did not differ significantly between treatments. Zero-grazed cows partitioned a greater proportion of N in faeces (0.21 v. 0.19; P = 0.024) and subsequently excreted a higher amount of N to faeces than G cows (0.134 v. 0.120 kg/day; P = 0.011). The proportion of N partitioned in milk and urine was similar for both treatments. The kilograms of N excreted in milk and urine were also similar. In addition, zero-grazing did not have an effect on total N excretion or nitrogen utilization efficiency.
Discussion
In Ireland, some dairy farmers choose to use zero-grazing to supply the herd with fresh grass from land parcels that are outside of the main grazing block during seasonal shortages or when in situ grazing is not possible due to adverse weather conditions (Holohan et al., Reference Holohan, Russell, Mulligan, Pierce and Lynch2021). Given the lack of understanding on the effect that this feeding method has on cow performance, the objective of the current study was to determine the effect of zero-grazing on cow performance in early-lactation dairy cows during both the autumn and spring, in comparison to conventional grazing. The experimental hypothesis was that zero-grazed cows would have higher DMI and milk yield. The results of the study show that cows offered zero-grazed grass had similar DMI and milk production compared to conventionally grazed cows; therefore, the hypothesis can be rejected.
DM intake, milk production, milk composition, animal BW, BCS and locomotion
It is recognized that DMI is the primary factor governing the ability of cows to meet their nutritional needs, particularly in early lactation (Allen, Reference Allen2000; Jorritsma et al., Reference Jorritsma, Wensing, Krui, Lam, Vos and Noordhuizen2003; Jouany, Reference Jouany2006). While grazing plays an important role in Ireland's pasture-based dairy production model, achieving high levels of DMI can be challenging (Wilkinson et al., Reference Wilkinson, Lee, Rivero and Chamberlain2019). According to Bargo et al. (Reference Bargo, Muller, Kolver and Delahoy2003), if the aim is to maximize pasture DMI of high producing dairy cows, management must ensure unrestricted pasture quality and quantity; however, this is not always possible during the autumn and spring periods. Zero-grazing has previously been reported to increase DMI in comparison to grazing cows (van Vuuren and van den Pol-van Dasselaar, Reference van Vuuren, van den Pol-van Dasselaar, Elgersma, Dijkstra and Tamminga2006; Boudon et al., Reference Boudon, Peyraud, Faverdin, Delagarde, Delaby and Chaves2009; Dohme-Meier et al., Reference Dohme-Meier, Kaufmann, Görs, Junghans, Metges, van Dorland and Münger2014). The reason, according to Oshita et al. (Reference Oshita, Sudo, Nonaka, Kume and Ochiai2008) and Dohme-Meier et al. (Reference Dohme-Meier, Kaufmann, Görs, Junghans, Metges, van Dorland and Münger2014), is that cows grazing pasture require more time to ingest the same amount of herbage as zero-grazed cows, and this can therefore limit DMI when the diet largely consists of fresh grass. Dohme-Meier et al. (Reference Dohme-Meier, Kaufmann, Görs, Junghans, Metges, van Dorland and Münger2014) fed a diet consisting of 0.78 grass and found significantly higher DMI in zero-grazed cows. In the current study, fresh grass made up 0.37 of the diet in experiment 1 and 0.65 in experiment 2, and DMI was similar and not statistically different between ZG and G cows in either experiment. In both experiments the cows were offered 7.2 kg DM concentrate per day. These levels are consistent with the energy requirements of the herd, expected grass intake of pasture and fed to meet energy requirements. Such daily feed allowances include approximately 15 UFL to come from grass and 7 UFL to come from concentrate with typical energy requirements in early lactation of 22 UFL per day. Such early-lactation concentrate allowances have been found to be consistent with good pasture utilization in high output grazing systems. There was however, a tendency in experiment 2 for higher DMI in ZG cows, which may indicate a potentially positive relationship between the proportion of grass in the diet and DMI in zero-grazed cows compared to grazing cows. Thus, lower concentrate allowances may induce an increase in DMI in ZG cows compared to conventionally grazed cows as previously reported (van Vuuren and van den Pol-van Dasselaar, Reference van Vuuren, van den Pol-van Dasselaar, Elgersma, Dijkstra and Tamminga2006; Boudon et al., Reference Boudon, Peyraud, Faverdin, Delagarde, Delaby and Chaves2009; Dohme-Meier et al., Reference Dohme-Meier, Kaufmann, Görs, Junghans, Metges, van Dorland and Münger2014).
