INTRODUCTION
Norovirus is the most common cause of acute non-bacterial gastroenteritis outbreaks worldwide [Reference Fankhauser1] (reviewed in [Reference Goodgame2, Reference Koopmans3]), typically manifesting with symptoms of diarrhoea, vomiting, abdominal pain, fever, chills, and myalgia [Reference Adler and Zickl4] (reviewed in [Reference Goodgame2, Reference Thornton, Jennings-Conklin and McCormick5]). Norovirus is mainly spread by the oral–fecal route through the ingestion of contaminated food or water vehicles, or by oral contact with a contaminated object (fomite) in the environment, such as shared toilet facilities or elevator buttons [Reference Ho6, Reference Wu7]. Thus, in this paper we consider foodborne, waterborne, and environmental transmission outbreaks as three causal mechanisms, each resulting from contamination of a common source (reviewed in [Reference Leon, Moe, Potter and Potter8]). Although person-to-person transmission is important (reviewed in [Reference Mattison9]) [Reference Blanton10], it will not be considered in the present analysis as outbreak characteristics for person-to-person outbreaks have been thoroughly addressed in the existing literature, including Matthews et al.'s recent systematic review that included analysis of person-to-person norovirus outbreaks [Reference Matthews11].
The attack rate, genogroup and strain distribution can provide important information for outbreak investigations [Reference Friesema12–Reference Xerry14]. Attack rate, defined as the number of cases per persons exposed, may be higher for transmission routes or vehicles that encourage more widespread exposure to norovirus or more efficient ingestion of viral particles (reviewed in [Reference Mattison9]) [Reference Kaplan15]. Genogroup and strain distribution may vary according to the characteristics of noroviruses that promote virus survival or propagation in a given media.
Noroviruses are a member of the Caliciviridae family, and are categorized into five genogroups GI–GV, of which three cause disease in humans: GI, GII, and GIV. Within genogroups, they are further categorized into clusters, and within clusters, the individual norovirus assigned to an outbreak is referred to as a strain [Reference Zheng16]. GII.4 cluster strains are the most common in outbreaks (reviewed in [Reference Mattison9]) [Reference Bull17], and will be referred to throughout as GII.4 strains to minimize redundancy. Both genogroup and GII.4 strain distribution may be associated with different outbreak transmission routes. For example, strains of the GII genogroup are more often associated with foodborne outbreaks [Reference Matthews11, Reference Lysen18], while GI strains are more often associated with waterborne outbreaks [Reference Matthews11, Reference Maunula, Miettinen and Von Bonsdorff19, Reference Riera-Montes20]; this is perhaps due to the stability of GI strains in water [Reference Lysen18, Reference Maunula, Miettinen and Von Bonsdorff19]. The presence of both GI and GII strains in a infected person's faecal or vomit samples may indicate food or water contamination by sewage, as sewage contains noroviruses circulating in the population and is likely to result in outbreaks with multiple strains [Reference ter Waarbeek21, Reference Gallimore22]. As identification of attack rate, genogroup distribution, or GII.4 strain distribution during an outbreak can implicate one transmission route or vehicle over others, a better understanding of the relationships between these outcomes with transmission routes and vehicles may facilitate outbreak investigations.