Another reason for the tendancy for a higher DMI in ZG cows in experiment 2 maybe due to the lower post grazing sward height for the grazing treatment (4.5 v. 4.6 cm for ZG and G) in comparison to experiment 1 (4.0 v. 5.3 cm for ZG and G) respectively. Previous research by Ganche et al. (Reference Ganche, Delaby, O'Donovan, Boland, Galvin and Kennedy2013) reported an effect of post grazing sward height in the first 10 weeks of lactation in Ireland on grass DMI. Albeit, the treatment differences reported were a post grazing sward height of 2.7 (severe) having an impact on grass DMI in comparison to 3.5 (low) and 4.2 (moderate), which were unaffected.
For experiment 1, all the experimental paddocks were cut and baled for grass silage in the rotation prior to the start of the experiment. The current recommendation (Agrisearch, 2018) is to avoid zero-grazing pastures grazed within the previous month in order to prevent the risk of sward contamination from dung pads left by grazing livestock. For experiment 2, the experimental paddocks were ungrazed since the previous autumn. Thus, it is unlikely a longer rest period (as was the case in experiment 2) after grazing and prior to the onset of experiment 1, instead of cutting, would have resulted in a different outcome in the results.
It is worth noting that weather conditions during both experiments 1 and 2 were unseasonably mild for those particular periods in Ireland. In experiment 1 in autumn 2018, rainfall levels were 27% lower than the 10-year average. In addition, the average soil moisture deficit was 2.4 times higher than the 10-year average. In experiment 2 in spring 2020, rainfall was 72% lower than the 10-year average for the same period, and average soil moisture deficit was 2.5 times higher than the 10-year average. The low levels of rainfall and soil moisture provided more favourable grazing conditions than would be expected in the late autumn and early spring periods, and it is possible that less favourable conditions could have resulted in lower performance in the grazing cows. During inclement weather, grazing cows experience behavioural changes (Redbo et al., Reference Redbo, Ehrlemark and Redbo-Torstensson2001; Webster et al., Reference Webster, Stewart, Rogers and Verkerk2008), and spend less time lying and have shorter and fewer daily lying bouts when exposed to wet and cold conditions (Hendriks et al., Reference Hendriks, Phyn, Turner, Mueller, Kuhn-Sherlock, Donaghy, Huzzey and Roche2019). Leso et al. (Reference Leso, O'Leary, Werner, Kennedy, Geoghegan and Shalloo2018) concluded that rainfall in particular reduces both lying and rumination time in grazing cows in Ireland which could have implications for milk production.
Milk production and composition were similar in experiments 1 and 2 between the ZG and G treatments, and this was likely due to DMI and sward nutritive value being similar across treatments in both experiments. While there was a tendancy in experiment 2 for higher grass DMI in ZG cows, this did not significantly increase milk production or composition. There was however a tendency for ZG cows to increase BCS at a higher rate than G cows in experiment 2. While not statistically significant, this may have been caused by increased DMI or possible differences in energy expenditure between G and ZG cows, or a combination of these factors. It has been previously reported that animals at pasture walk longer distances and spend considerably more time eating and foraging for food than conventionally housed animals (Osuji, Reference Osuji1974), while Dohme-Meier et al. (Reference Dohme-Meier, Kaufmann, Görs, Junghans, Metges, van Dorland and Münger2014) found that grazing cows can consume up to 19% more energy than cows fed the same herbage in the barn (Dohme-Meier et al., Reference Dohme-Meier, Kaufmann, Görs, Junghans, Metges, van Dorland and Münger2014).
While housing cows indoors may offer some benefit to the animal, such as reduced exposure to extreme weather conditions (Schütz et al., Reference Schütz, Clark, Cox, Matthews and Tucker2010), there are also a number of challenges associated with the housed environment. According to Arnott et al. (Reference Arnott, Ferris and O'Connell2015) confined cows typically have higher SCC and higher levels of clinical mastitis compared to grazing cows, while Haskell et al. (Reference Haskell, Rennie, Bowell, Bell and Lawrence2006) reported higher risk of lameness and leg inury in confined systems. In the current study however, zero-grazing did not have an effect on SCC, with SCC across both treatments in experiments 1 and 2 averaging 64 500 and 32 000 cells/ml respectively. This is well within the target industry threshold of <200 000 cells/ml (Schukken et al., Reference Schukken, Wilson, Welcome, Garrison-Tikofsky and Gonzalel2003). Locomotion score, which is an estimate of the prevalence of lame cows in the herd (Whay, Reference Whay2002), was similar for both ZG and G cows, in the current study. According to Tadich et al. (Reference Tadich, Flor and Green2010) cows with scores of 1 or 2 are not usually considered lame. Mean locomotion scores across treatments in the current study were 1.7 and 1.2 in experiments 1 and 2 respectively, which were within acceptable levels. Results from the current study suggest that good udder and hoof health can be maintained when cows are housed indoors for a short period during spring or autumn.
Nitrogen partitioning
Large amounts (0.70–0.80) of ingested N are excreted from dairy cows, which can have a detrimental effect on the environment (Tamminga, Reference Tamminga1992; Ryan et al., Reference Ryan, Hennessy, Murphy, Boland and Shalloo2011). A greater problem in pasture-based systems, compared with confinement systems, is that a large proportion of N is lost to the environment through volatilization and leaching when cows are grazing outdoors (Hyde et al., Reference Hyde, Carton, O'Toole and Misselbrook2003; Casey and Holden, Reference Casey and Holden2005; Ryan et al., Reference Ryan, Hennessy, Murphy, Boland and Shalloo2011). In the current study, total N excretion and N use efficiency did not significantly differ between G and ZG cows in either experiment 1 or 2. The partitioning of N into milk and urine was similar; however, the percentage of ingested N excreted in faeces was higher in ZG cows in both experiments 1 and 2. According to Huhtanen et al. (Reference Huhtanen, Nousiainen, Rinne, Kytölä and Khalili2008), faecal N is derived from excretion of undigested feed N, undigested microbial N and endogenous N. It is possible that the excretion of one or more of these was higher in ZG; however, it is difficult to pinpoint which, if either, was the contributory factor, particularly given that there was no significant difference between total ingested N and total excreted N. From an environmental perspective, an increased faecal:urinary N ratio is desirable in grazing cows because large amounts of ammonia in urine are lost to volatilization after a urination event as well as losses of N2O during the denitrification process (Totty et al., Reference Totty, Greenwood, Bryant and Edwards2013). Faecal N is also more stable than urinary N and is less susceptible to leaching (Powell et al., Reference Powell, Broderick, Grabber and Hymes-Fecht2009). It must be noted however, that an increased faecal:urinary N ratio may not translate to lower ammonia emissions in zero-grazed cows. According to Moraes et al. (Reference Moraes, Burgos, DePeters, Zhang and Fadel2017) the enzyme urease (which is found in faeces) causes rapid conversion of urea in urine to ammonia and CO2 when urine and faeces mix together on the floor surface of indoor housing. As a result, ammonia volatilization is typically higher when cows are housed indoors than grazing outdoors (Hennessy et al., Reference Hennessy, Delaby, van den Pol-van Dasselaar and Shalloo2020). Advancements in manure handling such as low-emission slurry spreading may however reduce ammonia volatilization and N leaching from indoor housing.