Other outbreak characteristics may confound the relationships between exposure routes and outcomes (attack rate, genogroup distribution, strain distribution). For example, previous studies have observed that attack rate, genogroup distribution, and GII.4 strain distribution of norovirus outbreaks have been associated with the setting of the outbreak (e.g. food service, leisure, school/daycare, healthcare) (reviewed in [Reference Thornton, Jennings-Conklin and McCormick5]) [Reference Matthews11, Reference Harris, Lopman and O'Brien23–Reference Bon26]. Genogroup distribution and GII.4 strain distribution have also been associated with season. Spring and summer outbreaks tend to have greater genetic diversity than winter outbreaks [Reference Matthews11, Reference Iritani27, Reference Verhoef28]. Outbreak characteristics also varied by hemisphere (reviewed in [Reference Thornton, Jennings-Conklin and McCormick5]) [Reference Marshall, Dimitriadis and Wright29, Reference Marshall30]. Due to the interrelatedness of outbreak characteristics, it is not clear which associations exist independently of other outbreak characteristics, and which associations are driven by the role of other outbreak characteristics. Therefore, in order to effectively characterize the relationships between outbreak outcomes and particular transmission routes or vehicles, it is important to control for other outbreak characteristics (e.g. setting, season, hemisphere). In one study, multivariate methods were employed to distinguish between outbreaks associated with food contaminated early in the processing chain, as opposed to those associated with a food-handler or person-to-person transmission, using strain profiles [Reference Verhoef31]. In another study, multivariate methods distinguished between foodborne and person-to-person outbreaks using the presence of GII.4 strain, number of cases, and setting [Reference Verhoef32]. Recently, our group employed multivariate techniques to describe the relationships for transmission and setting outcomes with attack rates and genogroup distribution for published norovirus outbreaks since 1992, but did not assess GII.4 strain distribution or commonly implicated vehicles [Reference Matthews11]. At present, the norovirus outbreak literature lacks a comprehensive analysis to assess whether certain measurable characteristics of an outbreak, such as attack rate, genogroup distribution, and GII.4 strain distribution are associated with foodborne, waterborne, or environmental transmission routes, as well as commonly implicated food or water vehicles of an outbreak.
We conducted such an analysis using a large collection of worldwide-published data through both bivariate and multivariate analysis.
METHODS
Outbreak data
Norovirus outbreak data were collected from peer-reviewed articles published between December 1993 and May 2011. Data abstraction methods are discussed in depth in Matthews et al. [Reference Matthews11]. Of 902 outbreaks confirmed by reverse transcription–polymerase chain reaction (RT–PCR), 435 contained information about norovirus transmissions for vehicles of interest. Categorical variables were constructed for primary transmission route (foodborne, waterborne, environmental), food vehicle categories (produce, shellfish, ready-to-eat) and water vehicle categories (tap and municipal water, ground water, surface water, recreational water) using information reported in publications. In outbreaks where multiple items were implicated, the item identified as most likely associated with the outbreak was used for the vehicle category (e.g. produce, shellfish, ready-to-eat for foods). For example, a specific vehicle was implicated if the authors explicitly mentioned that there was stronger circumstantial evidence in favour of that vehicle, or if epidemiological evidence (e.g. a higher significant odds ratio) was presented in favour of that vehicle. In our analyses, this implicated vehicle was then categorized into a vehicle category as described above. The outcome variables of interest were attack rate, genogroup distribution, and GII.4 strain distribution. Attack rate was defined as the number of cases out of all persons at risk for each outbreak. Genogroup was categorized for each outbreak according to the final classification reported by authors as the presence of GII strains only, GI strains only, or both GII and GI strains. GII.4 strain was categorized for each outbreak using published reports as either the presence of any GII.4 strain or the presence of only non-GII.4 strains.
Data analysis
Analyses were performed using statistical snalysis software v. 9.3 (SAS Institute, USA). The relationships were assessed between outbreak characteristic predictors (setting, season, hemisphere) and attack rate (via ANOVA tests followed by Tukey's post-hoc tests), genogroup, and strain (via Fisher's exact tests followed by multiple comparisons tests for proportions using the SAS compprop macro [Reference Elliott and Reisch33]). Multivariate analyses were performed using linear regression for attack rate, polytomous regression for the nominal, three-level variable for genogroup (GII only, GI only, and both GII and GI), and logistic regression for GII.4 strain (GII.4 and non-GII.4 strains). An interaction term for season and setting to assess effect modification was not possible due to paucity of data. Data were analysed to ensure that model assumptions were met (reviewed in [Reference Kleinbaum34, Reference Kleinbaum and Klein35]). Backward elimination was performed with partial F tests for linear models and likelihood ratio tests for polytomous and logistic models to determine which variables did not significantly improve prediction models and did not confound the relationship between the predictors of interest and outbreak outcome variables. Transmission route, food vehicles, and water vehicles were not eligible for backward elimination because these were the main exposures. In the instance of semi-complete separation of the predictor and the outcome, logistic regression modelling was supplemented with the Firth option to obtain estimates [Reference Heinze and Schemper36]. P values of <0·05 were considered statistically significant.
RESULTS
Bivariate analysis
A totalof 435 outbreaks with transmission route or vehicle information were included in the analysis. There was a significant association between attack rate and transmission route (Table 1). Additionally, significantly higher attack rates were observed for shellfish outbreaks compared to ready-to-eat outbreaks, for surface water outbreaks compared to tap water outbreaks, and for food-service outbreaks compared to either leisure setting outbreaks or healthcare setting outbreaks.
Table 1. Bivariate relationships between outbreak characteristics and outcomes of attack rate, genogroup, and GII.4 strain presence for published norovirus outbreaks
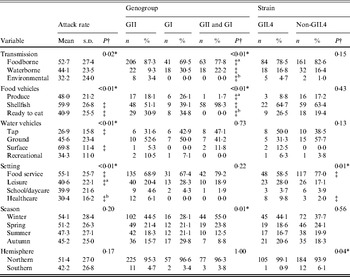
† ANOVA tests for normally distributed continuous variables and Fisher's exact tests for categorical variable assessment.
‡ Significantly different means and frequencies indicated.
a,b Superscript letters indicate values different from ‡ but not from each other.
* Significant at α = 0·05.
There was a significant association between genogroup and transmission, with a greater ratio of GII to GI outbreaks and a greater ratio of GII to both GI and GII outbreaks for foodborne and environmental outbreaks than for waterborne outbreaks. A significant association between genogroup and food vehicles was also present, with a greater ratio of GII only to both GI and GII outbreaks for produce and ready-to-eat foods than for shellfish outbreaks. There was also a significant association between genogroup and season.
There was a significant association between GII.4 outbreak strain and setting, with a greater ratio of GII.4 to non-GII.4 strain outbreaks in healthcare than in food-service setting outbreaks. Additionally, a significant association was present for hemisphere and GII.4 strain, with a greater ratio of GII.4 to non-GII.4 strain outbreaks in the Northern hemisphere than in the Southern hemisphere. In conclusion, we observed significant associations for attack rate with transmission, vehicles, and setting, for genogroup with transmission, food vehicle, and season, and GII.4 strain with setting and hemisphere.
Multivariate analysis
Transmission
Outbreaks (n = 432) with foodborne (n = 352), waterborne (n = 69), or environmental (n = 11) transmission were eligible for inclusion in models of attack rate, genogroup, and GII.4 strain (Table 2). Transmission route was not associated with attack rate or GII.4 strain, but was associated with genogroup distribution. Specifically, waterborne outbreaks, compared to foodborne outbreaks, were more likely to be associated with GI strains than GII strains, and both GI and GII strains than GII strains only. Season was associated with attack rate and genogroup distribution. A significantly lower attack rate was found for autumn outbreaks compared to winter outbreaks. GI strains rather than GII strains were more likely to be associated with spring and autumn outbreaks, compared to winter outbreaks. Setting was associated with attack rate, genogroup, and GII.4 strain. Lower attack rates were observed with leisure and healthcare than food-service setting outbreaks. GII strains only compared to both GI and GII strains were more likely to be associated with leisure than with food-service settings. GII.4 strains were more likely to be associated with leisure and healthcare than with foodservice setting outbreaks.
Table 2. Multivariate relationships between transmission and outcomes of attack rate, genogroup, and GII.4 strain presence for published norovirus outbreaks
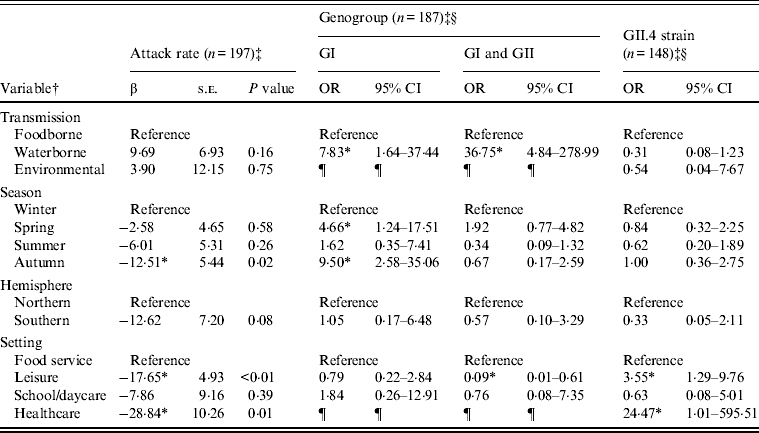
OR, Odds ratio; CI, confidence interval.