Blood metabolites and rumen fermentation
Measurement of blood metabolites allows for the determination of discrete differences in the energy status of cows (Al Ibrahim et al., Reference Al Ibrahim, Kelly, O'Grady, Gath, McCarney and Mulligan2010). In experiment 1, blood glucose levels (averaging 3.05 mmol/l) in both treatments were above the value of 2.2 mmol/l, below which indicates hypoglycaemia (Ruoff et al., Reference Ruoff, Borchardt and Heuwiese2017). Blood concentrations of NEFA (averaging 0.16 mmol/l) across both treatments were within the acceptable values of <0.6 mmol/l after calving (Adewuyi et al., Reference Adewuyi, Gruys and van Eerdenburg2005), and were not affected by grass feeding method. Plasma concentrations of BHB in both treatments (averaging 1.0 mmol/l) were above the range of normal values which is typically between 0.1 and 0.6 mmol/l in early lactation (Raboisson et al., Reference Raboisson, Mounié and Maigné2014). However, according to Mulligan et al. (Reference Mulligan, O'Grady, Rice and Doherty2006) the alarm level threshold for negative energy balance in lactating cows is a BHB concentration in excess of 1.4 mmol/l. Both treatment groups in experiment 1 were below this threshold. It is probable that no differences were observed in NEFA and BHB concentrations between treatments because DMI was similar and there was no difference in BW or BCS.
Rumen fluid ammonia concentrations of G and ZG cows in experiment 1 were not significantly different. Rumen ammonia concentration between both treatments averaged 8.07 mmol/l, which was just within the optimum range of 3.0–8.5 mmol/l (McDonald et al., Reference McDonald, Edwards, Greenhalgh, Morgan, Sinclair and Wilkinson2012). Rumen pH of 6.37 and 6.36 for ZG and G respectively indicates that both treatment groups were not experiencing acidosis at the time of sampling. According to Kleen et al. (Reference Kleen, Hooijer, Rehage and Noordhuizen2003) sub acute ruminal acidosis (SARA) occurs when rumen pH is depressed for prolonged periods each day, e.g. <5.6 for >3 h/day (Kleen et al., Reference Kleen, Hooijer, Rehage and Noordhuizen2003), and it has been previously highlighted by farmers as a potential issue when using ZG (Holohan et al., Reference Holohan, Russell, Mulligan, Pierce and Lynch2021). The occurrence of SARA in cows is linked to the feeding of high amounts of ryegrass pasture (O'Grady et al., Reference O'Grady, Doherty and Mulligan2008), and it is likely that the percentage of fresh grass in the diet in experiment 1 (0.37 of total diet DM) was not large enough to induce acidosis. It is possible that feeding higher proportions of grass than the current study may have negative consequences for rumen pH. According to Kolver and de Veth (Reference Kolver and de Veth2002), rumen pH is positively related to pasture NDF, meaning that as NDF increases so too does rumen pH. Given that rumen pH remained unchanged between treatments in this experiment, it provides further indication that dietary NDF was similar for both treatments.
Conclusion
The majority of dairy farmers in Ireland that use zero-grazing do so on a short-term basis in spring and autumn. While zero-grazing may enable them to supply fresh grass to early-lactation cows, in replacement of other conserved forages, this study found no additional benefits to cow performance when comparing zero-grazing to well-managed grazed grass.
Author contributions
M. B. Lynch and C. Holohan conceived and designed the study. C. Holohan, N. A. Walsh, M. McDonald and J. Somers conducted data gathering. C. Holohan performed statistical analyses. C. Holohan, M. B. Lynch, K. M. Pierce and F. J. Mulligan wrote the article. M. B. Lynch, K. M. Pierce and F. J. Mulligan were responsible for funding acquisition and supervision of graduate student.
Financial support
This study was funded by the Irish Department of Agriculture, Food and the Marine Research Stimulus Fund under the NutriGen project (15S675).
Conflict of interest
None.
Ethical standards
All procedures described in this study were approved by the Animal Research Ethics Committee (AREC) of University College Dublin (UCD) and were conducted under the experimental license from the Health Products Regulatory Authority (HPRA) under the European directive 2010/63/EU and S.I. No. 543 of 2012. Each person who carried out procedures during these experiments held an individual authorization from the HPRA.