† Italic covariates are eliminated by backward elimination (α = 0·05) without confounding covariate estimates.
‡ Attack rate estimates adjusted for genogroup. Genogroup and strain estimates adjusted for attack rate.
§ Reference category for genogroup is GII. Reference category for strain is non-GII.4.
¶ Categories not included due to sparse data.
* Significant β or OR estimate.
Food vehicles
Outbreaks (n = 206) with produce (n = 28), shellfish (n = 133), or ready-to-eat (n = 45) food vehicles were eligible for inclusion in models of attack rate and genogroup (Table 3). Outbreak characteristics were not significantly associated with GII.4 strain (n = 86) (data not shown). Food vehicle was not associated with attack rate, but was associated with genogroup distribution. Specifically, shellfish outbreaks, compared to produce outbreaks, were more likely to be associated with both GI and GII strains rather than GII strains only, and GII strains only compared to GI strains. Hemisphere was associated with both attack rate and with genogroup. Southern hemisphere outbreaks had fewer cases per persons at risk than Northern hemisphere outbreaks. Southern, compared to Northern, hemisphere outbreaks were more likely to be due to GII than multiple strains.
Table 3. Multivariate relationships between food vehicles and outcomes of attack rate and genogroup for published norovirus outbreaks
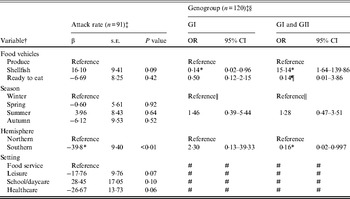
OR, Odds ratio; CI, confidence interval.
† Italic covariates are eliminated by backward elimination (α = 0·05) without confounding covariate estimates.
‡ Attack rate estimates adjusted for genogroup and vice versa.
§ Reference category for genogroup is GII.
¶ Estimates obtained using logistic model and Firth correction.
|| Spring, summer, and autumn are collapsed to obtain valid estimates.
# Variables not included due to sparse data.
* Significant β or OR estimate.
Water vehicles
Outbreaks (n = 60) with tap (n = 24), ground (n = 26), surface (n = 6), or recreational (n = 4) water vehicles were eligible for inclusion in a model of strain (data not shown). Outbreak characteristics were not significantly associated with attack rate (n = 31) or genogroup (n = 27) (data not shown). Water vehicle was not associated with GII.4 strain.
DISCUSSION
The goal of this study was to assess the association between outbreak transmission routes and vehicles with attack rates, genogroup distribution, and GII.4 strain distribution. We observed that genogroup distribution was significantly associated with transmission and food vehicles upon controlling for other outbreak characteristics. In contrast, both attack rate and GII.4 strain distribution were not associated with transmission, food vehicles, or water vehicles. We also observed other significant associations between outbreak characteristics (e.g. setting, season, hemisphere) and outcomes (attack rates, genogroup distribution, GII.4 strain distribution).
Our finding that attack rate was not associated with transmission, food vehicles, or water vehicles contradicts the findings in the literature that suggest these associations exist (reviewed in [Reference Mattison9]). Because we did observe significant bivariate associations for these relationships, this discrepancy may be due to the fact that variables such as setting confound the relationships between attack rate and transmission or food vehicles; it was only upon adjusting for these covariates that the significant association disappeared. This is in agreement with our group's previous analysis [Reference Matthews11]. For example, this confounding can be understood by observing that our data indicate that setting has a significant bivariate association with both attack rate (Table 1) and transmission (data not shown). Food-service settings have a higher attack rate than leisure settings, and food-service settings are more frequently reported for foodborne outbreaks while leisure settings are more frequently reported for waterborne outbreaks. It follows that an increased attack rate for food-service settings and increased proportion of foodborne outbreaks in food-service settings relative to leisure settings would lead to an apparent association between attack rate and transmission. An additional hypothesis to explain this finding is that stratifying by several variables yielded insufficient power to detect differences. However, we did detect attack rate differences for season, setting, and hemisphere, which suggest that we did have the power to detect differences, and supports the former hypothesis.
Genogroup distribution was significantly associated with transmission routes and food vehicles, but not water vehicles. Our findings support the existing literature regarding transmission and food vehicles; however, these previous analyses did not control for potential confounders, thus our findings strengthen previous observations of bivariate associations. The different genogroup distributions are likely to represent the varying stability of strains in different media and different contamination methods. The increased likelihood of GI only strains and both GI and GII strains rather than GII only strains in waterborne compared to foodborne transmission may be due to an increased stability of GI strains in water vs. GII strains [Reference Lysen18, Reference Maunula, Miettinen and Von Bonsdorff19]. In contrast, the genogroup distribution for shellfish and produce vehicles reflect different contamination methods. There was an increased likelihood of both GI and GII strains vs. GII strains only, and an increased likelihood of GII strains only vs. GI strains only, for shellfish compared to produce. The observation of increased likelihood of both GI and GII strains vs. GII strains for shellfish compared to produce may be due to the increased likelihood of shellfish to become contaminated by sewage with several strains of norovirus than by a single ill individual that spreads only a single strain or a few norovirus strains [Reference ter Waarbeek21, Reference Gallimore37]. The increased likelihood of GII strains only vs. GI strains only in shellfish compared to produce may be due to the higher proportion of GII strains circulating between individuals (reviewed in [Reference Mattison9]) [Reference Bull17, Reference ter Waarbeek21, Reference Gallimore37].
GII.4 strain distribution was not associated with transmission or vehicle. It is interesting that, while genogroup distribution varied to some extent by transmission or vehicle, the presence or absence of GII.4 strains in particular did not. GII.4 strains have been widely implicated in person-to-person outbreaks [Reference Zheng38], but may not represent an important outcome for foodborne, waterborne, and environmental outbreaks. This hypothesis is supported by Zheng et al.'s observation that GII.4 strains tend to predominate in settings with person-to-person transmission, while non-GII.4 strains were associated with outbreaks in settings with foodborne and environmental transmission [Reference Zheng38].
In addition to transmission and vehicles, these data indicate that other outbreak characteristics have important relationships with outbreak outcomes. Setting and season each appear to be important predictors for outbreak outcomes. During backward elimination, setting never dropped out, and season remained in models where setting could not be included due to sparse data. Furthermore, setting and season are likely to be interrelated with one another. We observed a significant association between season and setting (data not shown), which is consistent with existing literature [Reference Lopman39]. There was a higher proportion of leisure setting outbreaks than food-service setting outbreaks in the summer months. Unfortunately, data were too sparse to evaluate a potential interaction between setting and season in our multivariate models. Attack rate also varied by hemisphere for food vehicles. Evidence exists that outbreaks may vary by hemisphere, as outbreaks occur more frequently in the cooler months in the Northern hemisphere, and more frequently in the warmer months in the Southern hemisphere (reviewed in [Reference Thornton, Jennings-Conklin and McCormick5]) [Reference Marshall30]. The observed role of hemisphere could also reflect differences in reporting between hemispheres, as more than 90% of reported outbreaks occur in the Northern hemisphere [Reference Matthews11]. The potential for differences by hemisphere further demonstrates the need to control for covariates when analysing norovirus outbreak trends.
Limitations and strengths
These data provide a comprehensive approach to characterizing published foodborne, waterborne, and environmental outbreaks. However, as these data are from published norovirus outbreak reports, they are subject to reporting bias and publication bias. Our data may underrepresent regions with limited surveillance capacity, preventing extrapolation to areas with less reporting or decreased genotyping capabilities, such as developing countries. Our data are also restricted to the information provided in publications. As a result, there may be variation between outbreaks with respect to investigation techniques (e.g. investigators may be predisposed to suspect a particular transmission or vehicle), molecular detection or classification methods (e.g. deviations in assay sensitivity or phylogenetic classification), or threshold of detection [e.g. if it is easier to detect food-service cases than leisure cases, attack rate differences for food-service and leisure settings (Table 2) could result]. Although oubreak strains reported by different authors may vary due to different molecular methods, outbreak strains within an outbreak are unlikely to vary because most outbreak samples are collected during the acute phase of illness when strains have undergone few virus replication cycles [Reference Xerry14].
Norovirus outbreaks are often complicated, and multiple transmission routes or vehicles may be involved. We did examine the role of multiple transmission routes for the outbreaks in our dataset by adding an extra ‘multiple transmissions’ category to the existing single transmission routes (i.e. foodborne, waterborne, environmental). We observed that outbreaks with multiple transmission routes (n = 47) were not significantly associated with attack rate, genogroup distribution, or GII.4 strain distribution (data not shown). Only six outbreaks had multiple vehicles that fell into different categories (e.g. produce, shellfish, ready-to-eat). Multiple food vehicle categories were not significantly associated with attack rate (data not shown), and could not be assessed for genogroup or GII.4 strain. It was not possible to assess the role of multiple vehicles for water vehicle-mediated outbreaks. Although we were largely unable to assess the role of multiple vehicle category outbreaks, the size of this dataset provided the ability to examine vehicle-specific data with multivariate techniques for single vehicle category outbreaks – another strength of this study (reviewed in [Reference Mattison9]) [Reference Zheng38]. Additionally, the presence of significant predictors in our multivariate models suggests sufficient sensitivity to capture large differences between groups, despite stratification across several variables.
Conclusions and Implications
These data were employed to better understand the underlying relationships between outbreak predictors (transmission route and vehicles) and outcomes (attack rate, genogroup distribution, GII.4 strain distribution) that may be confounded by other outbreak characteristics. Although attack rate has important clinical implications for outbreaks, our analysis suggests that public health practitioners should not apply attack rate in arguments for or against a particular transmission route or vehicle during an outbreak investigation. Attack rate was not associated with transmission, food vehicle, or water vehicle, and appeared to be driven instead by setting, season, hemisphere, and genogroup or strain. On the other hand, genogroup distribution may strengthen the case against a particular transmission type or food vehicle because it did vary by transmission and food vehicles. GII.4 norovirus strains predominate in norovirus outbreaks; however, these data indicate that their presence may not be an important characteristic of foodborne, waterborne, and environmental outbreaks, as GII.4 strain was not associated with transmission, food vehicle, or water vehicle. As a result, GII.4 strain distribution may not be valuable for developing transmission or vehicle-specific prevention strategies and interventions. As many variables can impact norovirus outbreak outcomes, these data highlight the importance of controlling for potential confounders when examining the relationships between outbreak characteristics and outcomes. The observed relationships suggest that, when available, genogroup can be used to predict the likelihood of various transmission routes or vehicles to facilitate ongoing outbreak interventions and regulatory action. These relationships can also be utilized when developing transmission and vehicle-specific strategies to prevent future norovirus outbreaks.
ACKNOWLEDGEMENTS
The authors thank Rebecca Miller, Jamie Felzer, Bradley Dawson, Alice Sun Lee, Jennifer Rocks, Julia Montes, Barbara Abu-Zeid, Mia White, and Stephen Ragan for assistance with data abstraction, entry, and validation. This work was partially supported by the U.S. Environmental Protection Agency (J.N.S.E., STAR grant RD83172701), the National Institute of Allergy and Infectious Diseases at the National Institutes of Health (J.S.L., grant 1K01AI087724-01), the National Institute of Food and Agriculture at the U.S. Department of Agriculture (J.S.L., grant 2010-85212-20608), and the Emory University Global Health Institute (J.S.L.).
DECLARATION OF INTEREST
None.